Version française abrégée
1 Introduction
Quatorze gisements et occurrences de type porphyre à Cu et Cu–Au sont connus dans les monts Métallifères (Ouest de la Roumanie), parmi lesquels le gisement de Bolcana, situé à une quinzaine de kilomètres au nord-est de la ville de Deva (Fig. 1A). Ce gisement est connu et étudié depuis le début des années 1970 [5,6,32–34].
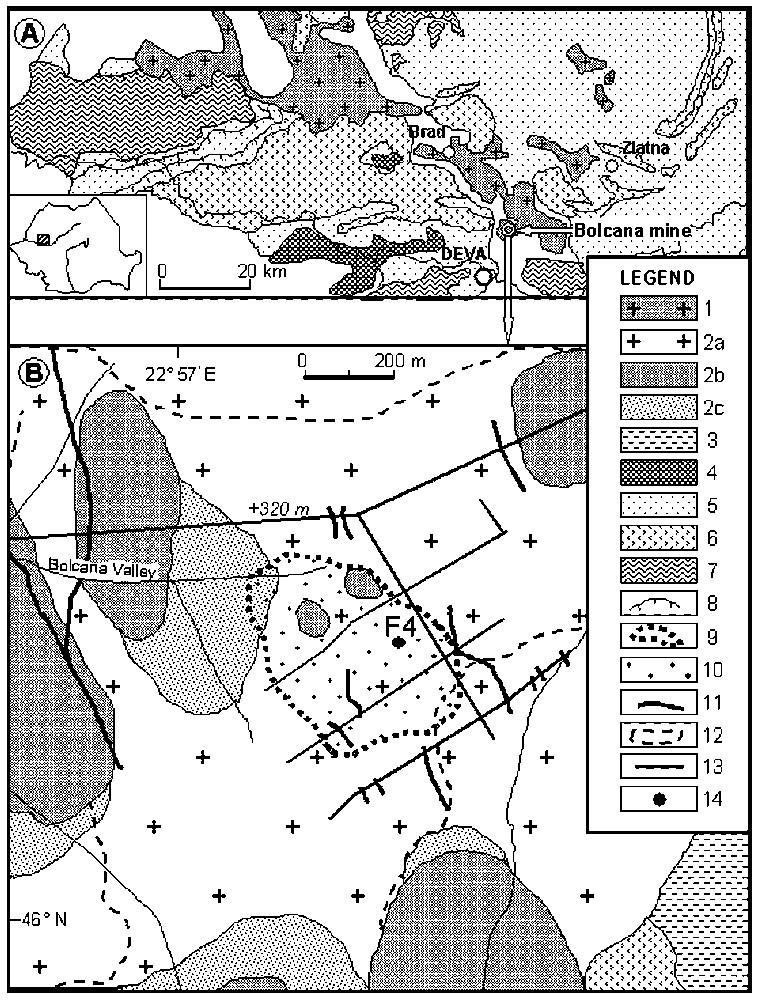
A. Simplified geological map of the Metaliferi Mountains showing the location of the studied area (after [10]). B. Geological map of the Bolcana mining area (after [33,34] with modifications). Location of F4 drill-hole is indicated. 1. Neogene magmatic rocks. 2. Upper Badenian–Sarmatian andesites-microdiorites (2a, lavas and pyroclastics; 2b, bodies; 2c, breccia); 3, Paleocene sedimentary rocks; 4, Upper Cretaceous–Paleocene magmatic rocks; 5, Mesozoic sedimentary rocks; 6, Mesozoic ophiolites; 7, Pre-alpine undifferentiated rocks; 8, overthrust; 9, limit of the Bolcana porphyritic intrusion at the level +320 m above see level (Grimm exploration level); 10, Porphyry copper mineralization; 11, epithermal vein; 12, limit of the area with altered rocks; 13, exploration galleries at the Grimm level; 14, drill-hole. Masquer
A. Simplified geological map of the Metaliferi Mountains showing the location of the studied area (after [10]). B. Geological map of the Bolcana mining area (after [33,34] with modifications). Location of F4 drill-hole is indicated. 1. Neogene magmatic ... Lire la suite
A. Carte géologique simplifiée des monts Métallifères, montrant la localisation de la zone étudiée (d'après [10]). B. Carte géologique de la zone minière de Bolcana (modifiée d'après [33,34]), avec localisation du forage F4. 1, Roches magmatiques néogènes ; 2, Andésites-microdiorites du Badénien–Sarmatien supérieur (2a, laves et pyroclastites ; 2b, corps ; 2c, brèches) ; 3, roches sédimentaires du Paléocène ; 4, roches magmatiques du Crétacé supérieur-Paléocène ; 5, roches sédimentaires mésozoı̈ques ; 6, ophiolites mésozoı̈ques ; 7, roches indifférenciées pré-alpines ; 8, chevauchement ; 9, limite de l'intrusion porphyrique de Bolcana au niveau +320 m au-dessus du niveau de la mer (niveau d'exploration Grimm) ; 10, minéralisation de type porphyre Cu ; 11, veines épithermales ; 12, limite de l'altération ; 13, galeries d'exploration au niveau Grimm ; 14, forage. Masquer
A. Carte géologique simplifiée des monts Métallifères, montrant la localisation de la zone étudiée (d'après [10]). B. Carte géologique de la zone minière de Bolcana (modifiée d'après [33,34]), avec localisation du forage F4. 1, Roches magmatiques néogènes ; ... Lire la suite
Le gisement de Bolcana appartient au district métallogénique néogène de Brad–Sacaramb (Fig. 1A). Dans la région de Bolcana, le magmatisme néogène débute par les andésites de Hondol-Faerag, d'âge Sarmatien (Fig. 1B). L'intrusion de Bolcana, de composition microdioritique, intrude ces andésites dans la partie centrale de la zone volcanique Dumbravita–Teascu–Faerag [9]. Ce corps sub-volcanique a été exploré jusqu'à une profondeur de 1300 m. Des brèches polygéniques à éléments d'ophiolite et d'andésite sont observées autour de celui-ci. Les produits volcaniques les plus récents sont représentés par des andésites quartzeuses dépourvues de minéralisation [5].
L'objectif de ce travail est de caractériser les paragenèses d'altération, leurs relations avec la minéralisation et d'utiliser leurs caractéristiques pour en estimer les températures de cristallisation.
2 Minéralisation
Bolcana est un gisement de type porphyre à Cu–Au, associé à des veines de type épithermal. La minéralisation de type porphyre est localisée dans le corps subvolcanique de Bolcana et est constituée de chalcopyrite, pyrite, bornite, magnétite, hématite, molybdénite, avec de l'or principalement en inclusions dans la chalcopyrite. L'extension maximale du corps minéralisé (750 m×440 m) se situe au niveau de l'horizon de Grimm, à l'altitude de 320 m (Fig. 1B). La minéralisation épithermale recoupe les roches altérées et minéralisées du type porphyre. Elle consiste en pyrite, sphalérite, galène, chalcopyrite, tétraédrite, bournonite, marcasite, or natif, arsénopyrite. Elle apparaı̂t sous la forme de veines polymétalliques à gangue de carbonates et de veines à quartz aurifère [32,33]. Le forage F4 (Figs. 1B et 2) permet d'observer une coupe de 1000 m dans les roches altérées et minéralisées. Les teneurs en Cu (0,11 pds % à 0,74 pds %) et Au (0,09 à 1,06 g t−1) augmentent avec la profondeur et sont maximales dans la zone d'altération potassique ± phylliteuse. Minéralisation à Cu, altération hydrothermale (potassique) et intrusion de Bolcana sont liées spatialement. Les analyses à l'échelle du gisement montrent que Au et Cu sont corrélés, mais qu'il n'y a pas de corrélation entre ces éléments et Mo.
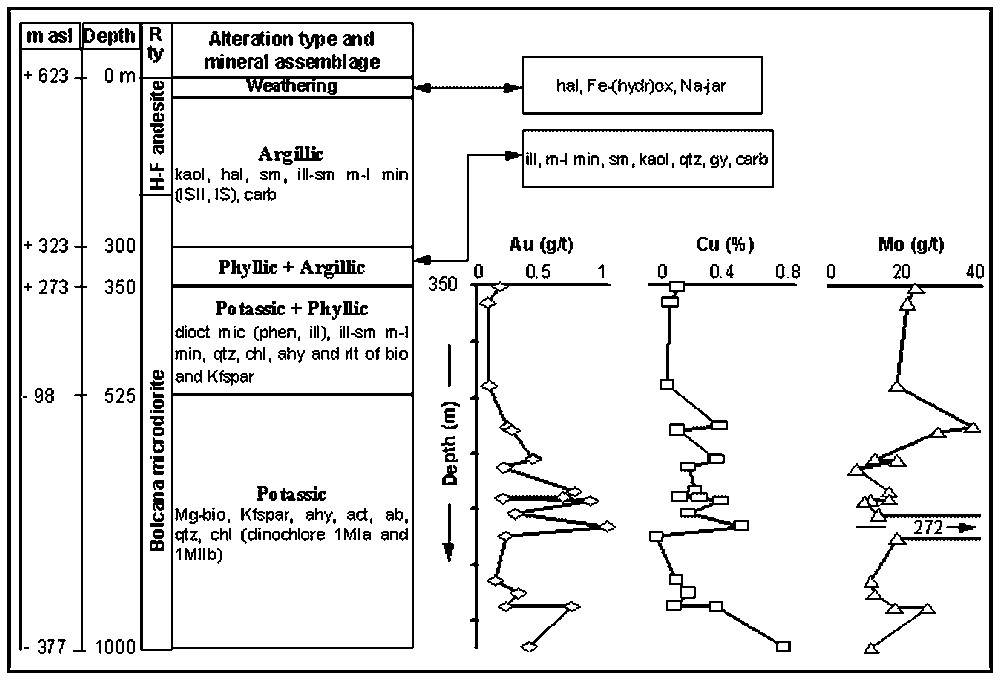
Synthetic column of F4 drill-hole, showing the characteristic mineral assemblages and the alteration types. The variation of Cu, Au and Mo contents in drillcore samples are also represented (content vs. depth diagram). ab, albite; act actinolite; ahy, anhydrite; bio, biotite; carb, carbonates; chl, chlorite; dioct mic, dioctahedral micas; Fe-(hydr)ox, Fe-(hydr)oxides; gp, gypsum; H–F, Hondol–Faerag; hal, halloysite; ill, illite; IS, illite/smectite; ISII, illite/smectite/illite/illite; kaol, kaolinite; Kfspar, K-feldspar; m asl, metres above sea level; Mg-bio, Mg-biotite; min, minerals; m-l, mixed-layer; Na-jar, Na-jarosite; phen, phengite; qtz, quartz; R ty, rock type; rtl, relicts; sm, smectite. Masquer
Synthetic column of F4 drill-hole, showing the characteristic mineral assemblages and the alteration types. The variation of Cu, Au and Mo contents in drillcore samples are also represented (content vs. depth diagram). ab, albite; act actinolite; ahy, ... Lire la suite
Log synthétique du forage F4, montrant les assemblages minéralogiques caractéristiques, les types d'altération, ainsi que les variations des teneurs en Cu, Au et Mo en fonction de la profondeur. ab, albite; act, actinote; ahy, anhydrite; bio, biotite; carb, carbonates; chl, chlorite; dioct mic, mica dioctaédrique; Fe-(hydr)ox, Fe-(hydr)oxydes; gp, gypse; H–F, Hondol–Faerag; hal, halloysite; ill, illite; IS, illite/smectite; ISII, illite/smectite/illite/illite; kaol, kaolinite ; Kfspar, feldspath K; m asl, altitude (en m) au-dessus du niveau de la mer ; Mg-bio, Mg-biotite ; min, minéraux ; m-l, minéraux interstratifiés ; Na-jar, Na-jarosite ; phen, phengite ; qtz, quartz ; Rty, type de roche ; rtl, reliques ; sm, smectite. Masquer
Log synthétique du forage F4, montrant les assemblages minéralogiques caractéristiques, les types d'altération, ainsi que les variations des teneurs en Cu, Au et Mo en fonction de la profondeur. ab, albite; act, actinote; ahy, anhydrite; bio, ... Lire la suite
3 Altérations hydrothermales
Quatre types d'altération ont été reconnus. L'altération potassique (Fig. 2) s'est développée dans la partie interne et profonde de l'intrusion et passe progressivement à l'altération phylliteuse, puis argileuse, ou brutalement à cette dernière. La biotite et le feldspath potassique en sont les principaux minéraux caractéristiques, avec chlorite, anhydrite, actinote, albite et séricite. L'altération phylliteuse s'est développée plus haut dans la structure et est caractérisée par illite, phengite, interstratifiés illite–smectite, quartz et pyrite, avec chlorite et anhydrite subordonnées. L'altération argileuse déborde largement autour de l'intrusion. Les minéraux caractéristiques sont kaolinite, smectite, interstratifiés et carbonates ( « altération argileuse intermédiaire »). L'altération propylitique est la plus externe et affecte principalement l'andésite de Hondol-Faerag. Elle est caractérisée par l'assemblage chlorite, épidote, actinote, avec séricite et carbonates subordonnés. En surface, une altération supergène se développe, conduisant à la formation de kaolinite, halloysite, Fe-(hydr)oxydes, Na-jarosite, etc.
Avec des valeurs de XFe comprises entre 0,27 et 0,31 et de AlVI entre 0,16 to 0,35 (formule calculée sur la base de 11 oxygènes), les biotites formées sont dans le champ des Mg-biotites [13]. Les chlorites sont toutes des clinochlores [1,12] (XFe entre 0,32 et 0,47 ; Si2.71Al1.29 à Si2.95Al1.05). Les minéraux phylliteux sont des phengite, illite, interstratifiés illite–smectite (IS and ISII) et K-smectite (Tableau 1), avec un continuum des phengites à la K-smectite (Fig. 3) [18].
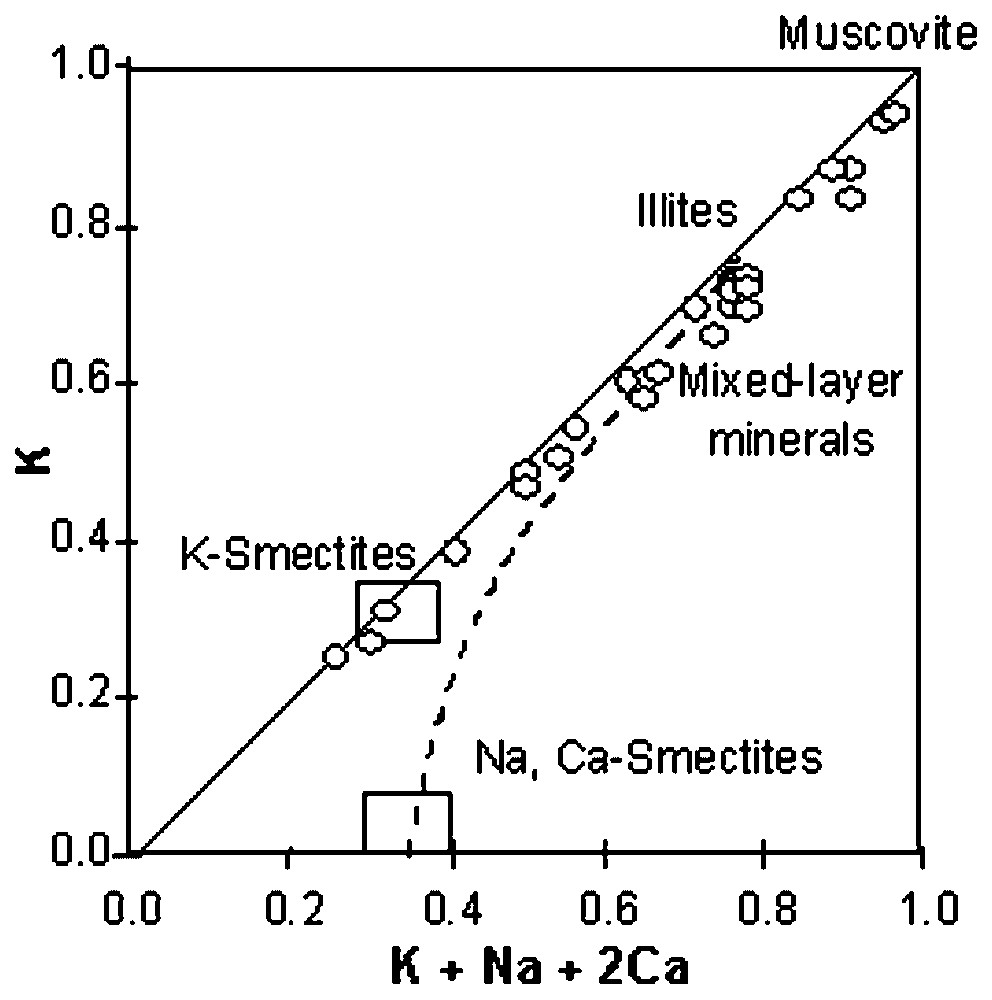
(K+Na+2 Ca) vs. K diagram (from [17]).
Diagramme (K+Na+2 Ca) vs. K [17].
4 Géothermomètres
Différents géothermomètres ont été utilisés (Tableau 2) afin d'approcher les températures de formation des minéraux d'altération. Ainsi, des températures de 350–450 °C ont été calculées pour la biotite à l'aide de la courbe de solubilité de Ti4+ en fonction de la température [22], conformément aux données de la littérature [2,11], 265–355 °C pour les chlorites [7,8], les températures les plus élevées correspondant à des chlorites en veine, 230–260 °C pour les illites [7].
Ranges of crystallisation temperatures of biotite, chlorite and illite from Bolcana ore deposit. bio, biotite; chl, chlorite; chl 1, chl in veinlet; chl 2 chl in groundmass; chl 3 chlorite on phenocrysts; ill illite; rpl replacement
Intervalle de température de formation des biotite, chlorite et illite néoformées du gisement de Bolcana. bio, biotite ; chl, chlorite ; chl 1, chl en veinule ; chl 2, chl dans la masse ; chl 3, chlorite sur phénocristaux ; ill, illite ; rpl, remplacement
Geothermometer | Crystallisation temperature (°C) | ||
Biotite [19] | 350–450 (rpl bio) | ||
Chlorite [7,8] | 345–355 (chl 1) | 285–320 (chl 2) | 265–272 (chl 3) |
Illite [7] | 230–260 (rpl ill) |
5 Discussion
Une diminution des températures de formation des biotites aux chlorites et aux illites, conforme aux données de la littérature [14,28,36] a été déterminée à l'aide des géothermomètres minéraux. Cette baisse de température est également indiquée par l'évolution minéralogique continue des phengites aux K-smectites (Fig. 3). En prenant en compte les zones d'altération observées et leurs relations spatio-temporelles, les températures estimées, ainsi que le domaine de stabilité des minéraux caractéristiques [14,19,21,35], l'altération hydrothermale développée à Bolcana est interprétée comme le résultat de la superposition de plusieurs types d'altération distincts. L'altération propylitique apparaı̂t comme synchrone de l'altération potassique, mais plus externe. L'altération phylliteuse est postérieure à l'altération potassique et a probablement formé un halo limité autour de l'intrusion de Bolcana. L'altération argileuse se superpose à l'ensemble des altérations.
Cette étude indique également l'association à Bolcana de deux systèmes, un système porphyre, tout à fait comparable à d'autres systèmes porphyres [23,25], et un système épithermal caractéristique des systèmes de type low sulphidation. Les stades précoces correspondent à une minéralisation de type porphyre à Cu–Au en relation, principalement, avec le développement de l'altération potassique. Dans les stades tardifs, des filons épithermaux à or–métaux de base recoupent les minéralisations et altérations porphyriques. Cette association spatiale entre système porphyrique à Cu (Au) et système épithermal de type low-sulphidation a été décrit en différents sites, comme en Papouasie-Nouvelle-Guinée (Ladolam) [27].
1 Introduction
The Metaliferi Mountains (western Romania) host 14 Neogene porphyry Cu and Cu–Au ore deposits and occurrences, including the Bolcana Cu–Au deposit. The Bolcana deposit belongs to the Brad-Sacaramb metallogenic district and is located approximately 15 km northeast of the town of Deva (Fig. 1A). The ore deposit has been identified and studied starting in the early 1970s and these studies resulted in important contributions [5,6,32–34]. However, there is no published data concerning the chemical composition of minerals associated with the Bolcana deposit.
This paper discusses both alteration and mineralization styles. The hydrothermal minerals are used to determine: (1) mineral assemblages and the alteration types; (2) relationships between alteration and mineralization; (3) alteration patterns. Mineral chemistry of some alteration minerals was used in order to estimate their crystallization temperatures.
2 Methods
Mineralogical studies were performed on samples collected from outcrops, exploration galleries and drill cores. Thin and polished thin sections were examined by optical microscopy. Mineral analyses were carried out using a Camebax–SX50 electron microprobe with a wavelength-dispersive spectrometer. Operating conditions: acceleration voltage was 15 kV, counting time 6 s, sample current of 12 nA. Analysed elements: Si, Al, Fe, Mg, Mn, K, Ca, Ti, and Cr (±Sr, Cl). X-ray diffraction analyses on whole rock samples and on argillic fractions were performed using a Siemens D5000 diffractometer. Major and trace elements were analysed by inductively coupled plasma (ICP) methods and Au by atomic absorption spectrophotometry (AAS). All these analyses were carried out at BRGM (Orléans, France) and at the Henri-Poincaré University (Nancy, France).
3 Geological setting
Neogene calc-alkaline magmatic activity is known to have started in western Romania at about 15 Ma and continued up to 7.4 Ma. After a gap of about 6 Ma, a more alkaline type activity is recorded [29,30].
The Bolcana deposit belongs to the Neogene Brad-Sacaramb metallogenic district (Fig. 1A). The basement of the area consists of Middle Jurassic–Lower Cretaceous ophiolitic rocks (basaltic andesites and basalts lava flows and pyroclastics) and Lower Cretaceous rhyolites (Baita rhyolites) overlain by Palaeocene sedimentary series (Almasul Mare Formation), composed of gravels, sands and silty clays [3,4], and sedimentary series of Middle Miocene age, consisting of clays, sands and gravels outcropping unconformably [24]. In the area of Bolcana, the oldest Neogene igneous rocks are represented by andesites that occur as lava flows, intrusions and pyroclastics (Fig. 1B). They are of Sarmatian age and are known as Hondol-Faerag andesites. A subvolcanic body (Bolcana intrusion) of microdioritic composition intrudes these andesites. The Bolcana intrusion is located in the central part of the Dumbravita–Teascu–Faerag Neogene volcanic zone [9] and it outcrops only in a small area. The Bolcana intrusion was explored down to 1300 m in depth. Intrusive polymictic breccias, with ophiolitic and Neogene andesitic elements, are observed around this subvolcanic body. The youngest volcanic products comprise quartz andesites, but they are devoid of mineralisation [5].
4 Mineralisation
Bolcana is a porphyry-type Cu–Au ore deposit with associated epithermal veins. The porphyry Cu–Au mineralisation is located in the Bolcana subvolcanic body and is represented by chalcopyrite, pyrite, bornite, magnetite, hematite, molybdenite, with subordinate gold (mainly as inclusions in chalcopyrite). Chalcopyrite is the dominant copper mineral. The maximum extension of the orebody (750/440 m) is observed at the Grimm horizon (level +320 m) (Fig. 1B). At the same horizon, the distribution of the epithermal veins is also presented. The veins cut the early altered and mineralised rocks. The epithermal mineralisation consists of pyrite, sphalerite, galena, chalcopyrite, tetrahedrite, bournonite, marcasite, native gold, arsenopyrite and pyrrhotite [32,33]. There are polymetallic veins with predominantly carbonate gangue and gold-bearing quartz veins; illite and mixed-layer minerals also form in and envelop these veins.
The F4 drill-hole (Fig. 1B), carried out by the MINEXFOR Company, provides 1000 m long section in altered and mineralisation-rich rocks. Within the mineralised section (Fig. 2), the Cu content ranges from 0.11 to 0.74 wt%, and the gold content varies from 0.09 to 1.06 g t−1. The Mo content varies from 7 to 38 g t−1; a higher Mo content (272 g t−1) was found in a sample with molybdenite vein located at the depth of 781 m. Whole-rock analyses indicate that Cu is positively correlated with Au, whereas there is no correlation between these elements and Mo. The Cu and Au concentrations increase with depth and the high-grade ore is located within the Bolcana intrusion affected by potassic ± phyllic alteration (Fig. 2). Many gold-bearing porphyry copper deposits have a close correlation between the gold grade and copper grade in the potassic alteration zone [20,31].
5 Hydrothermal alteration
5.1 Mineral assemblages and alteration types
The chemical compositions (average values) and structural formulae of some alteration minerals from Bolcana deposit are given in Table 1. In this deposit, the potassic alteration is dominated by secondary biotite that occurs mainly as replacement biotite (resulting from the alteration of primary hornblende and plagioclase) and disseminated biotite (fine aggregates in the groundmass). The presence of secondary biotite is restricted to the subvolcanic body. All the analysed biotites show an XFe ratio ranging between 0.27 and 0.31; the AlVI values vary from 0.16 to 0.35 (structural formula calculated on the basis of 11 oxygens). Mg/(Mg+Fe) and AlVI values indicate Mg-biotite [13]. Plagioclase retains its original forms but usually is more or less altered toward a variable assemblage including K-feldspar (e.g., Or74Ab23), albite, and phyllic minerals. Albite appears either as a rim around the more or less albitized primary plagioclase, or as veinlets or being disseminated in the altered rock. Chlorites, collected both from exploration galleries and drill-cores at different depths, are systematically clinochlore [1,12] and have a restricted compositional range. Their XFe molar ratio varies from 0.32 to 0.47 (the average: 0.39; Table 1) and the Si and Al occupancy in tetrahedral sites varies from Si2.71Al1.29 to Si2.95Al1.05.
Electron microprobe analyses (wt%) and structural formulae of some alteration minerals from Bolcana deposit. Structural formulae of minerals have been calculated on a basis of 11 oxygens, except for chlorite, whose formulae have been calculated on a basis of 14 oxygens. IS, illite-smectite mixed layer minerals; ISII, illite–smectite–illite–illite mixed layer minerals; n, number of analyses averaged; XFe=Fe/(Fe+Mg); ∗ΣFe determined as FeO
Composition chimique (moyenne en poids %, microsonde électronique) et formule structurale de quelques minéraux d'altération du gisement de Bolcana. Les formules structurales sont calculées sur la base de 11 oxygènes et de 14 oxygènes pour les chlorites. IS, minéraux interstratifiés illite–smectite ; ISII, minéraux interstratifiés illite–smectite–illite–illite; n, nombre d'analyses utilisées; XFe=Fe/(Fe+Mg); ∗ΣFe déterminé sous la forme de FeO
n | Biotite | Chlorite | Phengite | Illite | IS | ISII | Smectite |
4 | 15 | 3 | 7 | 6 | 2 | 4 | |
SiO2 | 37.25 | 27.94 | 49.00 | 48.32 | 48.74 | 48.04 | 51.92 |
Al2O3 | 16.51 | 20.29 | 31.11 | 30.30 | 31.17 | 28.79 | 35.13 |
Cr2O3 | 0.01 | 0.02 | 0.00 | 0.02 | 0.00 | 0.10 | 0.05 |
TiO2 | 2.23 | 0.07 | 0.05 | 0.05 | 0.04 | 0.01 | 0.04 |
FeO∗ | 11.91 | 19.64 | 2.47 | 3.27 | 3.29 | 4.40 | 1.08 |
MgO | 16.44 | 18.03 | 1.83 | 2.29 | 2.37 | 4.10 | 0.60 |
MnO | 0.21 | 0.34 | 0.08 | 0.07 | 0.03 | 0.15 | 0.05 |
K2O | 9.38 | 0.14 | 10.12 | 8.49 | 6.37 | 7.44 | 3.67 |
Na2O | 0.19 | 0.01 | 0.33 | 0.22 | 0.07 | 0.08 | 0.04 |
CaO | 0.01 | 0.12 | 0.08 | 0.19 | 0.17 | 0.16 | 0.11 |
Total | 94.15 | 86.61 | 95.07 | 93.20 | 92.25 | 93.27 | 92.69 |
SiIV | 2.77 | 2.88 | 3.27 | 3.27 | 3.28 | 3.25 | 3.35 |
AlIV | 1.23 | 1.12 | 0.73 | 0.73 | 0.72 | 0.75 | 0.65 |
AlVI | 0.21 | 2.09 | 1.71 | 1.69 | 1.75 | 1.55 | 2.02 |
Cr | 0.00 | 0.00 | 0.00 | 0.00 | 0.00 | 0.01 | 0.00 |
Ti | 0.12 | 0.01 | 0.00 | 0.00 | 0.00 | 0.00 | 0.00 |
Fe | 0.74 | 2.65 | 0.14 | 0.19 | 0.19 | 0.25 | 0.06 |
Mg | 1.82 | 4.16 | 0.18 | 0.23 | 0.24 | 0.43 | 0.06 |
Mn | 0.01 | 0.05 | 0.00 | 0.00 | 0.00 | 0.01 | 0.00 |
K | 0.91 | 0.03 | 0.88 | 0.75 | 0.56 | 0.66 | 0.31 |
Na | 0.03 | 0.00 | 0.04 | 0.03 | 0.01 | 0.01 | 0.01 |
Ca | 0.00 | 0.03 | 0.01 | 0.01 | 0.01 | 0.01 | 0.01 |
X Fe | 0.29 | 0.39 | 0.43 | 0.44 | 0.41 | 0.45 | 0.51 |
Furthermore, microprobe and XRD analyses indicate the presence of various phyllic minerals, like phengite, illite, illite-smectite mixed-layer minerals (IS and ISII) and K-smectite (Table 1). In Fig. 3, the K content in interlayer site and the interlayer charge values of these minerals were plotted in a discrimination diagram [18]. As it can be seen, both the terms of illite-smectite series and the smectites are dominantly potassic, with a continuous mineralogical variation from phengite to K-smectite.
On the basis of different parageneses, four alteration types were distinguished [26]: potassic, phyllic, argillic and propylitic.
The potassic alteration is developed in the deepest and innermost part of the intrusive body; it grades upward either to phyllic and then to argillic alteration or directly to the argillic alteration type. Drillings carried out within the core of the porphyry intrusion indicate that potassic alteration still occurs at the depth of 1000 m (e.g., F4-drill hole, Fig. 2). Mg-biotite and K-feldspar are the main minerals; chlorite (clinochlore 1MIa and 1MIb), anhydrite, actinolite, albite and sericite are also present.
The phyllic alteration is less developed and the characteristic minerals either crystallized in veinlets or replaced the pre-existing minerals in wall rocks. The phyllic assemblage consists of sericite, quartz and pyrite, with subordinate chlorite, anhydrite and relicts of biotite and K-feldspar. In the description of this alteration type, we use the term ‘sericite’ in the sense to include both illite and illite-smectite mixed-layer minerals [15,16].
The argillic alteration is developed in the upper part of the Bolcana ore-forming system and it affected both the porphyritic body and associated intrusion breccias, and the host rocks. The mineral assemblage corresponds to the intermediate argillic alteration type, characterized by the presence of kaolinite, smectite, mixed-layer minerals and carbonates. Fissures filled with minerals characteristic of the phyllic and argillic alterations crosscut even the deeper part of the porphyritic intrusion. In the argillic alteration zone, relicts of phyllic-altered rocks occur.
The propylitic alteration affected mainly the Hondol-Faerag andesites and occurs either like a broad halo around the other alteration types, or as relict islands in the argillic alteration zone. Propylitized rocks consist of chlorite, epidote, actinolite, and minor sericite and carbonates, which replaced plagioclase and mafic minerals.
A large development of sulphidation [32] and carbonatation (calcite) is a peculiarity of the Bolcana hydrothermal system. Anhydrite, often replaced by gypsum (especially where phyllic+argillic alteration overprints the potassic alteration), occurs both in the veinlets and groundmass.
At the surface, supergene alteration is developed. This type of alteration is characterized by the occurrence of kaolinite, halloysite, Fe-(hydr)oxides, Na-jarosite, etc.
5.2 Mineral geothermometry
Using the calculated structural formulae of some alteration minerals, their crystallisation temperatures were estimated applying different geothermometers (Table 2): the illite geothermometer using its K-interlayer content [7], the chlorite geothermometer [7,8] applied to different types of chlorites (formed by alteration of mafic phenocrysts and groundmass, and crystallized in veinlets), the solubility curve of Ti4+ as a function of temperature [22] for secondary biotite.
Geothermometry, based on biotite composition, indicates temperatures in the range 350–450 °C for the formation of a replacement biotite, similar to the crystallisation temperatures of biotite from other porphyry copper deposits (e.g., Santa Rita, New Mexico; Ray and Safford, Arizona; Panguna, Papua New Guinea) [2,11].
The composition of chlorite from Bolcana indicates temperatures of formation between 265 and 355 °C. The highest calculated formation temperatures (345–355 °C) correspond to chlorite in veinlets and the calculated temperatures decrease from chlorite in veinlet to chlorite formed in groundmass and to chlorite formed from phenocrysts.
Applying the illite geothermometer, a temperature range from 230 to 260 °C was obtained for the replacement illite, formed in groundmass.
6 Discussion
The continuous mineralogical variation from phengite to K-smectite, observed in Fig. 3, gives evidence of, and is related to the decrease of temperature and/or to the chemical evolution of the hydrothermal fluids in the system [17]. Concerning the estimated crystallisation temperatures of biotite, chlorite and illite (Table 2), a temperature decrease is observed from biotite to chlorite and illite. The thermometric data are consistent with the crystallization temperatures of these minerals [14,28,36]. Taking into consideration the values obtained and also the alteration zones and the stability domains of specific minerals [14,19,21,35], the alteration pattern at Bolcana is considered as the result of an overprinting of alteration processes. Propylitisation seems to be nearly simultaneous with, but peripheral to, the potassic alteration. Phyllic alteration is posterior to the potassic alteration and is supposed to have formed a reduced halo around the Bolcana intrusion. Argillic alteration overprints the other alteration types.
At Bolcana, both porphyry and epithermal systems are distinguished. The alteration assemblages associated to porphyry copper mineralisation are similar to those described in other porphyry copper systems [23,25]. The potassic alteration and porphyry copper mineralisation are spatially related and are thought to be formed almost at the same stage with the likelihood that the mineralisation process continued during later alteration processes. In the epithermal veins, mineralisation and alteration assemblages are characteristic of a low-sulphidation epithermal type mineralisation.
The paragenetic studies indicate an evolution of the system from an early period of porphyry Cu–Au mineralisation (mainly chalcopyrite, pyrite, magnetite, hematite, bornite, and molybdenite, with subordinate native gold) to a late period of low-sulphidation epithermal mineralisation (pyrite, galena, chalcopyrite, tetrahedrite, marcasite, bournonite, arsenopyrite, pyrrothite and native gold).
Therefore, the Bolcana deposit is characterized by a spatial association between a porphyry Cu (Au) and a low-sulphidation epithermal mineralization, as described in several places such as in Papua New Guinea (e.g., Ladolam) [27].
Acknowledgements
The MININVEST and the MINEXFOR Companies are thanked for allowing accesses to their mines. Field support by E. Orlandea and D. Filipescu is acknowledged. We would like to thank Prof. Dr. E. Marcoux and an anonymous reviewer for their useful comments.