1 Introduction
The Upper Palaeozoic massif of SW England has long been recognized as an integral part of the European Variscides and correlation with the Rhenohercynian Zone/South Portuguese Zone of mainland Europe is widely accepted [38,45,62]. SW England features prominently in Variscan tectonic models due to the presence of the Lizard ophiolite [11,52].
A 40-year programme of 1:10,000 scale geological re-mapping and collaborative research by the British Geological Survey (BGS) in SW England forms the basis for much of this article (Fig. 1). It has substantially refined understanding and models of passive margin evolution, Variscan convergence and post-Variscan sedimentation [18,19,29,35,37,57,59,60,69]. In addition, extensional reactivation of the Rhenohercynian suture zone and inverted passive margin has been interpreted to exert a significant control on Early Permian magmatism and sedimentary basin development [46,88–90].

Simplified geological map of SW England, modified after [58]. Inset shows location within European Variscides.
Fig. 1. Carte géologique simplifiée du Sud-Ouest de l’Angleterre, d’après [58] modifiée. Sa localisation dans la Chaîne varisque d’Europe est indiquée dans l’encadré.
The purpose of this contribution is to combine the above elements into an integrated overview of the Upper Palaeozoic tectonic evolution of SW England that demonstrates:
- • Early Devonian to Early Carboniferous development of the Rhenohercynian passive margin (northern lower plate);
- • Mid-Late Devonian accretion along the southern active margin (upper plate represented by Normannian Nappe, correlated with the Mid-German Crystalline High of the Saxothuringian Zone);
- • Carboniferous collision and progressive inversion of the passive margin;
- • Latest Carboniferous to Early Permian post-convergence extension, magmatism, mineralization and sedimentary basin development.
The wider implications of the SW England data for models of Variscan tectonics and the Rheic Ocean are then discussed.
2 Development of the passive margin
Rift and extensional basin models have been progressively refined since the 1970s, as more stratigraphical and structural detail has emerged from the BGS mapping programme [63,83,87]. The full definition of basins has recently been completed [58,60]. Evidence for the development of the passive margin is preserved within the Lizard Complex and Devonian-Carboniferous basins to the north (Fig. 1).
2.1 Pre-rift basement
Pre-rift basement is exposed within the Lizard Complex (Fig. 2), where it occurs structurally below mantle peridotites [49,72]. The Man of War Gneiss comprises tonalitic gneiss with continental magmatic arc affinities and a Late Cambrian magmatic U-Pb zircon age of 499 +8/-3 (2σ) Ma [81]. It has a faulted upper boundary with amphibolite facies metasedimentary and metabasic rocks of the Old Lizard Head Formation [49], that host granodiorite sheets with similar magmatic ages and geochemistry as the Man of War Gneiss [17,69]. Further north, rare xenoliths of foliated granitoid occur in the parautochthonous Late Devonian syn-rift basalts of the Gramscatho Basin [39,40].

Simplified geological map of the Lizard Complex, after [19,37,72].
Fig. 2. Carte géologique simplifiée du Complexe du Lizard, d’après [19,37,72].
2.2 Lizard Complex
Outcrop, borehole and geophysical data indicate that the Lizard Complex (Fig. 2) comprises c.1–1.5 km of fault-bounded sheets underlain by Devonian metasedimentary rocks [6,11,27,49,76]. The mantle peridotites are predominantly spinel lherzolites that initially equilibrated at 1119 °C and 15.7 kbar and then partially re-equilibrated to plagioclase peridotite (1070 °C, 11 kbar) [18]. There are transitional contacts with kilometer-scale higher strain zones, comprising mylonitic plagioclase peridotites (equilibrated at 1010 °C, 7.5 kbar) and mylonitic amphibole peridotites (equilibrated at 990 °C, 7.5 kbar), that commonly exhibit a NNW-SSE striking steeply dipping foliation and down-dip mineral lineation [18].
In the east, the spinel lherzolites are overlain by the c. 1 km thick Crousa Gabbro [37]. It is cut by three sets of NW-SE striking dolerite dykes, each with a slightly different MORB geochemistry [53,75]. The two earlier sets were rotated by NW-SE striking brittle-ductile shear zones, that thinned the crust by c. 40%, before emplacement of the final subvertical set that forms the lowermost part of a sheeted dyke complex [75]. These shear zones have locally controlled syntectonic emplacement of Fe-Ti oxide gabbros [48]. Variably deformed gabbro and dolerite sheets are also hosted by peridotite [3,41].
The mafic and ultramafic rocks of the Traboe Cumulate Complex [55] are interlayered with the mylonitic peridotites and record the same fabric [18,19]. Cambro-Ordovician zircon cores imply proximity to pre-rift basement [69]. Quartz-feldspar-amphibole veins developed during granulite facies partial melting have yielded a magmatic U-Pb zircon age of 397 ± 2 (2σ) Ma (Late Emsian) [16]. In the south and west, the mantle peridotites are underlain by the Landewednack Amphibolites; their protoliths are inferred to be basalts, dolerite dykes and minor gabbros that have a similar MORB geochemistry to the Crousa Gabbro [19,37]. SHRIMP U-Pb ages of c. 500 Ma from zircons in rare metasedimentary rocks are similar to those in pre-rift basement [17,69]. An early high temperature (550–700 °C, 2–6 kbar) prograde brown amphibole fabric, dated at 393–386 Ma by SHRIMP U-Pb metamorphic zircon also exhibits kinematic similarity with the mylonitic peridotites [19,69].
2.3 Devonian-Early Carboniferous rift basins
North of the Lizard Complex, Devonian-Carboniferous successions are preserved within six, east-west trending, sedimentary basins (Fig. 1). The oldest succession occurs in the Looe Basin (Fig. 3a) and comprises Lochkovian-Pragian non-marine (Dartmouth Group) and Pragian shallow marine (Meadfoot Group) sedimentary rocks and bimodal rift-related igneous rocks [60,65]. Significant northerly-derived sediment input occurred in the Late Emsian (Staddon Formation) and may have been coeval with a hiatus in subsidence; it resulted in the brief resumption of non-marine depositional environments. The development of later basins is indicated by the availability or blocking of sediment supply, including from inter-basinal highs. The progressive northwards initiation of one or more half graben or graben with distinct stratigraphies is recorded (Fig. 3): South Devon Basin (Late Emsian), Tavy Basin (Givetian) and Culm Basin (Late Famennian) [58,60]. A segmented marine shelf with limestone reefs on basement highs persisted throughout much of the Middle-Upper Devonian (Fig. 3b and c). To the south, the syn-rift infill of the Gramscatho Basin (Fig. 4) is largely occluded by subsequent Upper Devonian syn-convergence sedimentation. Seismic refraction studies imply pre-rift continental basement may occur within the Carrick Nappe [27]. A late syn- to post-rift succession is preserved at the base of the Veryan Nappe (Fig. 4), where Eifelian mudstones, radiolarian cherts, platform-derived limestone turbidites and basement-sourced sandstones overlie T-MORB pillow basalts [58,59]. The Start Complex (Fig. 1) comprises greenschist facies metasedimentary and metavolcanic rocks with N-MORB characteristics [36] that might be correlated with allochthonous parts of Gramscatho Basin.
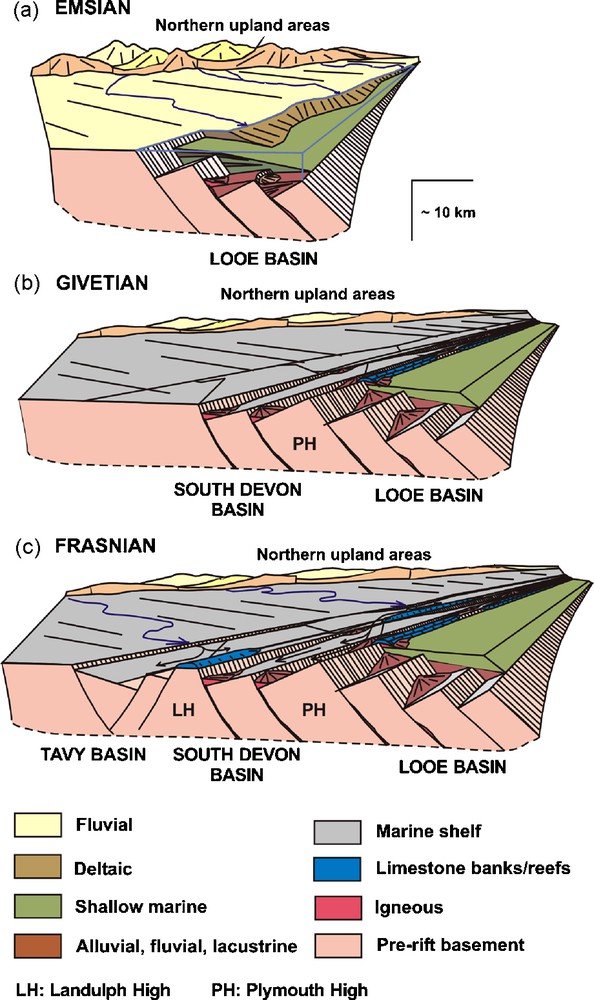
Progressive development of SW England passive margin basins during Devonian, after [60].
Fig. 3. Développement progressif des bassins de la marge passive du sud-ouest de l’Angleterre durant le Dévonien, d’après [60].
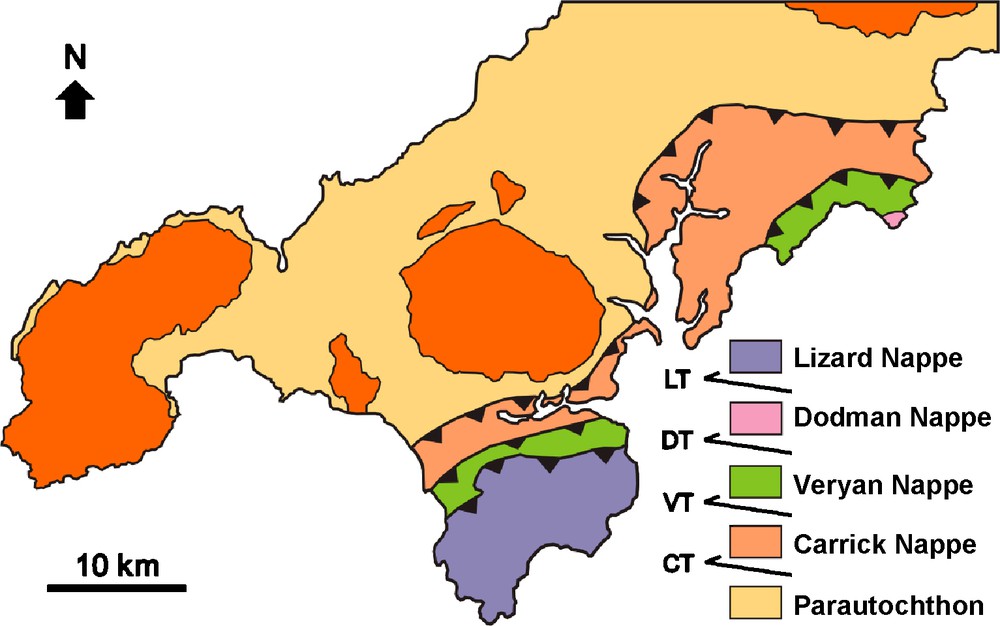
Simplified geological map of Gramscatho Basin, showing principal structural units, after [59].
Fig. 4. Carte géologique simplifiée du bassin de Gramscatho, montrant les principales unités tectoniques, d’après [59].
3 Convergence and passive margin inversion
Seismic reflection investigations to the South of the peninsula have identified prominent, semi-continuous, south-east dipping seismic reflectors within the crust that are generally interpreted as (reactivated) Variscan thrusts, some of which have an onshore expression [8,43,58]. The geometry and extent of these reflectors is consistent with the southern part of SW England forming a Variscan orogenic wedge [58].
3.1 Onset of convergence and accretion of the distal passive margin
The Gramscatho Basin succession is dominated by a southerly-sourced deep marine siliciclastic depositional system that prograded across the distal passive margin during its progressive frontal accretion to the upper plate in the Mid- to Upper Devonian; it is preserved in a parautochthonous northern region plus the Carrick, Veryan and Dodman nappes (Fig. 4) [44,57–59]. The onset of convergence is marked in the Veryan Nappe by the transition, from a Late Eifelian syn- to post-rift hemipelagic-dominated succession (Pendower Formation), to a deep marine sandstone-dominated succession (Carne Formation) and overlying olistostrome (Roseland Breccia Formation), up to 2 km thick, in the footwall of the Dodman and Lizard nappes [7,44].
The olistostrome clast assemblage includes sandstones, mudstones, limestone (Silurian, Lower Devonian and Eifelian), radiolarian chert (Eifelian), basalt, gabbro, and rare serpentinite, quartzites (Ordovician), slates, phyllites, schists, granites (variably foliated), rhyolites and amphibolites [5,35,57]. The Ordovician quartzites have a Llandeilo trilobite and brachiopod fauna that has been correlated with Armorican successions [78]. The upper, most proximal, parts of the olistostrome contain kilometer scale megaclasts of T-MORB pillow lavas and dolerite sheets [33,34]. There is also contemporaneous bimodal magmatism [57].
The final stages of accretion are marked by Famennian-? Tournaisian olistostromes at the top of the parautochthonous succession that record uplift and erosion of the Carrick Nappe [44,58]. They were immediately preceded, in the parautochthon, by the Late Frasnian-Famennian eruption of substantial volumes of basalt lavas and emplacement of dolerite/gabbro sills, all with enriched T-MORB geochemistry [34,36,59].
Accretion of the Gramscatho Group succession resulted in deformation and intermediate pressure facies series anchizonal-epizonal regional metamorphism [96]. D1 deformation is characterized by ENE-WSW slaty cleavage, tight to isoclinal curvilinear folds, and a NNW-SSE mineral lineation that are compatible with a top sense of shear to the NNW [58]. Metamorphic inclusion fluid studies in the Carrick Nappe indicate peak (syn-D1) P/T conditions of ∼300–330 °C and 2.9–3.5 kbar [88]. K-Ar whole rock data [26], recalculated with revised decay constants, and excluding contact metamorphic overprinted samples, yield Early Tournaisian to Famennnian mean ages of 358 ± 8 (2σ) (Carrick Nappe), 361 ± 8 (2σ) (Veryan Nappe) and 371 ± 8 (2σ) Dodman Nappe. A more recent precise Ar-Ar, whole rock age plateau for the Dodman Nappe is 385 ± 2 Ma (Earliest Frasnian) [15].
In the Lizard Complex, the boundary between mantle peridotites and the underlying Landewednack Amphibolites is formed by gently dipping amphibolite facies shear zones that have a top sense of shear to the northwest [19]. Deformed, variably commingled and mixed, mafic and felsic igneous rocks (Kennack Gneiss) intrude the boundary zone [11,82]. The mafic component has an E-MORB geochemistry and the felsic component has a continental crust source [69,82]. Estimates of magmatic age by U-Pb zircon methods vary from 376.4 ± 1.7 (2σ) Ma (Late Frasnian, by TIMS) [82] to 396 ± 20 Ma (Early Eifelian, by SHRIMP) [69]; see discussion [17]. Amphibolite facies emplacement-related deformation and metamorphism of the Landewednack Amphibolite, Kennack Gneiss, Man of War Gneiss and Old Lizard Head Formation are all dated between Latest Frasnian and Earliest Tournaisian [15,80–82].
The upper plate (Normannian Nappe), defined by offshore seismic mapping [44,45,58], crops out as garnetiferous granite gneiss at Eddystone Rocks (Fig. 1); it has a Frasnian K-Ar biotite cooling age, recalculated using revised decay constants, of 382 ± 17 Ma [66].
3.2 Collision and basin inversion
The orogenic wedge, comprising frontally accreted elements of the distal passive margin (Gramscatho Basin/Lizard Complex) and upper plate (Normannian Nappe) collided with the continental slope in the Earliest Carboniferous. South of the Culm Basin, K-Ar whole rock ages, reflecting syn-D1 anchizone-epizone grade regional metamorphism, range from 345–325 Ma (Late Tournaisian–Early Namurian) [26,96] and are consistent with 40Ar-39Ar whole-rock laser probe dates for Looe Basin anchizonal metamorphism of 331–329 ± 2 Ma [15]. Shortening was accommodated by “thick-skinned” inversion of basin fill and pre-rift basement [58,83,87] above a regional “décollement” at the base of the middle crust [12]. Rift basin geometry exerted a dominant control on the style of deformation (Fig. 5c). S-dipping half graben bounding faults were reactivated as thrusts and often developed km-scale hangingwall antiforms; successions from highs were locally thrust northwards, via footwall shortcuts, over adjacent basinal sequences [60]. Backthrusting, controlled by north-dipping faults defining the southern margin of full graben. Tavy Basin, Culm Basin (Fig. 5b), resulted in syn-D1 facing confrontations with regional persistence (Fig. 5d) [57,60]. Mesoscopic D1 structures include thrusts, an ubiquitous cleavage, and close to isoclinal folds, that all typically trend E-W; extension lineations are generally orientated NNW-SSE. Dextral displacement on NW-SE strike-slip faults developed late in D1 [60].
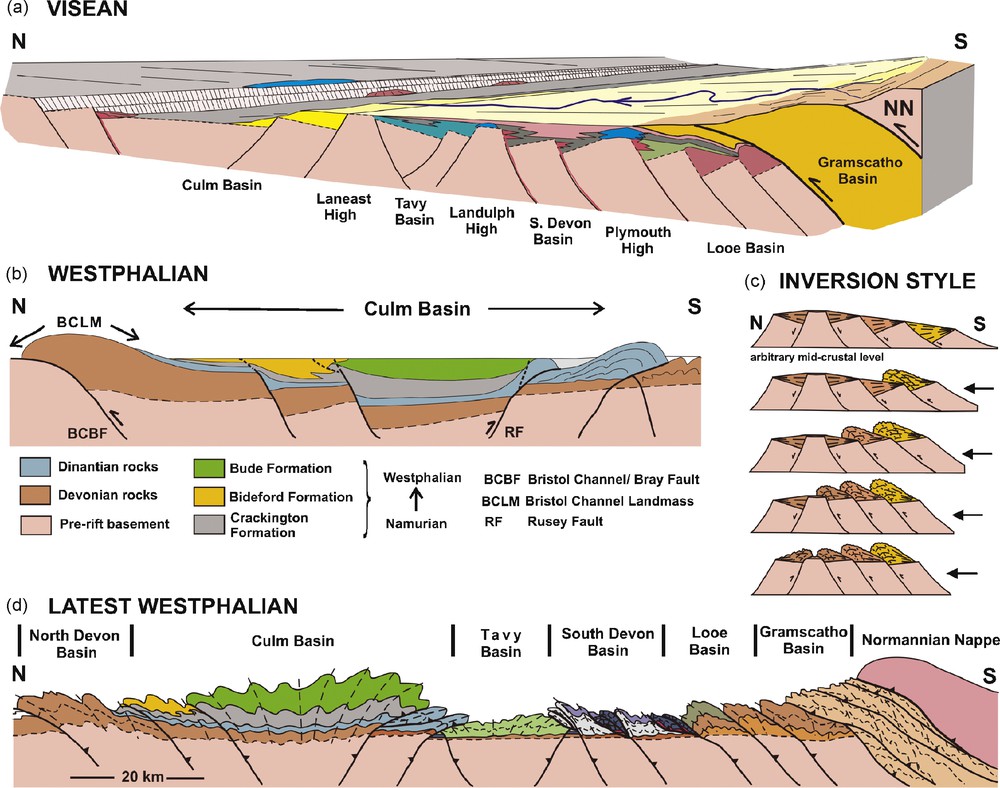
Schematic north-south cross-sections, showing progressive inversion of the SW England passive margin; a: early stages (Visean); b: detail of Culm Basin (Westphalian); c: cartoon depicting rift basin geometry control on inversion style; d: End-Westphalian (convergence ceased). After [57,60].
Fig. 5. Coupes nord-sud schématiques, montrant l’inversion progressive de la marge passive du Sud-Ouest de l’Angleterre ; a : stades précoces (Viséen) ; b : détail du bassin du Culm (Westphalien) ; c : schéma montrant l’influence de la géométrie des bassins sur le style de l’inversion tectonique ; d : Westphalien terminal (fin de la convergence). D’après [57,60].
Syn-rift sedimentation and basaltic magmatism persisted along the southern margin of the Culm Basin until the Late Visean. These successions were expelled southwards during Early-Mid Namurian D1 inversion and include Tournaisian turbidite sandstones (St Mellion Formation) that have southerly derived palaeocurrents and include clasts of low-grade metamorphic rocks and K-feldspar that were probably derived from the Gramscatho-Normannian orogenic wedge (Fig. 5a) [57]. However, the main part of the Culm Basin fill, as currently observed, comprises Namurian and Westphalian sandstones and mudstones (Crackington, Bude and Bideford formations) that were sourced from the north and east and deposited synchronously with inversion [57].
A second episode of deformation (D2) is variably developed throughout SW England; for post-Namurian successions of the Culm Basin and the North Devon Basin, it was the first episode of post-lithification deformation. D2 deformation is strongly partitioned, typically into zones of reactivated or out-of-sequence thrusts, and is characterized by north-east or ENE trending folds and a gently to steeply inclined crenulation cleavage [60]. The kinematics of D2 are compatible with a top sense of shear to the NNW or NW; D2 structures are commonly coaxial with D1 structures, but there can be an anticlockwise divergence of up to 30° [57]. Transpressive fabrics are developed where there is obliquity with earlier basinal and deformation structures. K-Ar whole rock ages for the North Devon Basin group around 305 Ma [26,96].
4 Extensional reactivation
The youngest deformed rocks of the Culm Basin are Westphalian C in age; Variscan convergence across SW England ended in the Late Carboniferous c. 305–300 Ma [29]. It was replaced by a Latest Carboniferous-Early Permian NNW-SSE extensional regime that brought about substantial thinning of the crust, largely through reactivation of earlier structures, and was associated with sedimentary basin development and voluminous magmatism [46,88].
4.1 Structures developed during extension
In the orogenic wedge (Gramscatho Group/Lizard Complex), postconvergence deformation (D3) is represented by zones of distributed shear, detachments and later high-angle faults [1,2,89]. Zones of distributed shear are ubiquitous throughout the parautochthonous footwall of the Carrick Thrust and are defined by ENE striking domains of F3 folds and a subhorizontal or NNW dipping cleavage (S3) that locally transposes earlier fabrics. Fold vergence is predominantly to the SSE, although can undergo reversal in high-strain zones [1,2]. Detachments occur in proximity to the Carrick and Dodman thrusts and are ENE striking, low-angle faults, generally bedding and cleavage parallel, that are associated with complex arrangements of secondary extensional faults and SSE-verging folds; the kinematics of all structures are compatible with a top sense of shear to the SSE [1,2].
A north-dipping S3 cleavage is also sporadically developed throughout the Looe and South Devon basins [57] and is axial planar to a large-scale south-verging monoform that crops out across the peninsula towards the southern margin of the Looe Basin [20,21]. Its steeply inclined limb (Fig. 1), up to 5 km across and characterised by high strain secondary transposition fabrics, has been termed the Start-Perranporth Zone [47]. The association of steeply dipping foliations, containing rare east-west trending D1 mineral lineations and highly curvilinear D2 fold hinges, has been interpreted in terms of persistent convergence-related dextral transpression across a steeply inclined basement fault zone [47]. Nevertheless, field relations indicate that steeply dipping fabrics are primarily a result of the back rotation of gently S dipping D1 and high strain D2 fabrics during the D3 development of the monoform [20,21]. Whilst elements of D1 and D2 dextral transpression may be present, some “oblique” D2 structures are a consequence of non-coaxial reorientation during D3. Rather than marking a major transpressional zone or convergence-related backfold, the Start-Perranporth Zone formed by top-to-the south extensional reactivation of a major out of sequence high strain D2 thrust zone, probably aided by forced folding above an extensionally reactivated basement fault [58]. Recalculated K-Ar whole-rock ages within this zone range from 315–292 Ma [26,96].
Further north, D2 chevron folds that relate to the main episode of Culm Basin inversion are modified by southerly directed D3 simple shear [61]. The St Mellion “Outlier” (Fig. 1) comprises Tournaisian rocks that were originally deposited on the southern margin of the Culm Basin; they were translated up to 22 km southwards on subhorizontal faults during post-D2 gravitational collapse of the inverted Culm Basin [60].
There is a temporal progression, across the whole region, from gently dipping extensional faults, folds and cleavage (plastic-brittle) to higher angle brittle extensional faults that cross-cut earlier structures and are compatible with continued NNW-SSE extension [1,2]. These exert a fundamental control on outcrop geometry and can result in the cut-out of thrust faults that had previously undergone extensional reactivation, e.g. Lizard Boundary Fault, Carrick Thrust [1,2,6,72].
4.2 Early Permian magmatism, mineralization and sedimentary basins
The earliest onshore evidence for the development of post-Variscan sedimentary basins is in the Crediton Trough (Fig. 1), a 45 km long half graben controlled by thrust fault reactivation that predates emplacement of the Dartmoor Granite [29]. Deformed Culm Basin rocks (Namurian-Westphalian), exhumed from 6–7 km [96], are unconformably overlain by? Stephanian-Sakmarian alluvial “red-bed” sedimentary rocks (Exeter Group) that predate, and are intercalated, with basalts and lamprophyres yielding 40Ar-39Ar biotite ages between 290.8 ± 0.8 Ma and 281.8 ± 0.8 Ma [29]. Offshore, to the South of the peninsula, the Western Approaches basins unconformably overlie the Variscan orogenic wedge [8,25,30,43]. The Plymouth Bay Basin contains up to 10 km of Permo-Triassic sedimentary rocks and is underlain by prominent zone of south-east dipping seismic reflections that passes through the whole crust and into the mantle [8]. The lower two megasequences are ENE striking, overlain by bright reflectors interpreted as Latest Carboniferous-Early Permian lavas similar to those onshore, and are considered to have formed by extensional reactivation of thrusts defining the orogenic wedge and upper plate [77].
The SW England Batholith extends at least 300 km, from Dartmoor to west of the Scilly Isles; granitoids also occur further south-west on the Goban Spur [24,30]. The batholith is hosted by the lower plate, in the immediate footwall of the orogenic wedge, and was emplaced during the Early Permian (∼295–270 Ma) [14]. Gravity modelling indicates plutons with a tabular form and a thickness of up to 9 km, with deeper conduits to the south of the Dartmoor, Bodmin Moor and St Austell Granites [95]. Two mica, peraluminous, coarse-grained granite predominates and has initial 87Sr/86Sr ratios of 0·710–0·716 and ɛNd values of -4·7 to -7·1 [22]. A dominant lower crustal source is implied, but a mantle component has been inferred on the basis of whole-rock geochemistry, Sm-Nd isotope systematics [22], enclave compositions [93], a close spatial and temporal association with lamprophyres and basalts [56], and the helium isotope characteristics of inclusion fluids [90]. Emplacement post-dates the D3 plastic-brittle structures generated during the extensional reactivation of thrust faults [1,2,54]. Magmatic fabrics are often compatible with moderate NW–SE extension of magma batches [10,54,67]. There is a clear spatial association between the granite batholith and W-As-Sn-Cu-Zn mineralisation [86]. Most mineral production was from tourmaline–quartz breccia veins (Sn) and chlorite–tourmaline–sulphide veins (Sn, Cu, As, Zn, Pb) [86]; in most areas these are hosted by steeply dipping extensional faults and/or tensile fractures that formed in response to continued NNW–SSE to north–south extension [88–90].
5 Tectonic evolution
5.1 Rifting and passive margin development
The non-marine sedimentary and associated igneous rocks of the Looe Basin (Dartmouth Group) indicate that rifting of normal thickness continental lithosphere had initiated by the Late Lochkovian and had brought about sufficient thinning to form a south facing shallow marine shelf (Meadfoot Group) by the Pragian [58,60,65]. To the south, exhumation of the Lizard mantle peridotites probably also commenced in the Early Devonian; the Traboe Cumulate Complex was emplaced, close to the base of the continental crust, and experienced rift-related granulite facies partial melting by the Latest Emsian [16,18]. N-MORB basalts and dolerites (Landewednack Amphibolites) underwent high temperature metamorphism due to shear zone juxtaposition against exhumed mantle during the Eifelian [19,69]. Further north, Early Eifelian T-MORB pillow lavas and radiolarian cherts, formed in proximity to highly attenuated continental lithosphere (base Veryan Nappe and clasts within olistostrome). The above, and consideration of the onset of convergence, suggests that the distal passive margin reached its maximum width in the Eifelian, during formation of the Crousa Gabbro and dolerite dykes. Mylonitic fabrics in the mantle peridotites, Traboe Cumulate Complex, Landewednack-Porthoustock amphibolites and Crousa Gabbros, and the orientation of dolerite dykes, are all broadly consistent with (E)NE-(W)SW lithospheric extension during continental rifting, mantle exhumation and early sea-floor spreading [3,18,19,41,75].
Asymmetrical extension in a non-volcanic margin setting, prior to the opening of a short-lived spreading centre has been proposed [18,19]. Such a model supports earlier suggestions that the Lizard ophiolite formed in a narrow oceanic basin, like the Red Sea [23] or Gulf of California [34], and has many similarities with Ligurian ophiolites [73]. There is no evidence of a supra-subduction zone (SSZ) setting [18,34,75]. The high temperature extensional deformation of the Crousa Gabbro [75], shear zone hosted Fe-Ti oxide gabbros [48], multiple dyke (magma) batches with distinct compositions [53,75], emplacement of gabbro and dolerite sheets in exhumed peridotite [3,75], juxtaposition of upper oceanic crust against exhumed mantle [19] and a relatively fertile spinel-plagioclase lherzolite with evidence of melt infiltration/melt-rock interaction [18,23,50] are also features described from slow spreading ridges.
5.2 Convergence
The northwest-directed thrusting of mantle peridotites over the Landewednack Amphibolites had commenced by the time syn-kinematic bimodal melts (Kennack Gneiss) were emplaced into the boundary zone. The continental crust source component of the felsic gneiss implies that mantle peridotite had been thrust over pre-rift continental lithosphere and/or syn-convergence sedimentary rocks [82], although they were not necessarily widely separated prior to the onset of convergence. Determinations of emplacement age vary from Early Eifelian to Late Frasnian [17,69,82], whilst the lower age of syn-convergence amphibolite facies metamorphism is Famennian [80,82]. A clast of Early Famennian granite (U-Pb zircon age of 373 ± 6 (2σ) Ma [28] within the Veryan Nappe olistostrome attests to at least local syn-convergence magmatism in the upper plate; the olistrostrome also hosts syn-convergent magmatic rocks [57]. Sedimentological and provenance data from the Gramscatho Group, and the geochronology of Lizard Complex magmatism and metamorphism, are consistent with the progressive, predominantly frontal, accretion of the distal passive margin during the Givetian to Famennian [44,57].
The onset of continental collision was marked by the emergence of nappes comprising deep marine sedimentary and volcanic rocks (Carrick and Veryan nappes), oceanic lithosphere and pre-rift basement (Lizard nappe) and upper plate high-grade gneisses (Normannian Nappe) onto the northern passive margin by the Earliest Carboniferous [44,57]. Deformation migrated through the passive margin during the Mississippian, inverting rift basins, including the southern part of the Carboniferous Culm Basin. The style of deformation, including the presence of facing confrontations, was strongly influenced by rift basin geometry [60]. The Culm Basin evolved from a rift basin to a Pennsylvanian thrust-top basin; its infill, derived from the east and then the north, indicating the influence of uplift caused by frontal thrusting and strike-slip along the Bristol Channel-Bray Fault [57]. “Docking” with southern Britain resulted in Late Westphalian D2 deformation across SW England and contemporaneous inversion of the Culm and North Devon basins.
5.3 Extensional reactivation
Variscan convergence across southern Britain ceased during the Latest Carboniferous (c. 305–300 Ma) and was post-dated by a NNW-SSE extensional regime (D3) that persisted through much of the Early Permian [29,89]. Early post-convergence extension brought about reactivation of the Rhenohercynian suture and subjacent D1/D2 thrust faults in the orogenic wedge and was associated with a dominant top sense of shear to the SSE [1,2]. Anomalous zones of steeply dipping primary/secondary cleavage, notably the ‘Start-Perranporth Zone, were formed by D3 backfolding associated with thrust fault reactivation and forced folding above extensionally reactivated faults in pre-Devonian basement [57]. Significant extensional/erosional thinning and exhumation of the lower plate occurred prior to the initiation of Early Permian bimodal magmatism at c. 295 Ma. Whilst Variscan convergence brought about accretion and inversion of a thermally young passive margin, that increased the transient geothermal gradient within the lower plate, mantle derived melts underplated and/or injected during extension provided the heat to generate an anomalously large volume of granite over 25 Ma [22,90]. Steeply dipping fracture networks, in both granites and their host rocks, hosting magmatic-hydrothermal Sn-Cu mineralization, reflect continued NNW-SSE extension until c. 275 Ma [89]. The early infill of the Plymouth Bay Basin formed above the subsiding upper plate during extensional reactivation of the Rhenohercynian suture [77].
6 Wider implications and unresolved issues
The Upper Palaeozoic geological evolution of SW England provides important constraint on Variscan tectonic models due to its location between Avalonia and Armorica, and hence proximity to the Rhenohercynian and Rheic suture(s). There are strong similarities with mainland Europe, where Late Devonian convergence along the active northern margin of the Saxothuringian Zone (Mid-German Crystalline High) resulted in continental collision and progressive inversion of the Devonian (Rhenohercynian) passive margin during the Carboniferous [38,70]. The westwards correlation of the Rhenohercynian suture, across the Bristol Channel-Bray Fault, to SW England appears justified [38,45,62]. The Rheic suture is also placed immediately south of SW England and is coincident with the Rhenohercynian suture in most tectonic reconstructions [62,64,97]. However, limited knowledge of both the pre-Devonian geology of SW England, and hence its Avalonian affinities, and the pre-Permian offshore geology between Britain and France results in considerable uncertainty. These and other outstanding issues are discussed below.
6.1 Is SW England part of Avalonia?
SW England (Fig. 6) is bounded, offshore to the south, by a reactivated Late Devonian Rhenohercynian suture with the upper plate Normannian Terrane, and to the north and east by the Bristol Channel-Bray Fault [25] that brought about Late Westphalian juxtaposition against unequivocal Avalonian lithosphere [58]. There are no Lower Palaeozoic palaeobiogeographical or palaeomagnetic data for SW England. The only exposed pre-Devonian rocks, the Man of War Gneiss and Old Lizard Head Formation (Lizard Complex), have Late Cambrian arc affinities characteristic of peri-Gondwanan terranes [68,81], but it is unclear whether they are derived from the lower or upper plate.
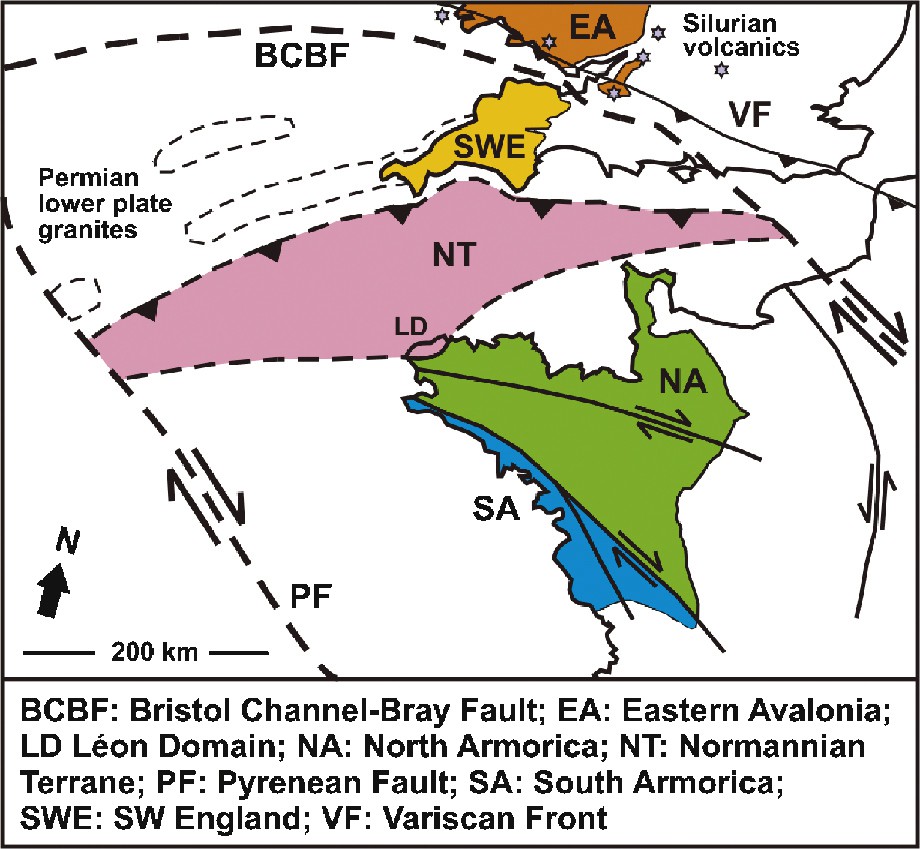
Simplified tectonic map of southern Britain and NW France showing the key elements that are discussed in the text and form the basis for models in Fig. 7; modified after [30,45,31,71].
Fig. 6. Carte tectonique simplifiée du Sud de la Grande Bretagne et du Nord-Ouest de la France montrant les éléments clés discutés dans le texte et qui servent de base aux modèles de la Fig. 7 ; modifié d’après [30,45,31,71].
Lower plate crust was indirectly sampled, and mixed with a juvenile mantle-derived component, during formation of the Kennack Gneiss (granitic facies) that has ɛNd (376 Ma) values of +0.7 to −3.0 and TDM model ages of 849–1266 Ma [82]; individual zircons range from 490 Ma to greater than 1700 Ma [69]. The Early Permian SW England granites also indirectly sample lower plate crust and have ɛNd (290 Ma) values of −4.7 to −7.1 and TDM model ages of 1271–1788 Ma [22]. The zircon data from the Kennack Gneiss indicate a significant Mesoproterozoic source component, typical of Avalonian basement [79] and the TDM model ages from both the Kennack Gneiss and SW England granites are compatible with this (dependent upon the proportion of admixed juvenile mantle-derived component). However, the age corrected ɛNd values for the Kennack Gneiss and, in particular, the SW England granites are rather more negative than typical Avalonian basement and overlap with values for Armorica/Saxothuringia [68].
North and east of the Bristol Channel-Bray Fault, rocks of Avalonian affinity form most of the lower plate [38]. SW England occupies a similar position, north of the Rheic and/or Rhenohercynian suture(s), and hence also probably represents the southern margin of Avalonia. However, as no data unambiguously confirm the presence of Avalonian basement, a pre-Devonian origin as a separate Gondwana-derived terrane cannot be excluded [25].
6.2 What is the affinity of the upper plate (Normannian Terrane)?
The upper plate Normannian Terrane is largely confined to the English Channel (Fig. 6) and hence is poorly constrained. The only exposure along its northern margin is the garnetiferous granite gneiss of Eddystone Rock that has a Frasnian K-Ar biotite cooling age [66]. Exploration well, 87/12-1A, located 55 km southeast of the Lizard, penetrated 479 m of Middle Devonian strata that was dominated by Givetian limestone and overlain by a “shallow water” siliciclastic succession [30]. Clasts within the southerly derived Gramscatho Basin olistostromes (Section 3.1) provide additional data. Ordovician quartzites have an undisputed Armorican fauna [78]. A greywacke (presumed resedimented) has yielded a laser-probe 40Ar-39Ar whole-rock plateau age of 415 ± 2 Ma (Pridoli) [15] and is similar to the 40Ar-39Ar whole-rock plateau age of 420–410 Ma (Ludlow-Pridoli) obtained from a cleaved metasiltstone clast [32]. Gramscatho Group sandstones in the Carrick Nappe (Portscatho Fomation) indicate a dominant continental magmatic arc source and have ɛNd (370 Ma) values of −4.3 to −10.3 and TDM model ages between 1177–1658 Ma [35]. Two granite clasts, with volcanic arc or syncollisional geochemistries, have yielded magmatic U-Pb zircon ages of 422 ± 4 (2σ) Ma (Ludlow) and 373 ± 6 (2σ) Ma (Early Famennian); upper discordia intercept ages of 2445 Ma and 2606 Ma suggest a Late Archean or Early Proterozoic source component [28].
The southern margin of the Normannian Terrane may be represented by the Léon Domain in northern Brittany [31,85,92]. These rocks have affinities with the Saxothuringian Zone and have undergone Variscan deformation and metamorphism [85]. Partially retrogressed eclogites, derived from N-MORB, may have been metamorphosed as early as 439 ± 12 Ma (Early Silurian), but possibly later [85].
The above data indicate that the Normannian Terrane probably originated as part of the Armorican Terrane Assemblage. Clasts sourced from its northern margin, in the Upper Devonian, also suggest Ludlow and Famennian continental arc magmatism and Ludlow-Pridoli cleavage development [15,28,32]. These data are limited and could reflect either discrete magmatic/deformation episodes or incomplete sampling of more or less continuous magmatism and accretion-related deformation. In addition, the possibility that some source components might be derived from accreted lower plate material cannot be excluded. However, east of the Bristol Channel-Bray Fault, data from the Mid-German Crystalline High are more abundant and compatible with separate episodes of Silurian and Late Devonian-Carboniferous continental arc magmatism [38]. Middle Devonian limestones in the upper part of the Normannian Terrane (well 87/12-1A) are also matched by limestones of similar age overlying arc rocks of the Mid-German Crystalline High in Germany (Saar 1 borehole) [38,45].
Although the Normannian/Léon Terrane originated as part of the Armorican Terrane Assemblage, it might not have been a contiguous part of Armorica sensu stricto during the Silurian-Devonian. The Léon Domain eclogites have been variably interpreted as:
Similar ambiguities occur east of the Bristol Channel-Bray Fault, where the Vesser Rift separated the Mid-German Crystalline High from the remainder of the Saxothuringian Zone to the south [51].
6.3 The SW England Rhenohercynian passive margin and the Rheic Ocean
The relationship between the development and subsequent inversion of the Rhenohercynian passive margin and the closure of the Rheic Ocean is poorly constrained (Fig. 7). The Rhenohercynian and Rheic sutures could be:
- • (A) temporally and spatially coincident, i.e. the Rhenohercynian passive margin represented the northern margin of the Rheic Ocean [31];
- • (B) spatially coincident but temporally separated, i.e. the Rhenohercynian passive margin formed in a successor basin after the closure of the Rheic Ocean [38];
- • (C) spatially ± temporally separated, i.e. the Rhenohercynian passive margin developed in a marginal basin to the Rheic Ocean [92].
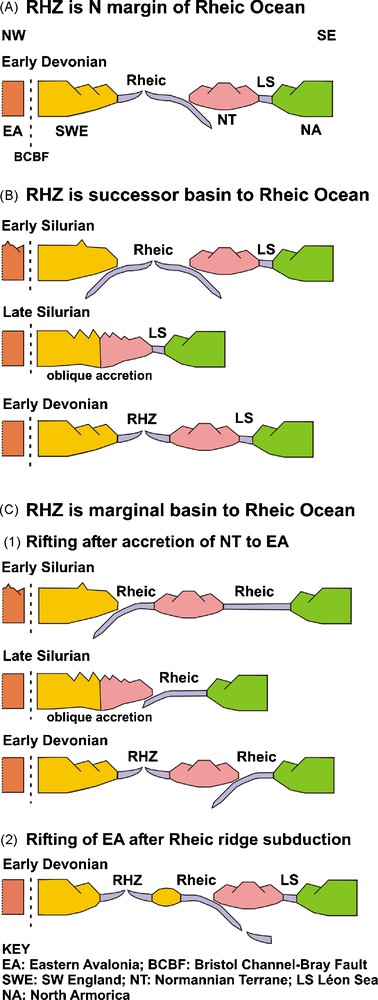
Cartoon showing some of possible relations between the development of the SW England Rhenohercynian passive margin and the Rheic Ocean; in part after [31,38,92].
Fig. 7. Schéma montrant les relations possibles entre le développement de la marge passive Rhénohercynienne du Sud-Ouest de l’Angleterre et l’océan Rhéïque ; en partie d’après [31,38,92].
Model A is considered unlikely. SW England provides evidence for the Lochkovian-Eifelian development of a passive margin associated with an incipient or small ocean (Section 6.1). It is difficult to reconcile these data with interpretation of the Lizard ophiolite as:
- • a late formed segment of Rheic Ocean (ridge push forces should suppress coeval rifting of the adjacent passive margin, the Rheic passive margin should have developed much earlier);
- • an oceanic crust formed in a forearc setting above the subducting Rheic Ocean (no indication of SSZ geochemistry).
In Model B, Rhenohercynian rifting occurred close to a Late Silurian-Earliest Devonian suture between Eastern Avalonia and the Armorican Terrane Assemblage that resulted from northwards subduction and closure of the Rheic Ocean [38]. One possible expression of Silurian northwards-directed subduction of the Rheic Ocean below southern Britain is the east-west trending zone of Llandovery intraplate magmatism (Fig. 6) that occurs north and south of the Variscan Front [71]. It might conceivably represent the northern margin of a short-lived Early Silurian back arc rift. Any associated upper plate magmatic arc would be hidden below the post-Silurian cover of SW England. The Ludlow-Pridoli regional metamorphism [15,32] and magmatism [28] inferred from the Normannian Terrane might record its Silurian oblique accretion to SW England but the magmatism would also require southwards subduction of Rheic Ocean below the Normannian Terrane. If the Normannian Terrane was a contiguous part of the Armorican Terrane Assemblage, the Rheic Ocean closed during this event.
In Model C, the Rhenohercynian passive margin developed in a marginal basin setting to the Rheic Ocean. There are two possibilities. Model C1 is a modification of Model B where the Normannian Terrane was not a contiguous part of the Armorican Terrane Assemblage. Continued northwards subduction of the Rheic Ocean outboard (south) of the accreted Normannian Terrane was accompanied by slab roll back and upper plate extension. In this scenario, the Rheic suture would be preserved within the Léon Domain [85,92]. In Model C2, the Rhenohercynian marginal basin formed entirely within the southern Avalonian margin, as a consequence of slab pull forces established following southwards subduction of the Rheic Ocean ridge [92]. In these models, marginal basin (Lizard) oceanic lithosphere is separated by a narrow strip of either Armorican (C1) or Avalonian (C2) continental lithosphere from the closing Rheic Ocean further south.
All of the remaining models (B, C1, C2) are broadly admissible with available data from SW England (Fig. 7), although numerous details are necessarily speculative. No model accounts for all aspects and there are presently insufficient data to adequately test between them. Most palaeogeographical reconstructions consider a faunal barrier, implied to be the narrowed Rheic Ocean, persisted between Armorica and Avalonia during the Early Devonian e.g [64], which would mitigate against Model B. Early Devonian palaeomagnetic poles from South Wales (Lat. 7°S, Long. 307°E) [13] and northern Armorica (Lat. 13 °S, Long. 315°E) [94] (but see also [74]) suggest a separation of ∼1100 km at the onset of Rhenohercynian rifting. Whilst the Normannian Terrane has Armorican affinities that would support Model C1, the possibility that Avalonian crust from the southern side of the Rhenohercynian marginal basin (Model C2) was accreted to, or subducted below it, during closure of the Rheic Ocean [92] cannot be precluded. Indeed, the ɛNd data and TDM model ages for lower plate SW England granites and upper plate sourced Gramscatho Group sandstones are very similar. These outstanding issues will be best addressed through more widespread application of U-Pb zircon dating provenance methods to clasts from the Gramscatho Basin olistostromes.
The (E)NE–(W)SW extension, implied by Lizard Complex mantle peridotite fabrics and dyke orientations, is oblique to the east-west trending Rhenohercynian Zone and has been used to infer a dextral transform origin for Model A [7,18,19] that could also be applied to models B and C. However, this model contrasts with the orthogonal rift geometry implied by the development of the passive margin further north [57]. Palaeomagmetic relations are ambiguous [42] and the possibility of vertical axis rotation of the entire Lizard Complex, prior to final emplacement, is a possibility in view of an apparent 35° clockwise rotation with respect to remanent magnetisation within rocks of similar age to the north [9].
6.4 Processes causing rifting
Development of the SW England passive margin represents two or three tectonic episodes. Lochkovian to Emsian rifting was followed by a short period of sea-floor spreading within the Emsian-Eifelian. Its duration may have been only 10 Ma, but even at a very slow spreading rate of 20 mm/yr could still have generated ∼200 km of ocean floor. The Emsian hiatus within the Staddon Formation (Section 2.3) may reflect the onset of sea-floor spreading. Ridge push forces generated during this short interval may have contributed towards Acadian deformation [97] north of Variscan Front but this appears slightly younger and would also require deformation to have been effectively be partitioned away from the passive margin. Subsequent Late Eifelian to Famennian rifting was coeval with convergence further south and may have been driven by slab pull following subduction of the Lizard ocean ridge. Rift-related magmatism and basin formation persisted in the lower plate to the Visean [57]. The reason for this is unclear. Convergence, following initial accretion of the distal passive margin may have been punctuated by slab detachment [84].
6.5 Significance of Bristol Channel-Bray Fault
The Bristol Channel-Bray Fault is a major structure in the northern Variscides [45]. A dextral displacement of up to several hundred km during the Carboniferous can account for the absence of Acadian (Eifelian) deformation in SW England [97] and the anomalous provenance of Late Carboniferous foreland successions [91]. It may also account for along-strike variations in the Rhenohercynian Zone. The SW England passive margin is somewhat narrower than in Germany, and this might be due to its excision along the fault [97]. The generation and anomalous thickness of the Cornubian Batholith was strongly influenced by extensional reactivation of the Rhenohercynian suture and footwall, possibly during dextral transtension between the NW-SE trending Bristol Channel-Bray Fault and the Pyrenean Fault [4].
7 Conclusions
The SW England Rhenohercynian lower plate passive margin developed during Lochkovian-Eifelian rifting. Evidence for pre-rift Avalonian basement is equivocal and SW England may represent a separate Lower Palaeozoic terrane.
The Lizard ophiolite preserves exhumed mantle and early-formed oceanic crust (probably Eifelian) that is compatible with formation of a small ocean basin. It is unlikely to represent a fragment of Rheic Ocean or associated forearc (SSZ) ocean crust.
The upper plate, represented by the Normannian/Léon Terrane, has Armorican affinities and can be correlated with the Mid-German Crystalline High of the Saxothuringian Zone. It may have previously docked with SW England in the Silurian.
The Rhenohercynian passive margin probably developed within a marginal basin north of the Rheic Ocean or, possibly, a successor basin following its closure. The Rheic and Rhenohercynian sutures may be coincident or the Rheic suture may be located further south in the Léon Domain. There is presently insufficient data to test between these models.
Convergence initiated in the Late Eifelian, following closure of the Rheic Ocean, and included the Upper Devonian frontal accretion of elements of the distal passive margin. Collision was accompanied by inversion of the passive margin basins and was strongly influenced by rift basin geometry, resulting in local facing confrontations.
Juxtaposition of SW England with southern Britain occurred during dextral displacement on the Bristol Channel-Bray Fault during Carboniferous convergence.
Latest Carboniferous-Early Permian extensional reactivation of the suture and accretionary wedge brought about thinning and exhumation of the lower plate and was accompanied by bimodal magmatism, including the generation and emplacement of the 400 km Cornubian Batholith. Upper plate sedimentary basins were developed coevally above the reactivated suture.
Acknowledgements
We thank Karel Schulmann for encouraging our participation in the Orléans meeting and this volume. Martin Holder made a significant contribution to developing our understanding of SW England passive margin evolution. Wolfgang Franke has provided inspiration and hospitality in Germany over the last 25 years. Duncan Pirrie and Peter Frost provided support in Scotland during writing. Thanks to Rob Strachan and Nigel Woodcock for constructive reviews. This article is published with the approval of the Executive Director of the British Geological Survey (NERC).