1 Introduction
The pre-Upper Ordovician sequence of the Pyrenees constitutes a thick (3000 m) succession of metasedimentary rocks that crop out extensively in the central part of the chain (Fig. 1). Classically, it was divided into two parts. Its lower part (1500 m thick) is lithologically heterogeneous and made up of metapelites and metagreywackes interbedded with numerous layers of marbles, quartzites and calc-silicates (“Série de Canaveilles”, Cavet, 1957; Canaveilles Group, Laumonier, 1988). It includes syn-sedimentary bimodal metavolcanic rocks (Ayora and Casas, 1986; Navidad and Carreras, 2002). The upper part is a rather monotonous, 1500 m thick, succession composed of a rhythmic alternation of sandstones, siltstones and argillites (“Capas de Planoles”, Fontboté, 1949; “Schistes de Jujols”, Cavet, 1957; Jujols Group, Laumonier, 1988). In contrast to the lower sequence, no metavolcanic intercalations have been found in the upper part. A discontinuous intercalation of carbonate and black phyllites may correspond to the contact with the lower part of the succession. The age of the pre-Upper Ordovician sequence has been a subject of debate owing to its unfossiliferous character. According to its stratigraphic position, underlying the fossiliferous Upper Ordovician, the sequence was correlated with the Cambrian and Ordovician successions of the southern slope of the Montagne Noire, and a Cambrian age was attributed to the “Série de Canaveilles”, and an Ordovician age sensu lato to the “Schistes de Jujols” (Cavet, 1957; Fontboté, 1949). In the study area an erosive angular unconformity (Fig. 2) separates the Jujols Group from a well-dated Upper Ordovician succession (Casas and Fernández, 2007; García-Sansegundo et al., 2004; Santanach, 1972;). Recently, volcanic rocks have yielded Late Neoproterozoic-Earliest Cambrian ages (SHRIMP U-Pb in zircon; 581 ± 10 Ma, Cocherie et al., 2005; ∼540 Ma, Castiñeiras et al., 2008) for the lower part of the succession. On the other hand, on the basis of lithostratigraphic correlations, a Mid-Late Cambrian age (Abad, 1987; Laumonier, 1988) or Late Cambrian/Early Ordovician age (Guitard et al., 1998) has been proposed for the upper part of the sequence. Early Cambrian fossils have been found in an isolated outcrop bounded by Alpine faults in the Eastern Pyrenees (Abad, 1988; Perejón et al., 1994). However, the lack of continuity of this outcrop with the neighbouring massifs precludes any correlation with the pre-Upper Ordovician successions of the Pyrenees. In the Mouthoumet massif, north of the Pyrenees and south of the Montagne Noire, Baudelot and Bessière (1975, 1977) and Cocchio (1982) attributed an Early Ordovician age (Tremadocian-Arenigian) to detrital formations on the basis of abundant microfossils (acritarchs). These authors highlight the similarities between the acritarch assemblages described in the Mouthoumet massif with that described in the Montage Noire. In this paper, we present the first biostratigraphic age data on the pre-Upper Ordovician succession of the Pyrenees based on organic-walled microfossils (acritarchs). Our results improve our understanding of the affinity of the pre-Upper Ordovician rocks of the Pyrenees with those from other fragments of the Variscan orogen, and provide new data on the age of the pre-Variscan deformations in the Pyrenees.

Simplified geological map of the central part of the Pyrenees with the location of study area (Fig. 3).
Carte géologique simplifiée de la partie centrale des Pyrénées avec la localisation de la région étudiée (Fig. 3).
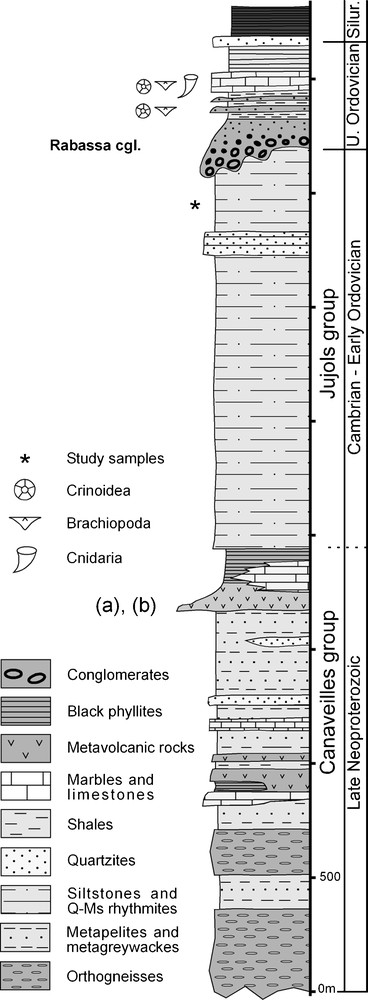
Synthetic stratigraphic column of pre-Silurian rocks of the Canigó massif with the location of the study samples and the dated volcanic rocks: (a) Cocherie et al., 2005; (b) Castiñeiras et al., 2008. Data from Guitard (1970), Hartevelt (1970), Santanach (1972) and Ayora and Casas (1986).
Colonne stratigraphique synthétique des roches pré-Silurien du massif du Canigou, avec la localisation des échantillons étudiés et des roches volcaniques datées : (a) Cocherie et al., 2005 ; (b) Castiñeiras et al., 2008. D’après Guitard (1970), Hartevelt (1970), Santanach (1972) et Ayora and Casas (1986).
2 Geological setting and samples
Samples were collected near the village of La Molina, on the southern slope of the Canigó massif, near the Upper Ordovician unconformity, which occurs in the upper part of the Jujols Group (Fig. 1–3). In this area, the Jujols Group consists of a rhythmic alternation of sandstones, siltstones and argillite layers, 1 mm to several centimetres thick. Layers range in colour from grey to a characteristic light green or light brown. Quartzitic sandstones up to 1 m in thickness occur at the top of the succession, exhibiting graded bedding, load casts, cross-bedding and fluid escape structures.
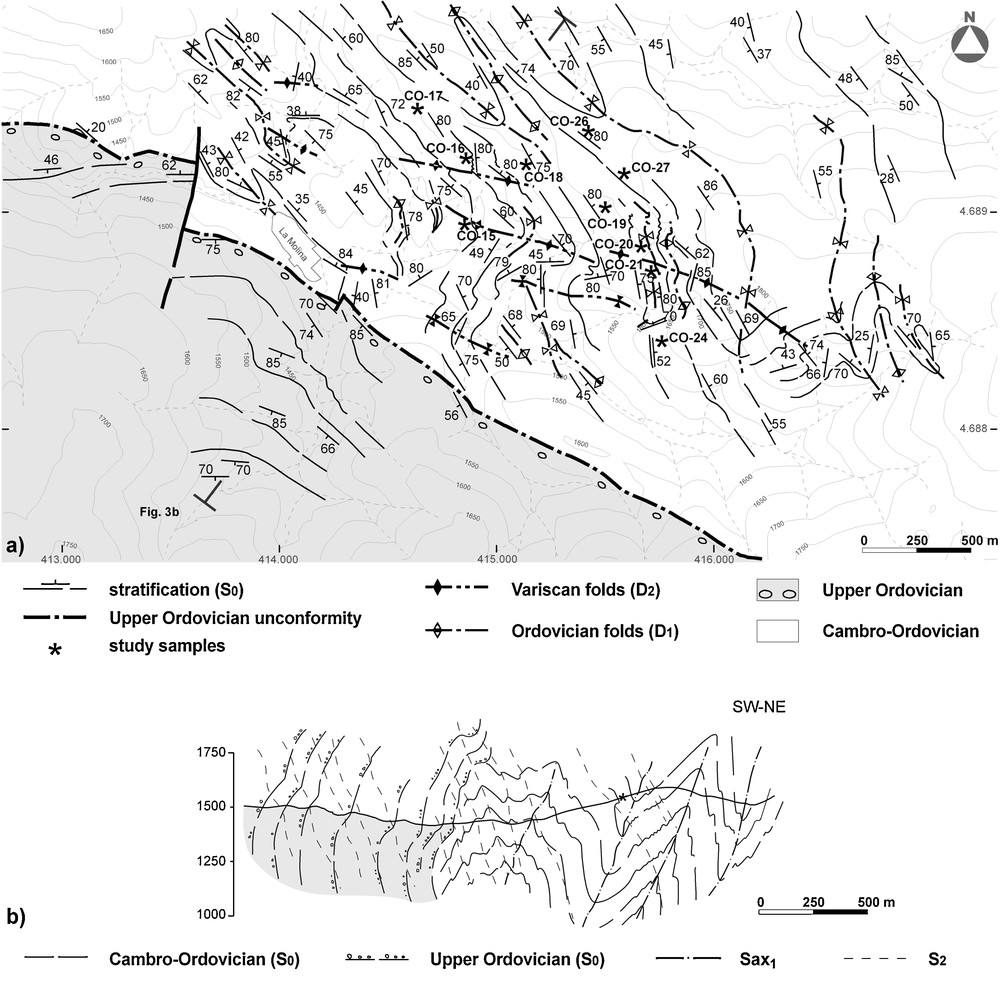
a: Geological sketch of the La Molina area showing the location of Fig. 3b; b: Cross-section through the study area. Fig. 1 for location.
a : Schéma géologique de la région étudiée montrant la situation de la Fig. 3b ; b : Coupe géologique de la région étudiée. Fig. 1 pour la localisation.
In this area, the pre-Upper Ordovician succession exhibits a complex structure, owing to the superposition of two Variscan folding phases; D2 and D3, over a D1 Ordovician folding episode (Casas, 2010). A series of D1 anticlines and synclines with subvertical axial surfaces and subhorizontal NW-SE axes affect the pre-Upper Ordovician rhythmites (Fig. 3). These folds are responsible for the NW-SE trending and the north-east and south-west dipping of the bedding and are, in turn, deformed by the D2 structures.
Sampling was undertaken in the southern limb of a D1 fold (Fig. 3) in beds located approximately 250 m below the Upper Ordovician unconformity. From the collected material, rock samples of ca. 50 gram were treated with standard acid digestion palynological procedures (see Vidal, 1988). The residue was mounted on palynological slides using PetroPoxy resin and studied under transmitted light with a ZeissAxio Imaginer M1 microscope with a computerized Axiocam Hrc microcamera. Palynological slides containing figured and representative material are stored with the collections of the Area de Paleontología de la Universidad de Extremadura, Badajoz with accession numbers UEXP217O-15 to 27 and UEXP918FL10-6-2.
3 Acritarch biostratigraphy
A scarce material of acritarchs was recovered from ten of the 14 analyzed samples, with only samples CO-15 and CO-19 containing more than ten moderately preserved specimens. The material is poorly preserved, black, with little vesicle surface detail and broken processes, which makes determination at genus or species-level problematic. However, on the basis of vesicle shape, much of the material can be assigned to informal acritarch subgroups, with the recognition of acanthomorph and diacromorph acritarchs (sensu Downie et al., 1963), and galeate acritarchs (sensu Servais and Eiserhardt, 1995). The samples CO-17, CO-18, CO-26 and CO-27 yielded only acanthomorph acritarchs, the sample CO-15 mainly galeate acritarchs and scarce acanthomorphs, and the samples CO-19, CO-20, CO-21 and CO-24 contain diacromorph and acanthomorph acritarchs. The acanthomorph acritarchs in these samples cannot be taxonomically identified and provide no biostratigraphical information. In contrast, diacromorph and galeate acritarchs have restricted stratigraphical ranges, as discussed below.
3.1 Diacromorph acritarchs
The informal subgroup Diacromorphitae was defined by Downie et al. (1963) to include all acritarchs exhibiting a bipolar central body with ornaments at each pole (e.g. Raevskaya and Servais, 2009). Specimens with similar ornamentation at each pole (Fig. 4A, B) can be tentatively assigned to the genus Acanthodiacrodium Timofeev 1958. The specimen in Fig 4A shows morphological features similar to A. angustum, a species which in NW Gondwana is restricted to the Tremadocian (Vecoli and Le Hérissé, 2004, fig. 9), although the poor preservation prevents any confident assignment to this species. Another diacrodian acritarch (Fig. 4B) has a broken vesicle but apparently the number of processes is similar at each pole. The specimen illustrated in Fig. 4C has different processes at each pole, but the distal end of the processes is not preserved and it is not possible to assign it to Dasydiacrodium Timofeev 1959, Arbusculidium Deunff 1968, or to another genus.
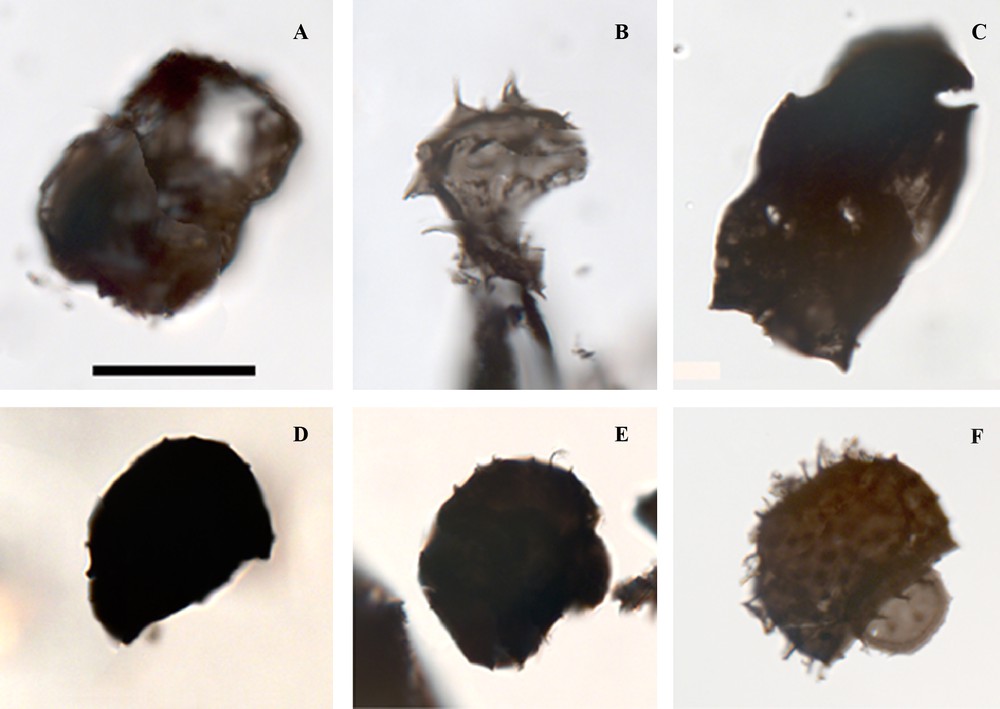
Acritarchs from the Jujols Group, except F. In this figure is given sample number and England finder coordinates. Scale bar is equivalent to 20 microns. A–C. Diacromoph Acritarchs. A. Acantodiacrodium sp. UEXP2517CO-24-1, H-29-1-2. Metamorphosed specimen close to A. angustum (Downie) Combaz 1967. B. Acantodiacrodium? sp. UEXP217CO-20-2, L-43-1. C. Diacrodian acritarch. UEXP217CO-19-1, R-16-2. D. Galeate acritarch. UEXP217CO-15-2, E-30-1. E. Galeate acritarch, UEXP217CO-15-2, T-27-1. F. Stelliferidium trifidum (Rasul) Fensome et al. (1990). UEXP918FL10-6-2, U-34. Specimen from the Barriga Formation, Tremadocian-Floian of the Ossa Morena Zone, South-West Spain.
Acritarches du Groupe de Jujols, à l’exception de F. Dans cette figure, on donne les numéros des échantillons et les coordonnées England finder. La barre d’échelle est équivalente á 20 μm. A–C. Acritarches diacromophes A. Acanthodiacrodium sp. UEXP217CO-24-1, H-29-1-2. Échantillon métamorphisé semblable à A. angustum (Downie) Combaz 1967. B. Acanthodiacrodium? sp. UEXP217CO-20-2, L-43-1. C. Acritarche diacrodian. UEXP217CO-19-1, R-16-2. D. Acritarche galéate. UEXP255CO-15-2, E-30-1. E. Acritarche galéate, UEXP217CO-15-2, T-27-1. F. Stelliferidium trifidum. (Rasul) Fensome et al.1990 UEXP918FL10-6-2, U-34. Spécimen de la Formation Barriga, Trémadocien-Floian de la zone d’Ossa Morena, Sud-Ouest de l’Espagne.
The oldest occurrence of diacrodian acritarchs is documented from the Late Cambrian P. spinulosa trilobite Biozone in eastern Newfoundland (e.g. Martin and Dean, 1988). Diacromorphs remained rare in the Late Cambrian (Furongian) to become abundant and highly diversified during the Early Ordovician, where they constitute a major component of acritarch assemblages during the Tremadocian (e.g. Molyneux et al., 1996, fig. 6; Vecoli and Le Hérissé, 2004, fig. 4).
3.2 Galeate acritarchs
Servais and Eiserhardt (1995) introduced the term “galeate acritarchs” for acritarchs with a hemispherical vesicle outline and a large polar opening closed by an operculum. Genera belonging to this plexus are differentiated on the basis of vesicle ornamentation and/or the nature of the processes. The morphological characters of the specimens illustrated in Fig. 4D and E identified them as galeate acritarchs. The two main galeate genera are Stelliferidium (with star-shaped striae on the base of the processes) and Cymatiogalea (with polygonal arrangment of the surface ornamentation). However, poor preservation prevents us from differentiating these characters in the present material.
The Galeate acritarch illustrated in Fig. 4E shows an operculum, fine processes and dimensions comparable to those of several species of the genus Stelliferidium, such as Stelliferidium trifidum (Fig. 4F) from the Upper Tremadocian-Floian, or S. furcatum from the Tremadocian. Stelliferidium ranges from the Furongian to the Middle Ordovician with maximum diversity in the Tremadocian-Floian (Vecoli and Le Hérissé, 2004, fig. 9). Compared with the two species of Stelliferidium that reach the Middle Ordovician, S. striatulum (Upper Tremadocian-Darriwillian) has broader processes and a large operculum and S. philippoti (Darriwillian) exhibits a larger operculum that differs from the material in the Jujols Group. In comparison with the Cymatiogalea species (Furongian-Middle Ordovician), the specimen illustrated in Fig. 4E has dimensions of processes similar to those of C. cristata (Tremadocian). However, all the species of Cymatiogalea that reach the Middle Ordovician have broader processes (C. granulata, C. ancora and C. catherinae).
Galeate acritarchs are biostratigraphically important in the Upper Cambrian and Lower Ordovician of peri-Gondwana and Baltica (Molyneux et al., 1996). The first appearance of the galeate plexus occurs at the beginning of the Late Cambrian in the East European Platform (Volkova, 1990). The plexus was abundant in the Latest Cambrian and a dominant constituent of Early Ordovician assemblages, especially in the Tremadoc-Floian time interval (Molyneux et al., 1996). In general, Stelliferidium, Cymatiogalea, and Acanthodiacrodium tend to dominate the early Tremadocian assemblages (Servais et al., 2004). The acritarch assemblage from the upper part of the Jujols group provides solid evidence for a Late Cambrian to Early Ordovician age and more probably for the Early Ordovician.
4 Discussion
The data presented here are the first biostratigraphic age data on the pre-Upper Ordovician succession of the Pyrenees. It should be noted that in neighbouring areas that are also constituted by rhythmites of the Jujols Group, i.e. the northern and eastern slopes of the Canigó massif, the search for microfossils has yielded no results (Cocchio, 1982).
These data suggest a Late Cambrian-Early Ordovician age for the uppermost part of the pre-Upper Ordovician succession in the study area. The Rabassa conglomerate, which constitutes the basal level of the Upper Ordovician sequence, has been attributed to the Sandbian (Hartevelt, 1970). Furthermore, abundant fossil content has allowed us, since the pioneering studies on Pyrenean geology, to attribute a “Caradoc–Asghill” age to the rest of the Upper Ordovician succession (see discussion in Gil-Peña et al., 2004). From these age constraints, it is reasonable to assume that most of the Lower and the entire Middle Ordovician sequences were eroded before the deposition of the Upper Ordovician rocks, or were not deposited in the eastern Pyrenees. Moreover, a Cambrian age can be attributed to the rest of the succession, which suggests that the Late Neoproterozoic/Cambrian boundary may be located in the discontinuous carbonate level at the top of the lower sequence (Fig. 2). Similarly, Middle Ordovician sequences are also absent in the Mouthoumet and the Montagne Noire massifs (Baudelot and Bessière, 1975, 1977; Cocchio, 1982).
The acritarch data confirm the existence of an unconformity at the base of the Upper Ordovician sequence evidenced to date only by structural and cartographic criteria (Casas and Fernández, 2007; García-Sansegundo et al., 2004; Santanach, 1972). It also lends support to the Middle Ordovician age assigned to the D1 deformation recognized on the southern slope of the Canigó massif. This deformational episode could be responsible for the formation of this unconformity (Casas, 2010).
The study area thus shows strong similarities with both the Iglesiente and Sarrabus regions in southern Sardinia, where an Upper Ordovician (Sardic) angular and erosional unconformity has also been described (Naud, 1981; Teichmüller, 1931). In Sardinia, the sequence below the unconformity is dated as Tremadocian (Barca et al., 1987; Pillola and Gutiérrez-Marco, 1988; Pillola et al., 1998) or Tremadocian-Early Floian? (Pillola et al., 2008), and as a result, the age of the Sardic deformation can be estimated between the Early Ordovician and the Sandbian. These similarities allow us to correlate the Upper Ordovician unconformity described in the Pyrenees with the Sardic Unconformity. In this way, the Pyrenees closely resemble the most external part of the Sardinian fragment of the Variscan orogen and exhibit marked differences with the rest of the Iberian Massif, where thick (up to 4500 m) Early Ordovician detrital sediments were deposited coevally with volcanism and plutonism (Díez Montes et al., 2010; Pérez-Estaún et al., 1990; Valverde-Vaquero et al., 2005) and where evidence for an Ordovician deformation is limited (Martínez-Catalán et al., 1992). This suggests that the western (Iberian Massif) and eastern parts (Pyrenees, Sardinia, Sicily and the Alps) of this fragment of the northern Gondwana margin have evolved differently since the Early Ordovician.
Acknowledgements
JMC acknowledges the support of the projects CGL-2007-66857CO2-02, CGL2010-21298 and Consolider-Ingenio 2010, under CSD2006-00041 “Topoiberia”. T.P. acknowledges the support of the project CGL-2008-04373 (co-financed by FEDER). Detailed comments of S. Jensen, O. Fatka, B. Lefebvre and D. Vizcaíno have improved a previous version of the manuscript.