1 Introduction
In recent decades, human activity has significantly changed the hydrology and chemistry of catchments by retaining rivers water in dams and by introducing large masses of fertilizers from agricultural, industrial and household wastes. Agricultural nutrient inputs and urban wastes are strong and increase with population growth. In the Mediterranean catchments, water river discharge is low and decreases with irrigation and climate change (Garcia-Ruiz et al., 2011; Ludwig et al., 2010). This context leads to an increase of river nitrogen and phosphorus concentrations (Abdelkader et al., 2012; Guasmi et al., 2010; Maane-Messai et al., 2010; Mouni et al., 2009). To fulfill the growing water needs, many dams have been built all around the Mediterranean Sea (see Lehner et al., 2011 for dam inventory). Dams may have a strong impact on the water and nutrient river discharge due to silicate and phosphorus retention within sediments and, but not always, due to nitrogen removing (Avilés and Niell, 2007; Dürr et al., 2009). Also, for specific irrigation dams, river nutrient discharge decreases with the increasing rate of water and nutrient uptake by crops (Wahby and Bishara, 1980). On another hand, nowadays in Algeria, the intercepted runoff in dams and weirs hold about 5.2 billion m3, which form 42% of total runoff. Because of the growing irrigation needs and population intake, it is yet planned to store in the few coming years about 7.5 billion m3, which represent 60% of the precipitation received in coastal catchments. Urban and agricultural nutrient inputs and water residence time within dams also lead to a change on the nutrients ratios, as the Redfield ratio (Ludwig et al., 2009). River nutrient discharge and nutrient ratios are known to play a special role in supporting the Mediterranean production (Béthoux et al., 2002) where the productive areas are limited to the adjacent coast (Bosc et al., 2004). However, there are few studies for the southern Mediterranean basins. In addition, data on river nutrient loading to the Mediterranean watersheds are also scarce and are missing in North-African countries and can bias the general picture (Ibáñez et al., 2008; Ludwig et al., 2009). Moreover, data on the distribution and fluxes of N, P and Si in coastal catchments of Algeria are inexistent, except the work of Khélifi-Touhami et al. (2006) that had only considered seasonal fluxes of inorganic N and PO4 from the Mafragh estuary outlet. In addition, most studies about impacts of dams and river export of nutrients to the sea do not take into account organic compounds. However, some studies reveal that, even if the dissolved organic nitrogen (DON) and dissolved organic phosphorus (DOP) are important components of riverine inputs and coastal catchments (Purvina et al., 2010; Wiegner et al., 2006), they are rarely considered within nutrient loadings pool. For example, Wiegner et al. (2006) reported that DON often dominates the total dissolved nitrogen (TDN), but yet it is not considered to affect coastal water quality because of its assumed refractory nature. The authors add that DON needs to be considered into coastal nitrogen loading budgets, because of its rapid bioavailability as well as its atmospheric deposition in watersheds that forms about 15–30% of the total bioavailable dissolved nitrogen (Whitall and Paerl, 2001). Again, even on a worldwide scale, DON and DOP have never been considered in dam budgeting studies and it is here the first attempt to consider these forms along the aquatic continuum of river systems.
Considering the severe lack of geochemical data for coastal river, this study aims to evaluate nutrient fluxes and dam effects on the biogeochemistry of N, P and Si in three representative coastal catchments of Algeria. Data analysis will focus on the effects of dams on nutrient retention and dissolved organic nutrient production and budgeting. The study on hand will consider, for the first time, the role of dams in producing DON and DOP and challenges to reveal that function.
2 Sampling sites
The three catchments have a total surface of 11 160 km2 with a population of about 2 million people. They are mainly submitted to household and agricultural wastes (Fig. 1) and heavily managed by several dams that retain about half of the precipitation wealth. The largest dam is Beni-Haroun (960 million m3 storage capacity) built on the Kebir-Rhumel River (KR; with a catchment of 8110 km2). It is mainly used for water transfer to specific drinking water and irrigation reservoirs. But currently, only one reservoir (20 million m3) is functional; it is allocated to drinking water. The diverted water is pumped irregularly, depending on water needs, at mid-distance from the two tributaries input areas within the upper dam. The water abstraction for irrigation is today limited to subsistent agricultural activities, even if it was planned to large-scale irrigation. The catchment of the Kebir western River (KW; 1900 km2) is very weakly populated, with only 30 inhabitants per km2 and it is managed by a dam that retains about 120 million m3. This dam is used for irrigation and drinking water. The lower part is affected by marine intrusion and functions in the dry period as a core estuarine environment. This estuarine area does not exceed several kilometers. The Zardaza dam (50 million m3) built on the Safsaf River (SF; with a catchment of 1250 km2) is mainly used for drinking water. In the KR catchment, the great dam of Beni-Haroun was built in 2005 to intercept runoffs from the two main branches of Rhumel and Kebir. The Rhumel branch (Fig. 1) flow is more important than that of Kebir, but it is heavily submitted to direct untreated wastewater originating from Constantine city (about 1 Million people). The KR dam receives at its south side direct wastewater from the surrounding city of Mila and several other villages. Downstream the dam, the catchment is mainly forested and very weakly urbanized. By contrast to the eastern branch that is a temporary stream, the western branch of KW is a permanent stream receiving relative large urban wastes from used hydrothermal sources. The lower area of KW catchment is weakly populated, but relatively occupied by agricultural lands. The SF dam is alimented by the western stream that flows weakly but permanently. The upper part of the SF catchment is however largely forested and the lower part, relatively urbanized, is mainly occupied by agricultural lands. The catchments receive an annual precipitation yield of about 400–700 mm, inducing large fluctuation in river flow. In summer period, all rivers fall almost dry at the entering of dams, while their exits continue to deliver low flows. The residence time, which has an important role in the impact of dams on water chemistry, was estimated (storage capacity/discharge; Rueda et al., 2006). The water residence time of the studied dams is largely variable according to the tributaries input, storage capacities and clogging rates: about 3.6 years in KR dam; 2.4 years in KW dam and only 0.08 year in SF dam because of its advanced clogging rate.
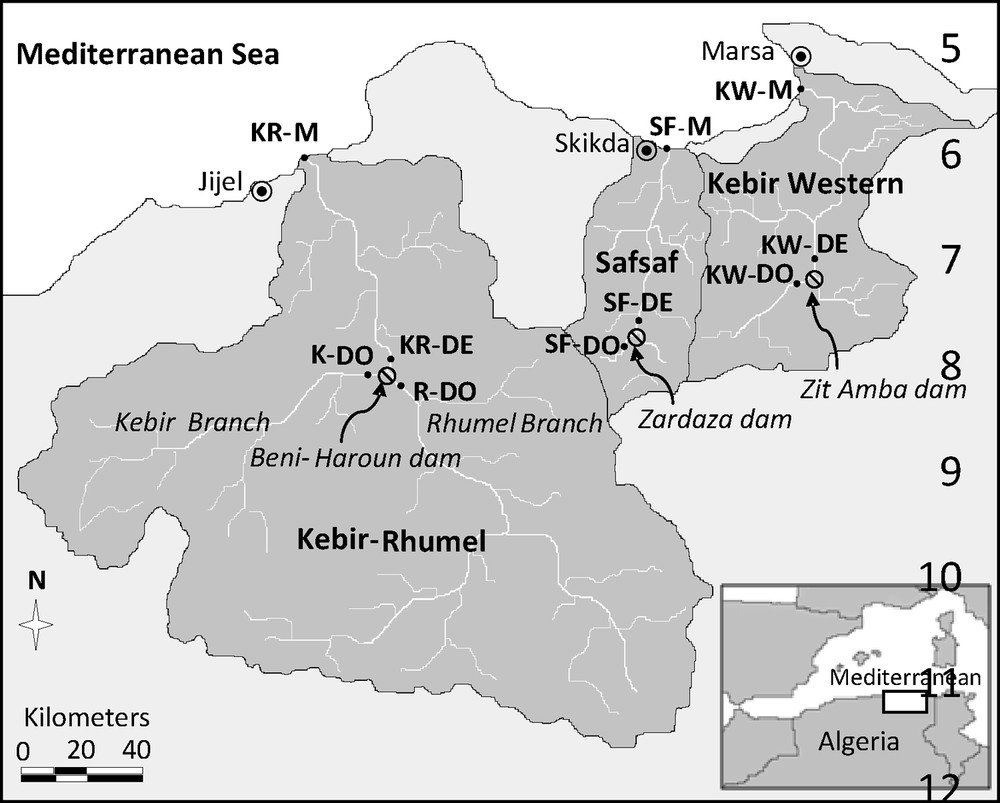
Sampling stations in the coastal rivers of Kebir-Rhumel (KR), Kebir West (KW) and Safsaf (SF). : dam. R-DO: Rhumel branch of KR dam opening; K-DO: Kebir branch of KR dam opening; KW-DO: opening of KW dam; SF-DO: opening of SF dam; KR-DE: exit of KR dam, KW-DE: exit of KW dam; SF-DE: exit of SF dam; KR-M: outlet of KR River; KW-M: outlet of KW River; SF-M: outlet of SF River.
Stations échantillonnées des différentes rivières côtières du Kebir-Rhumel (KR), Kebir ouest (KW) et Safsaf (SF). : barrage; R-DO : entrée de la branche Rhumel au barrage KR ; K-DO : entrée de la branche Kebir au barrage KR ; KW-DO : entrée du barrage KW ; SF-DO : entrée du barrage SF ; KR-DE : sortie du barrage KR, KW-DE : sortie du barrage KW ; SF-DE : sortie du barrage SF ; KR-M : embouchure du KR ; KW-M : embouchure du KW ; SF-M : embouchure du SF.
3 Analytical methods
Flow velocities of the streams, at the moment of water sampling, were assessed with the current meter CM-2 (Toho Dentan Co. Ltd, Tokyo). The flow rate (m3 s−1) was calculated by multiplying the water velocity (m s−1) by the total surface area (m2) of the rivers’ transecting at the sampling stations. Measurements of water velocity were taken at several points (three to 40) depending on the river section size and depth. These measurements allow computing the average current velocity.
All hydrological parameters and nutrients were measured twice a month from March 2010 to February 2011 in three stations for KW and SF Rivers and four stations in KR catchment (Fig. 1). Measurements in the SF River were performed monthly because of some practical constraints. Stations were located at the entrances and exits of dams and at the outlets of the respective rivers. KR River was however sampled from the entrances of the Rhumel and Kebir branches (Fig. 1).
Two liters of water from the middle of the flow were collected for nutrient analysis. Water samples for nutrient analyses were frozen in polyethylene bottles and processed within two days from collection. In the laboratory, after filtration of the sample through Whatman GF/C glass filters (0.5 μm porosity), dissolved inorganic nitrogen DIN (ammonia: NH4; nitrate: NO3; nitrite: NO2), dissolved organic nitrogen (DON), phosphates PO4 and silicates (SiO4) were determined by means of standard colorimetric methods described in Parsons et al. (1989). Total dissolved phosphorus (TDP), polyphosphate (P2O5), and dissolved organic phosphorus DOP were measured following the standard method of Rodier (1996). In this study, only the dissolved inorganic phosphorus (DIP) was considered as the sum of PO4 and P2O5.
The instantaneous fluxes of nutrients were calculated by multiplying their concentrations by the river flow. The annual loads of nutrients were estimated using the method of average instantaneous loads (Preston et al., 1989):
Retention = inflow flux [mainstream + wet atmospheric deposition] – outflow flux from dam storage. The atmospheric deposition to dams was neglected, as it would not exceed 1 to 3 t/yr/dam, for dissolved nitrogen for example (data not shown). Calculation of average loads of nutrients upstream to the Kebir-Rhumel dam was weighted for each tributary (Kebir and Rhumel branches) by their respective water discharge. Nutrient retention in the KR dam represents the mean values of Rhumel and Kebir branches compared to the exit dam ones.
4 Results
4.1 Nutrient levels and retention/production and discharges upstream and downstream the dams
At the entrance of KR dam, the levels of all nutrients were maximal in the wet period and peaked in April and January, as it can be observed in Fig. 2a. Among dissolved nitrogen forms, NH4 fraction dominated at 40% with an average value of about 32 μM in contrast to NO3 that did not exceed 18 μM. The opening of the KR dam was paradoxically highly polluted with NH4 along the year. Fortunately, the dam acts here as a real sink reducing the levels of NH4 by 97% at its exit (Fig. 2b). The bad conditions characterizing the introduced water into the dam were also expressed by the high PO4 levels that were reduced by the dam in the same magnitude of NH4. At the opening of the KR dam, not only SiO4 levels were found low, but have experienced large reductions (29%), being lowered to 55 μM in average. At the exit of the KR dam, inorganic nutrients levels followed remarkably those found at the entrance, but decreased, depending on the element, by 19 to 97% (Fig. 2b). In fact, DIN and DIP levels decreased by 75% and 86% respectively compared to the entrance ones. Paradoxically, the organic dissolved nutrients DON and DOP increased however by half at the outlet of KR dam. The levels of dissolved organic nutrients at dam entrance and exit vary differently globally according to the hydrological season with low values at the dam entrance and high values at the dam exit in dry period. They however follow the same tendency in the wet period (Fig. 2a).
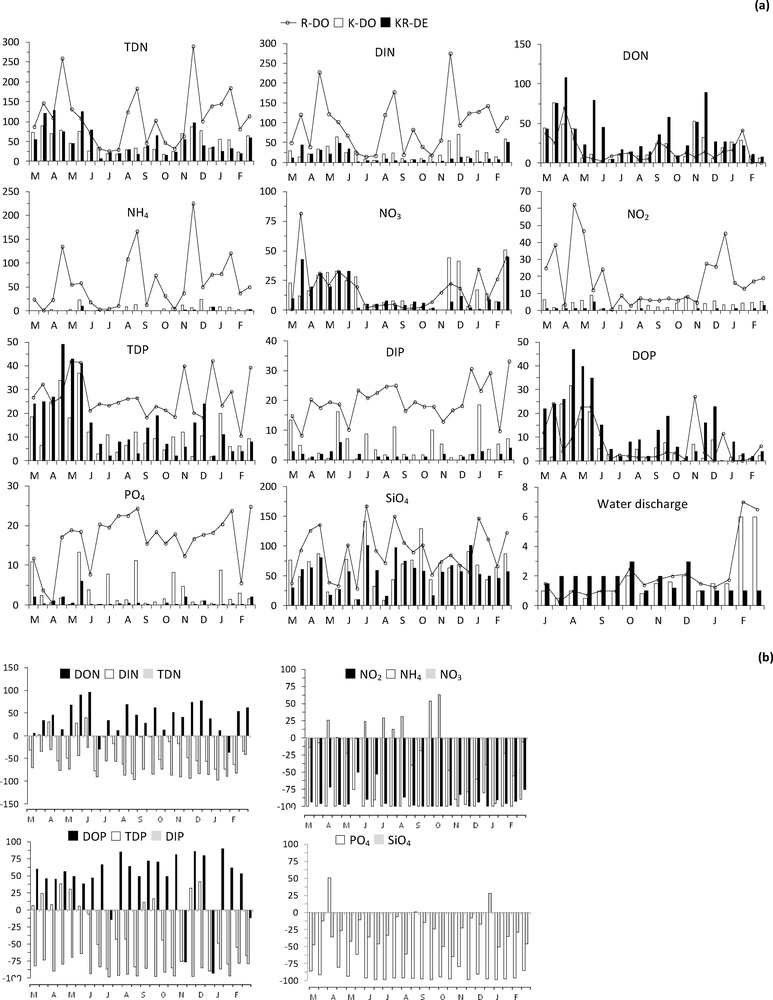
Variations in nutrient levels (μM) and water discharge (m3 s−1) (a) and retention rates (%) at the Kebir-Rhumel (KR) dam (b). Negative values denote retention and positive ones denote release.
Variations des teneurs en nutriments (μM) et des débits d’eau (m3 s−1) (a) et taux de rétention (%) pour le barrage de Kebir-Rhumel (KR) (b). Les valeurs négatives indiquent une rétention et les valeurs positives une production.
The introduced waters into KW dam showed the same seasonal cycle as in the KR catchment, but with low levels in TDN and TDP and higher values of SiO4 (Fig. 3a). If SiO4 levels did not follow any seasonal variation, TDN and TDP levels increased in the wet period, except for the late August sample when local storm had carried large amounts of different inorganic nutrients. At the dam opening, waters of KW showed higher levels in dissolved organic nutrients in spring and in late autumn (Fig. 3a). The fraction of DON was in the order of 25% and reached 50% for DOP. All inorganic nutrients were submitted to large retention in the KW dam, in particular for NH4 and PO4, which were lost at about 70 to 80%, reducing their levels at the exit of the dam to 4 μM and 2 μM, respectively (Fig. 3a, b). At the exit of the KW dam, the levels of SiO4 decreased by half, remaining at only about 50 μM. In contrast to mineral elements, there were large releases of DON (34%) and DOP (24%) in the dam, especially in spring, when production reached over 50% of dissolved nitrogen. Biogeochemical transformations within the KW dam were expressed by strong retention of DIN (48%) and DIP (67%) parallel to the relative large production of DON (34%) and DOP (24%), as it can be seen in Fig. 3b.
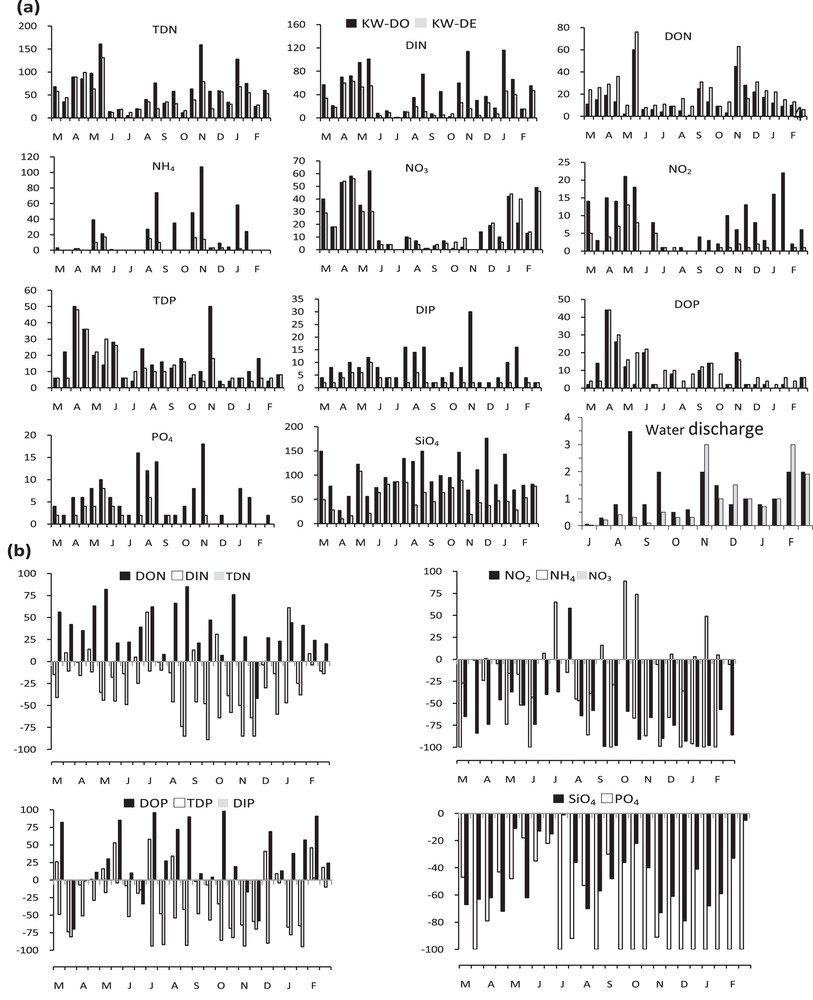
Variations in nutrient levels (μM) and water discharge (m3 s−1) (a) and retention rates (%) at the Kebir western (KW) dam (b). Negative values denote retention and positive ones denote release.
Variations des teneurs en nutriments (μM) et des débits d’eau (m3 s−1) (a) et taux de rétention (%) pour le barrage Kebir Ouest (KW) (b). Les valeurs négatives indiquent une rétention et les valeurs positives une production.
The SF dam receives low levels in TDN varying in the 12–80 μM range, with an average of 30 μM, where DIN and DON were in average equally represented (Fig. 4a). The main characters were the dominance of NO3 at 38% in the wet period and the dominance of DON (51%) in the dry season (relatively to NO3). Waters entering the SF dam were also impoverished in TDP, in particular the PO4 fraction that forms only 15% within the TDP (Fig. 4a). Here, the DOP abounds along the year, with an average level of 4.2 μM (78%). The SF River delivered into the dam high levels of SiO4, exceeding 150 μM in winter and spring. Upstream the dam, the Si:DIN was remarkably elevated, reaching 9, and DIN:PO4 was about 19, indicating the near-pristine water characteristics entering the dam in this period of the year. Downstream the dam, not all biogeochemical parameters were modified as for the other dams, but only PO4 and SiO4 have been reduced to about 30% and NH4 decreased to more than a half (Fig. 4b). Compared to their levels upstream the SF dam, the DON and DOP fractions increased however downstream the dam by 64% and 84%, respectively.
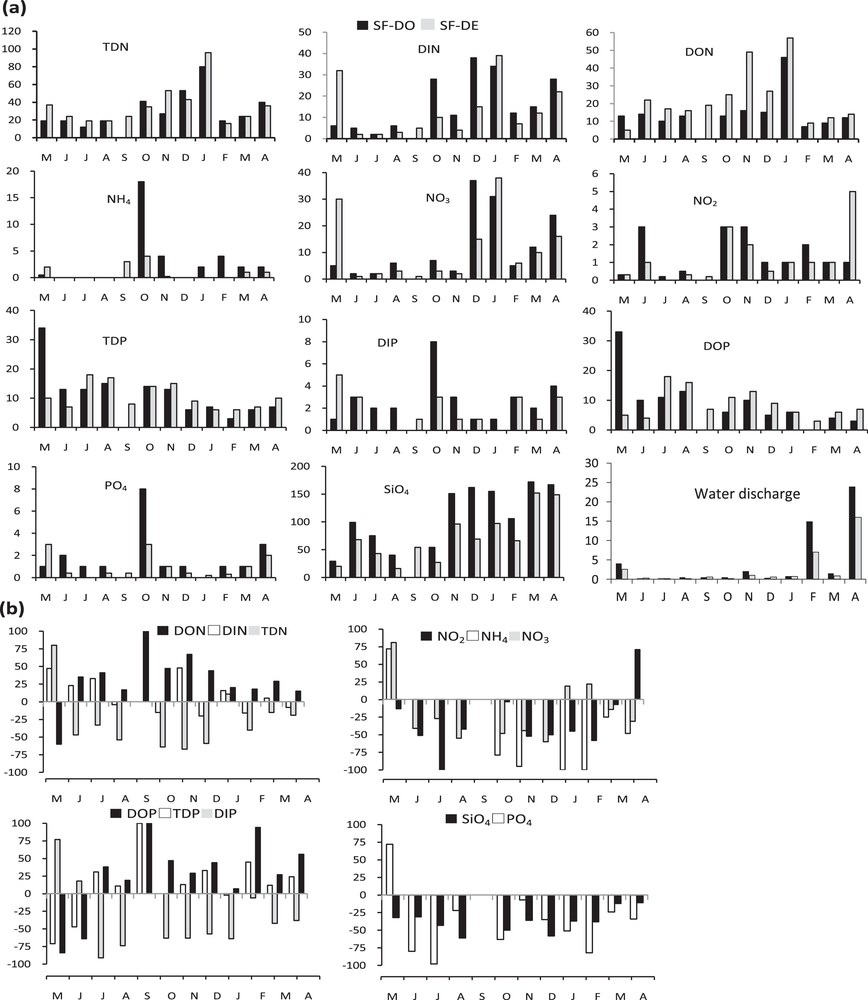
Variations in nutrient levels (μM) and water discharge (m3 s−1) (a) and retention rates (%) at the Safsaf (SF) dam (b). Negative values denote retention and positive ones denote release.
Variations des teneurs en nutriments (μM) et des débits d’eau (m3 s−1) (a) et taux de rétention (%) pour le barrage Safsaf (SF) (b). Les valeurs négatives indiquent une rétention et les valeurs positives indiquent une production.
4.2 Nutrient distribution and discharge at river outlets
As can be seen in Fig. 5a, the maximum levels in the KR River outlet of all dissolved nitrogen were observed in the wet period extending from November to April, when DIN values increased by 28% compared to their average value. However, SiO4 and dissolved phosphorus components did not show any clear tendency. Over the year, SiO4 levels fluctuated in the 10–100 μM range, showing surprisingly no clear relationship with the hydrological cycle and the river flow. The same seasonal cycle may be observed for dissolved phosphorus compounds (Fig. 5a). The TDN reaches in average 55 μM in the KR outlet (Fig. 5a), where DON forms 39% and 32% was in the form of NO3 and 26% in the form of NH4. The major component of TDP was DOP, which represents 78% (12 μM); the PO4 fraction did not exceed 13%. At the outlet of KR River, the molar ratio of TDN:TDP was about 7, NO3:PO4 reached 32 and DIN:PO4 increased in average to 51. In most cases, DIN:PO4 ratios deviated from Redfield values, 50% of cases above 20 and 21% of cases below 4. The SiO4 levels were found low, not exceeding in average 64 μM; here the Si:DIN ratio increased to 3.7 and Si:PO4 was about 54.
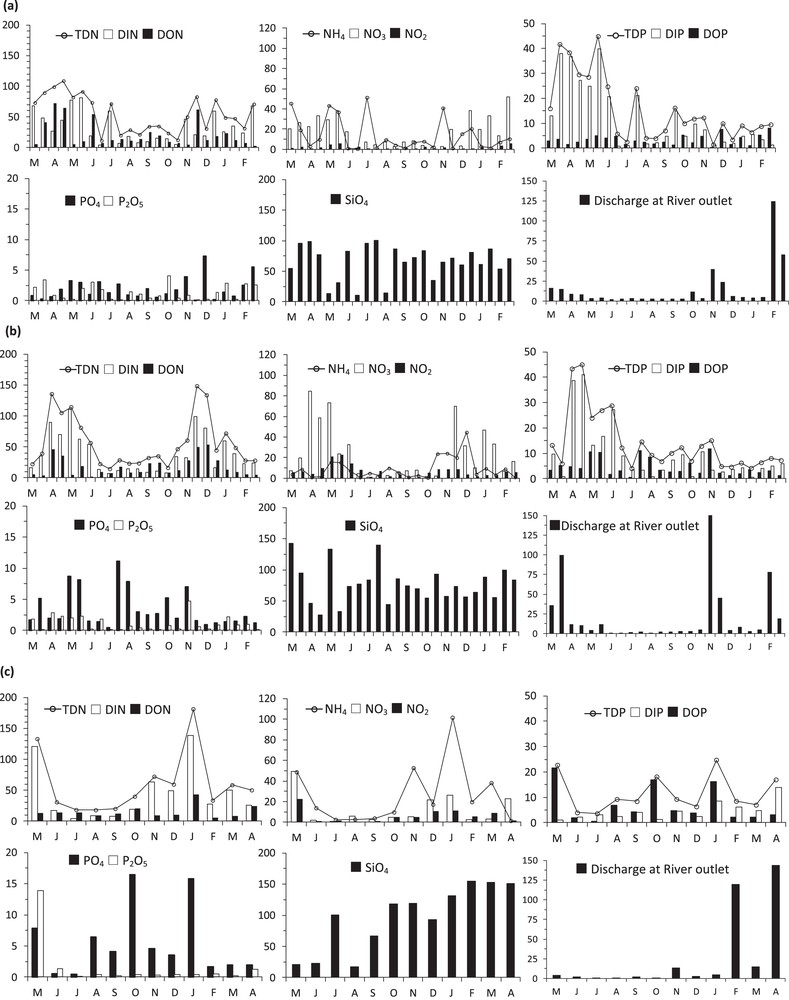
Variations in nutrient levels (μM) during the period between March 2010 and February 2011 in the different river outlets: Kebir-Rhumel (KR) (a); Kebir western (KW) (b) and Safsaf (SF) (c) outlets. The water discharge (m3 s−1) is also given for each river outlet.
Variations des teneurs en nutriments (μM) durant la période entre mars 2010 et février 2011 dans les différentes embouchures des rivières : (KR) : Kebir-Rhumel (KR) (a) ; Kebir ouest (KW) (b) et Safsaf (SF) (c). Les débits d’eau (m3 s−1) à chaque embouchure sont également indiqués.
As shown in Fig. 5b, the dissolved nitrogen forms in KW outlet follow clear seasonal variations reaching high values in wet period and lower levels in dry season (late spring and summer) in contrast to PO4 and SiO4, which did not show any seasonal trend. When compared to annual average values, levels of DIN and NO3 decreased by 60% and NH4 and DON decreased by 30% in the dry season. In the KW outlet, the levels of TDN were found elevated, with an average of 57 μM and oscillated within the 14–148 μM range. The organic fraction forms only 31%, NO3: 40% and NH4: 16%. Within the dissolved phosphorus, the organic form dominated (67%) all the time, with a mean value of 9.4 μM and PO4 represents only 24%. SiO4 levels varied in the 28–143 μM range, with an average value of 77 μM. The delivered waters from the KW outlet were highly disturbed in terms of Redfield ratios as DIN:PO4 and Si:PO4 that were increased respectively to 20 and 41. Even if Si:DIN was in average about 4, it was however below 2 for 42% of samples.
As for the other rivers, the TDN followed clear seasonal variation in the SF River (Fig. 5c) showing large levels in the wet period and low values in the dry one. In the wet season, levels of DIN, NO3 and NH4 increased by 55% in comparison to the annual mean value. The same seasonal cycle may be observed in SiO4 variations where levels in the wet period enhanced by 23%. There was no seasonal apparent tendency in P forms, except the DOP levels which were increased by 20% (Fig. 5c) relative to the annual mean value. In the SF River outlet, the levels of TDN ranged between 18 and 181 μM, with an average of 59.5 μM (Fig. 5c). The DIN represents a majority fraction of 74% and NH4 accounts for 43.5%, forming the double of the NO3 one. The discharged waters from the SF mouth were impoverished in DON; the average level declines to 15 μM there. In terms of TDP, the levels were in average of 12 μM, in which DOP represents 38% and the PO4 represents 46%. The other particularity of the SF outlet waters was the high level of PO4 (5.4 μM) relative to the other rivers. Considering the Redfield N:P ratio, the related ratios were: TDN:TDP = 5.4; NO3:PO4 = 3; DON:DOP = 5 and DIN:PO4 = 13. Since NH4 dominates the dissolved nitrogen components as a particularity of the SF River, the DIN:DIP ratio reached 8.7. The SiO4 levels fluctuated between 17 and 154 μM, with an average of 94 μM. The Si:DIN ratio reached in average 5 and Si:DIP was about 40, indicating the dominance of N relative to SiO4.
4.3 Nutrient fluxes into and from dams and at river outlets
All dams act as filters retaining large masses of dissolved inorganic nutrients, especially the DIN, which was strongly trapped at the rate of 150 to 640 t/yr and the Si–SiO4 that was trapped at the rate of 500 to 1918 t/yr, depending on the dam (Table 1). Even if P–PO4 appears to be trapped in low masses (26–320 t/yr), this loss represents however more than half of the incoming flux. By contrast to the mineral fraction, DON and DOP were produced at different rates according to the dam. The KR and KW dams produced low rates of DON, reaching only 1 and 23 t/yr respectively compared to the SF one, which seems to be weakly productive, presenting paradoxically a negative budget as it can be remarked in Table 1. The negative budget of SF dam may be related to its exceptionally high winter renewing. The production of DOP in all dams may be considered as a general rule; where fluxes delivered downstream, they were in the order of 9–50% or 4–56 t/yr. The loading ratios of Redfield were found profoundly modified (Table 1). The dams regulate the ratios from high and unbalanced values to low and near-balanced ones. In the same way, Si:DIN loading ratios increased to more balanced values (Table 1).
Flux annuel des nutriments à l’amont, à l’aval de chaque barrage et aux embouchures des rivières de bassins respectives.
Flow (m3 s−1) |
NH4 (t/yr) |
NO2 (t/yr) |
NO3 (t/yr) |
DIN (t/yr) |
DON (t/yr) |
TDN (t/yr) |
PO4 (t/yr) |
TDP (t/yr) |
DOP (t/yr) |
DIP (t/yr) |
SiO4 (t/yr) |
N:Pa (g/g) |
Si:Na (g/g) |
Si:Pa (g/g) |
||
KR dam | Entrance | 6.5 | 261 | 150 | 275 | 686 | 153 | 840 | 329 | 554 | 54 | 434 | 2184 | 3 | 4 | 7 |
Exit | 2.7 | 3.63 | 40 | 3.63 | 47.3 | 154 | 201.3 | 8 | 124 | 110 | 14 | 680 | 31 | 23 | 180 | |
R/P (%) | –98 | –73 | –98 | –93 | +1 | –70 | –98 | –78 | +51 | –97 | –67 | |||||
KR River | Outlet | 31.7 | 95 | 19 | 136 | 251 | 102 | 353 | 40 | 180 | 112 | 70 | 901 | 29 | 8 | 75 |
(12) | (17) | (2) | (31) | (13) | (44) | (6) | (22) | (14) | (10) | (111) | ||||||
KW dam | Entrance | 1.44 | 141 | 23 | 53 | 217 | 58 | 275 | 62 | 132 | 46 | 84 | 927 | 12 | 22 | 91 |
Exit | 0.95 | 14 | 3.63 | 53 | 70.6 | 80.5 | 221.7 | 8 | 62 | 50 | 14 | 333 | 53 | 20 | 133 | |
R/P (%) | –90 | –84 | 0 | –67 | +28 | –19 | –88 | –53 | +9 | –83 | –64 | |||||
KW River | Outlet | 24.3 | 162 | 82 | 172 | 416 | 212 | 628 | 110 | 290 | 124 | 166 | 2122 | 20 | 9 | 76 |
(85) | (92) | (45) | (222) | (112) | (331) | (58) | (152) | (65) | (88) | (1117) | ||||||
SF dam | Entrance | 4.1 | 47 | 25 | 214 | 286 | 149 | 435 | 48 | 190 | 81 | 142 | 3861 | 25 | 20 | 146 |
Exit | 2.5 | 7 | 26 | 106 | 139 | 127 | 266 | 22 | 158 | 114 | 45 | 1943 | 29 | 25 | 159 | |
R/P (%) | –85 | +4 | –50 | –51 | –14 | –39 | –54 | –16 | +29 | –68 | –50 | |||||
SF River | Outlet | 26 | 170 | 48 | 152 | 370 | 53 | 423 | 60 | 158 | 86 | 72 | 3368 | 9 | 34 | 63 |
(136) | (122) | (38) | (296) | (42) | (338) | (48) | (172) | (67) | (58) | (2694) |
a Different loading Redfield ratios expressed as N:P: DIN:PO4; Si:N: SiO4:DIN and Si:P: SiO4:PO4.
Nutrient fluxes and specific loadings from the studied rivers are shown in Table 1. The highest values of nutrient fluxes were found at the outlets of KW and SF Rivers. The TDN fluxes varied in the range of 2 folds, from 353 t/yr in KR to 628 t/yr in SF where DIN forms 66–87% in contrast to values expressed in terms of levels. When evaluated in specific fluxes, the largest watershed of KR delivers low masses compared to the little watersheds of SF and KW. The KW River was the major diffusive source in P delivery, similarly to the N inputs. The DOP accounts for more than half and P–PO4 accounts for more than third of TDP. The specific P loading in the relative large watershed of KR was several times lesser than that of the KW and SF. The same tendency was observed in Si–SiO4 delivery, as it can be seen in Table 1. All the Redfield loading ratios have been changed, decreasing in N:P and increasing in Si:N and Si:P. There was excessive amount of P relative to N masses introduced into the adjacent marine environment. At the opposite of inorganic nutrients that largely increased behind dams (by 1.5 to 5 fold depending on the element), there was some perceptible loss of organic nutrient reaching the river outlets of KR and SF. However, as an estuarine part, the outlet of the KW River produces, on the contrary, large amounts of DON and DOP (Table 1).
5 Discussion
It seems that dams act as biogeochemical transformers; they partially convert the received inorganic nutrients into dissolved organic nutrients (DOP and DON) released from plankton metabolism. This is clear in terms of levels but it is less evident for fluxes estimations. Yet, at their entrances, KR and KW were heavily charged in DIN and DIP; they were always higher in the entrance compared to the downstream dams. The SF River, in contrary, penetrates the dam at low concentrations, but was charged in high inorganic nutrient when reaching the outlet. Dam effects on biogeochemical transformations were largely expressed by:
- • retaining dissolved inorganic nutrients;
- • producing dissolved organic matter;
- • modulating the balance of Redfield ratios.
The inorganic nutrients were greatly trapped in dams at 65 to 97% for NH4 and 38 to 93% for PO4 in terms of levels or fluxes. The most important retention rates were found in KR and KW dams, while the SF has the lowest retention rates because perhaps of its little residence time that allows rapid renewing. In terms of loading, the incoming flux of Si–SiO4 was submitted to relative great retention compared to the N and P, varying between 67% in KR dam to 64% in KW dam. In the temperate reservoirs of Marne, Seine, and Aube, Garnier et al. (1999) reported relative low retention of N–NO3 (40%) and more elevated rates for Si (50%) and (60%) for P–PO4. In the other temperate reservoir of Iron Gate I built on the Rhine River, Humborg et al. (2000) demonstrated that over 80% of dissolved Si reduction can be related to the retention by Iron Gate I.
From another point of view and considering the growing concern about the impact of dams on inorganic nutrient cycling (Conley et al., 2000; Humborg et al., 2000; Thieu et al., 2009), surprisingly no attention has been given to dissolved organic matter released from dams. Despite the ecological importance of DON in aquatic systems (Mortazavi et al., 2001; Seitzinger and Sanders, 1997; Wiegner et al., 2006), information on the spatial and temporal distribution of DON in dams is lacking. The results on hand show clearly large releases of DON and DOP in terms of levels at dam exits that should be considered in nutrient budgeting over the catchment system. The release of dissolved organic matter (DOM) in terms of flux is not more evident because of the shifting between nutrient levels and water discharges upstream and downstream the dams. Globally, the high DOM values coincide with low discharges and, on the contrary, the low values rise with high water inputs. Moreover, the high residence time of dam water (2.4 yr in KW dam and 3.6 yr in KR dam) encourages the development of plankton organisms that maintain the release of DOM. When the water renewing is fast, as in the SF dam (12 times a year or 0.07 year), the DOM was retained. Therefore, a fraction of the retained inorganic nutrients would have served to induce releases on DON and DOP in the KR and KW dams. However, removing of nitrogen is not often observed within reservoirs (Frield and Wüest, 2002; Taleb et al., 2004). Besides the elimination in water bodies of DIN by denitrification (Seitzinger et al., 2006) and the removal of phosphorus by storage in sediments (Straskraba et al., 2007), these inorganic nutrients would partially be converted into dissolved organic matter from plankton metabolism and then released to the water layer. This transformation may be specific to warm environments, as the Mediterranean dams where the warm waters allow maintaining the plankton productivity along the extended dry period. Indeed, this study confirms that a part of DON is bioavailable, but that bioavailability depends on its origin. Notably, DON from urban waste is mostly bioavailable, DON from agricultural sources is also bioavailable but with a lower bioavailability rate and natural DON remains refractory (Petrone et al., 2009).
It is important, on the other hand, to point out the high DOP values found in this study compared to values recorded in continental waters that usually fluctuate around 0.5 μM. However, Prasad and Ramanathan (2008) recorded comparable values reaching in average 6 μM in a mangrove estuarine system under post-monsoon and summer conditions.
The other negative role of dams would be the modification of the loading Redfield ratios, changing from relative balanced values to high and altered ones. The delivered waters from the dam were highly disturbed in terms of Redfield ratio. The loading ratios of DIN:PO4 change from 9 to 19 upstream to 31 to 95 downstream respectively. Furthermore, the loading ratios Si:DIN increase in dam outflows to elevated values (> 8), indicating the large retention of N relative to Si. Downstream the dam, at the lower catchment part, the increase of DIN and DIP may be mostly related to intensive agricultural activities spreading in this area of the SF River. Despite the large inorganic nutrient losses in dams, there were additional inputs behind dams; the DIN rate delivery was important, varying from 38% in the KW to 70% in the SF and rate of DIP releases increased by 50–75% (Table 1). In contrast to N and P, derived mostly from anthropogenic inputs, SiO4 originated from rock weathering and entered streams downstream the dams, increased only in the range of 15–30% compared to their levels at dam exits. On the other hand, the release of dissolved organic matter in dams was in large part consumed downstream along with their progress to coastal waters. This in-stream elimination may be explained by bacterial consummation as reported by numerous works (Bustillo et al., 2011; Purvina et al., 2010; Wiegner et al., 2006; Wolfe et al., 1999).
In terms of specific fluxes, the small catchments of KW and SF deliver to the sea large masses of P–PO4 (48 to 58 kg/km2/yr) in comparison to the large catchment of KR that comprises the biggest dam of Algeria. These amounts may be considered among the highest one in Mediterranean rivers (eg., Ludwig et al., 2009). Again, because of the large retention in dams, the specific fluxes of DIN were low in all catchments (30 to 300 kg/km2/yr) in comparison to the Mediterranean rivers. The large amount of the DON that was introduced to coastal water (up to 212 t/yr) suggests that this fraction may contribute noticeably to marine eutrophication, as already demonstrated by Seitzinger and Sanders (1997). In the same way, the fluxes introduced to the coastal zone showed that DIN:PO4 and Si:PO4 ratios were often superior to the Redfield ratios values, suggesting that P may be the limiting factor for phytoplankton growth. Also, the Si:DIN ratios were in more than 30% of the cases below the diatoms requirements (Redfield et al., 1963), implying that N was delivered in excess over silica (Si:N < 1). Worldwide, the alteration of Si:N ratios become problematic, as reported in numerous works (Bernard et al., 2010; Ragueneau et al., 2006). In fact, silica is known to play a major role in earth systems by sustaining diatom productivity and by the elimination of atmospheric carbon dioxide (Conley, 2002). Also, the decrease in Si:N can result in Si limitation and may lead to drastic modifications of phytoplankton composition and marine ecosystems productivity (Turner et al., 1998).
In conclusion, it can be retained that the major characteristics of the studied rivers are the high values of NH4 and PO4, unlike SiO4 levels that were remarkably lowered because of its retention in dams. All inorganic nutrients experienced large removal in dams, constantly exceeding 50% relatively to the incoming flux. The inorganic N and P were more trapped (up to 98% for N and up to 93% for P). The intercepted runoff in dams has largely reduced the river flow, where about 30–60% of the incoming water was stored annually in these artificial impoundments. The other particularity is that the dams were heavily polluted by direct wastewater inputs from the neighboring big cities and several villages. Surprisingly, the KR and KW dams’ entrances, supposed to be protected, were heavily polluted by strong inputs of NH4 and PO4. Furthermore, the delivered waters from the dams were highly charged in dissolved organic matter, which let us assume that these artificial impoundments would play a crucial role in nutrient biogeochemical cycling. These hydrological and biogeochemical conditions would have severe impacts on the river systems and on the functioning and the productivity of the receiving coasts.
Acknowledgments
This study was supported by the National Research Program 4 (contract No 4U/23/253). We feel really indebted to the anonymous reviewers who have spent a lot of time to peel and vivify our paper. We are indebted to Dr C. Abdenour and Dr M. Khélifi-Touami from the Annaba University Badji Mokhtar, who have carefully polished up our English writing.