1 Introduction
The Dead Sea Fault is the boundary between the Arabian plate and the Mediterranean plate, being a left-lateral strike-slip fault since the Neogene. Its displacement is several millimetres annually, enabling M > 7 earthquakes. A relatively moderate seismicity in Syria indicates that displacement occurs through major, but rare earthquakes. The millennium-long historical record of seismic events (Ambraseys, 2009; Guidoboni and Comastri, 2005; Sbeinati et al., 2005) has been lengthened by extensive archaeoseismological studies, mostly in Israel (e.g., Amit et al., 2009; Ellenblum et al., 1998; Karcz and Kafri, 1978; Karcz et al., 1977; Korjenkov and Erickson-Gini, 2003; Korjenkov and Mazor, 1999a,b, 2003, 2005, 2013; Marco, 2008; Marco et al., 1997, 2003; Wechsler et al., 2009; Yagoda-Biran and Hatzor, 2010), and less in the West Bank (Alfonsi et al., 2013; Karcz and Kafri, 1981), in Jordan (Al-Tarazi and Korjenkov, 2007; Haynes et al., 2006; Niemi, 2009), in Lebanon (Daëron et al., 2005; Elias et al., 2007), and in Turkey (Altunel et al., 2009; Benjelloun et al., 2015). The number of studies along the 300-km-long Syrian sector is still meagre (Karakhanian et al., 2008; Kázmér and Major, 2010; Meghraoui et al., 2003; Sbeinati et al., 2010).
During the spring fieldwork season of 2010, we visited the town of Sāfitā, and the abandoned Late Antiquity village sites of Khirbat al-Qurshiyya, and ‘Ayn-Qadīb, in the Jabal Ansāriyya. We identified and surveyed various damages and failures visible on buildings, and also detected rockfalls. Some of those attributable to earthquakes are described, illustrated, and explained here. Surveying instruments included a Leica Disto D8 laser distance metre, compass, and clinometre. We provide new data on these localities, which preserved evidence of earthquake damage and directionality of strong motion.
1.1 Archaeoseismology
The instrumental record of seismic activity is less than hundred years old, while the recurrence of major earthquakes is measured on a centennial-to-millennial scale. Archaeoseismology aims at extending this record throughout the period of man-made structures. Ancient buildings bearing particular features of damages provide evidence of past earthquakes. Prime examples are the distance and time of repeated displacement of constructions across faults (on-fault studies) (Altunel et al., 2009; Ellenblum et al., 1998; Marco et al., 1997; Meghraoui et al., 2003; Passchier et al., 2013; Sbeinati et al., 2010), study of off-fault damages for the identification and assessment of displacement along the causative fault (e.g., Monaco and Tortorici, 2004), directionality of liquefaction-induced tilting in pagodas (e.g., Kázmér et al., 2011), and directionality of strong motion (e.g., Kázmér and Major, 2010; Korjenkov and Mazor, 2013). A comprehensive system of earthquake archaeological effects is available (Rodríguez-Pascua et al., 2011), allowing rapid correlation of observed damages with macroseismic scales (Rodríguez-Pascua et al., 2013).
2 Results
2.1 Sāfītā
The tower (donjon, keep), built of whitish Cretaceous limestone (Arabic: Burj Sāfītā, Sāfīthā, French Chastel Blanc, Latin Castrum Album), is today in the centre and highest point of Sāfītā town (34° 49′ 14.7″ N, 36° 07′ 02″ E, 374 m a.s.l.). It stands on compact Cretaceous limestone beds. Sāfītā is on the southern flanks of the Jabal Ansāriyya Range, halfway between Tartūs and the well-known castle of the Crac des Chevaliers. It overlooks the vital lowland, the Gap of Homs, which connects the interior part of Syria to the Mediterranean coast (Major, 1998: 221–222) (Fig. 1).
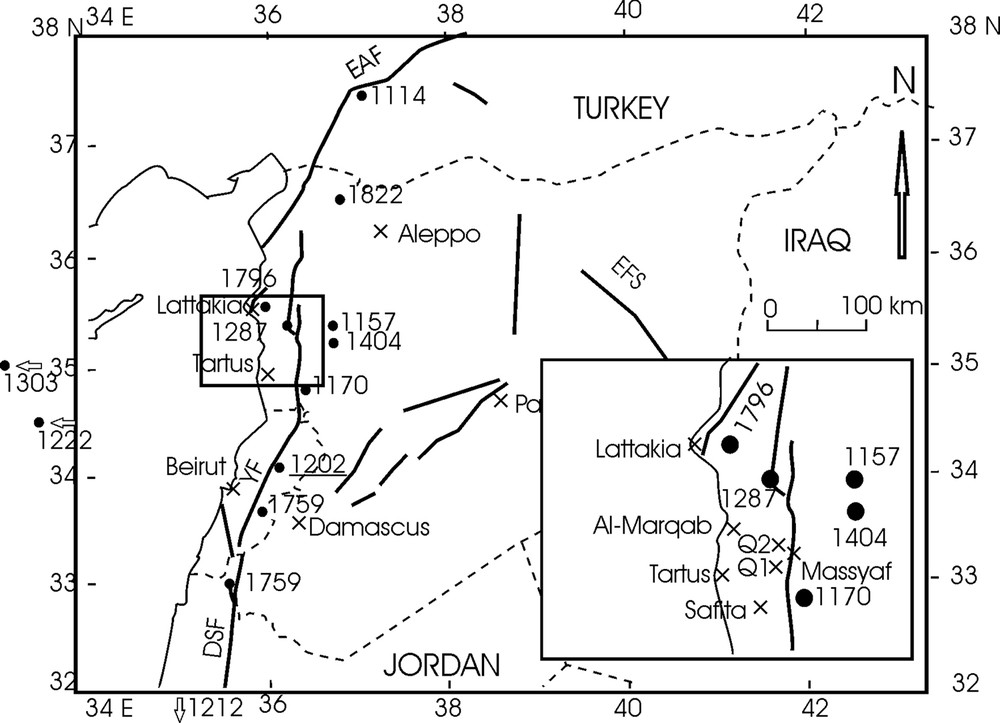
Location of studied sites in coastal Syria. Major historical earthquakes are centred along left-lateral strike-slip faults ranging from the Dead Sea Fault in the south to the East Anatolian Fault in the north (after Sbeinati et al., 2005, modified). The epicentre of the 1202 earthquake is underlined. The epicentres of the events of 1212, 1222, and 1303 are out of the map in Jordan, Cyprus, and Crete, respectively. Q1: Khirbat al-Qurshiya; Q2: ‘Ayn-Qadīb; DSF: Dead Sea Fault system; YF: Yammouneh Fault; EAF: East Anatolian Fault system; EFS: Euphrates Fault system. Epicentre locations are from Ambraseys (2009, electronic supplement).
The central part of the castle is comprised of the enormous rectangular donjon and the scanty remains of an inner enclosure. This is encircled by a huge outer enclosure with flanking towers and the remains of a great hall on two levels. The donjon covering an area of 31.2 × 18 m and being 28 m in height is an example of a so-called église-donjon, a tower with a significant part of it occupied by a chapel (Kennedy, 1994: 138–141) (Fig. 2a). The chapel is situated on the ground floor. One enters the western gateway straight into the church. The barrel vault of the nave is divided into three sections by two arches that rise from pilasters in the side walls. The apse at the eastern end is flanked by two sacristies built into the massive walls on both sides. There are only five windows (really firing slits) in the 3-m-thick masonry (Fig. 2c).
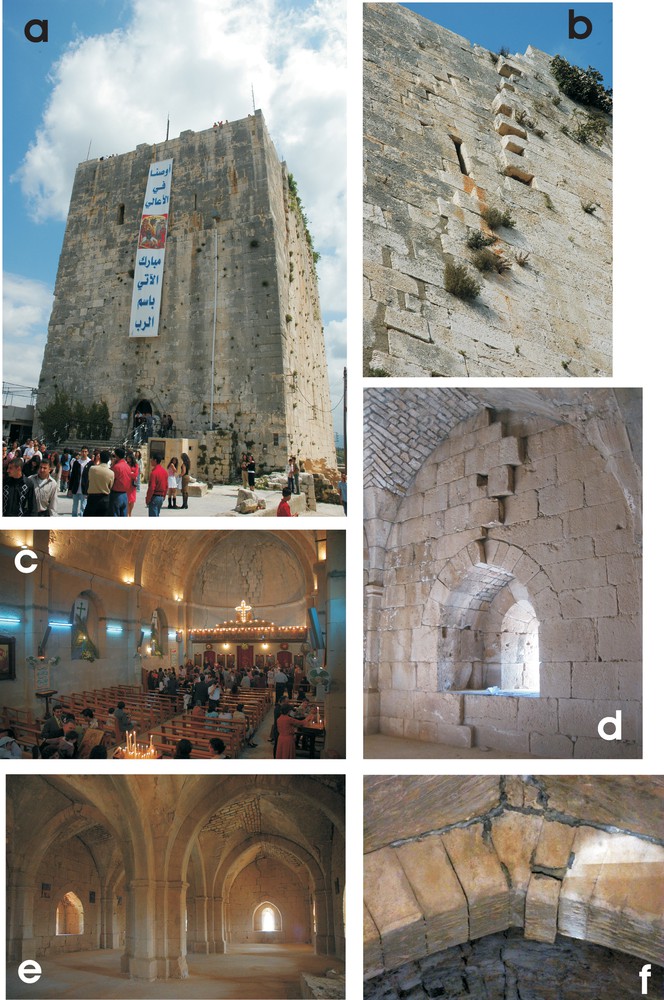
(Color online). The crusader donjon of Sāfitā: a: view from west. Note the window-less walls. The only entrance to the tower is a small door on the western wall; b: detail of the southern façade. Right-lateral displacement of ashlars near the top of the donjon diminishes downwards; c: the ground floor hall is a church, now in use by the local Maronite Christian community. Note the shifted ashlars seen above the illuminated cross in the axis of the hemispherical dome of the apse; d: first floor: left-lateral displacement of the northern wall; e: the first floor hall (above the ground floor church) was probably the common dormitory of the Knights Hospitaller. Two central pillars bear Gothic arches hold the weight of the roof. Heavy repairs in the vault of the right-side aisle are witnesses to shifted and/or broken ashlars there; f: dropped keystone in a north–south oriented Gothic arch of the first floor hall.
The staircase built into the thickness of the southern walls brings the visitor to the upper floor. The hall on this floor has three central cruciform pillars, which divide the room into two parts, each with four bays comprised of cross-groined arches (Fig. 2e). From each bay, a loophole with an arrow slit looks over the countryside. Another staircase leads to the terrace, which is a flat roof. The parapet consists of alternating loopholes and crenulations. Also intended as a last point of refuge, the donjon had its own rock cut cistern beneath. The chapel in the donjon still serves as the main Greek Orthodox church of the town, dedicated to Saint Michael.
2.1.1 History
Sāfītā boasts with the highest surviving donjon of the Holy Land. Making its first appearance in the written sources of the 12th century, nothing is known about its early history. However, the first certain mention of the site was in 1112, when the area of the Gap of Homs, conquered by the Crusaders around 1109, was handed over by Tancred, lord of Antioch, to Pons, the count of Tripoli (Ibn al-Qalānisī, Dzayl, 163; trans. 89). We do not have any certain data on the origins of the fortifications in Sāfītā, but whatever survived above ground clearly dates from the Crusader period on stylistic bases. The castle was one of the earliest possessions of the Order of the Temple in the Syrian coast, who might have acquired it as early as 1152, but in any case, it was certainly in Templar possession by 1155 (Piana, 2008: 295). Lying on the strategic route between the coast and the Syrian interior, the site had a rather troubled history under Crusader domination. In H. 562 (1166/67), it was sacked by the army of Sultan Nūr al-Dīn (Ibn al-Athīr, al-Kāmil, IX/330) and was captured and destroyed by the same ruler again in H. 567 (1171/72) (Ibn al-Athīr, al-Ta’rīkh al-Bāhir, 154) (Raphael, 2010). The great northern campaign of Sultan Saladin in 1188 and his brief raids against Sāfītā are unlikely to have had much effect on the fortress (Ibn al-Athīr, al-Kāmil, X/48), but it withstood a serious assault in 1218, when the army of al-Malik al-Ashraf took and destroyed its suburb (Ibn Wāsil, Mufarrij, III/265). According to a Latin source, some of its defensive towers were also damaged (Oliver Scholasticus, Historia Damiatina, xxxvi, 235). Sāfītā was finally taken by the army of Sultan Baybars on 20 February 1271 after a brief siege (Ibn al-Furāt, Ta’rīkh, 180–182; trans. 143–144).
The donjon is not only marked by its huge size, but also by enormous cracks on its façades, which later reconstructions were unable to conceal. In all probability, this was the “turris maior” [great tower] mentioned in the letter of the Master of the Temple, Philippe de Plessis, describing the effects the AD 1202 earthquake had on Sāfītā (Castrum Album) with the following words: “Castri autem Albi maxima pars murorum cecidit, turris autem maior, qua nullam credimus fortuis vel firmius edificatam, in hoc rimis et quassaturis debilitata est, quod melius nobis esset, si funditus corueret, quam ita stans permaneret” (Mayer, 1972, p. 309). In English: “At Chastel Blanc [Sāfītā], most of the walls collapsed, and the main tower, which we thought to have been built with outstanding strength and solidity, was so badly cracked and damaged that it would have been better for us if it had completely collapsed instead of being left standing in such a state” (translation from Guidoboni and Comastri, 2005, p. 224). The reparation of the effects of the grave destruction is still visible today; at the same time, it shows that the medieval architects working for the Templars did find a solution to the problem of the great tower. In any case it was described by Wilbrand of Oldenbourg in 1212 as “…quid est bonum et forte” [which is good and strong] (Wilbrand of Oldenbourg, Itinerarium Terrae Sanctae, 210). As the visible reparations of the earthquake damage have been executed in a Crusader style (including the gothic hall and a window for a church bell) and there is no serious earthquake mentioned in the years until 1271, it is logical to assume that this earthquake trace was caused by the great earthquake of 1202.
Following the Muslim occupation in 1271, the donjon lost its strategic importance and fell into disuse as a military installation.
Photographs preserved in the Deschamps archives (Deschamps, 1973) (folders XVI and XXI) of the Bibliothèque nationale (Paris) show missing blocks of masonry at random places of the four main walls and around the top floor. Most of these damages cannot be seen today due to the restoration of 1936, during the French Mandate. Perfect fit of masonry at the sites of strike-slip displacement (Fig. 2b, d) shows professional restoration by expert stonemasons. While missing and broken blocks were obviously replaced, there was no attempt to conceal the large-scale shift perpendicular to the plane of the wall. Lower-quality restoration of missing mortar between blocks in arches indicates a subsequent, hastily performed restoration, possibly by local builders (Fig. 2d, f).
2.1.2 Masonry and failures
The donjon of Sāfitā was built of stone masonry and opus caementitium, i.e., Roman concrete (Lamprecht, 2001). The four main walls were built of a single layer of well-dressed, rectangular stones of standard size, both on the external and the internal surfaces. No metal anchors have been used to connect the ashlars. These walls served as moulds for casting the core of the wall, several metres thick, made of layers of rubble and mortar (Ferretti and Bažant, 2006; Mistler et al., 2006). It is very similar to modern concrete in appearance and resistance to weathering and stresses. Masonry both served aesthetic demands and provided a hard, protective layer to counter weather effects and enemy attacks. This layer often served as framework during concrete pouring only, having no supporting function when concrete hardened.
In the following, we describe the major damage types observed on Sāfītā donjon, which are assigned to seismic activity.
2.1.2.1 Extruded walls
The east–west-oriented elongated rectangle of the donjon bore major damages on all four sides. Many of them have been restored since the 1930s, when the Armée du Levant of the French Mandate forces made most important documentary photographs of the building. However, there are four major dislocation features remaining, arranged symmetrically on the northern and southern façades.
Both the northern and the southern façades are symmetrically crosscut—in an approximately vertical direction—by two fractures each, extending from the top downwards through the upper two-thirds of the building (Fig. 2b). The displacement along the fractures is increasing upward. The fractures crosscut the 2.5-m-thick walls, displaying the shifted portions both outside and inside. The amount and sense of displacement along each fracture is identical both on the external and internal surfaces (Fig. 2d). Conspicuously, fractures displacing the northern and the southern walls are of opposite senses (Fig. 3). The net result is that the central portion of the donjon, bounded by the two pairs of fracture zones, is at least 0.5 m wider—in a north–south direction—than the eastern and western portions (Fig. 3). This feature is analogous to the folded (and collapsed) walls, as described by Rodríguez-Pascua et al. (2011).
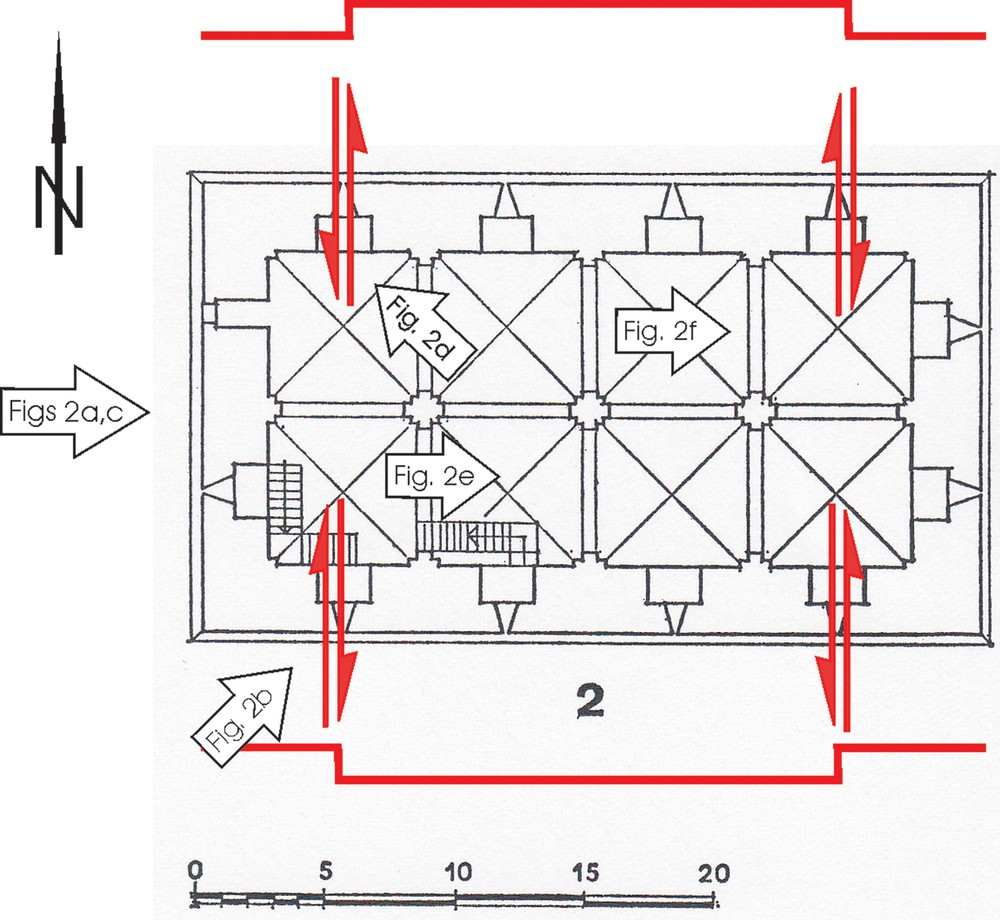
(Color online). Deformation of the donjon. There are four strike-slip shear zones dissecting the donjon across both the western and eastern window bays of the knights’ hall. Heavy red lines: Kázmér and Major (2014): Sāfitā castle 35 illustrate that the central portion of the northern wall moved about 20 cm to the north, while the central portion of the southern wall moved ca. 30 cm to the south. The resulting deformation is a north–south extension of the upper floor of the donjon. Scale in metres. Arrows refer to the photos in Fig. 2.
2.1.2.2 Dropped keystones
The highest points of the arches separating the groin vaults in the upper floor hall are invariably badly damaged (Fig. 2e). Keystones and adjacent ashlars have been broken and/or subsided by several centimetres (Fig. 2f). Finite-element modelling proved that only major earthquakes are capable of producing downward sliding of keystones (Kamai and Hatzor, 2008).
2.1.2.3 Shifted blocks
There are wide gaps, up to a few centimetres, between blocks, distributed seemingly at random. These are the ‘displaced masonry blocks’ of Rodríguez-Pascua et al. (2011).
2.2 Rockfall sites (Khirbat al-Qurshiya and ‘Ayn-Qadīb)
2.2.1 History
Field surveys of the Syro-Hungarian Archaeological Mission in the past years detected a large number of village sites of Romano-Byzantine origin in the deepest recesses of the coastal mountain ranges (Major, 2006, pp. 44–46). The observations of field surveying and sherding make it clear that these settlements were established in the Roman and Byzantine periods, perhaps not much later than the 4th century and the presence of characteristic 13th century pottery found on a much smaller area than the Roman period pottery scatter extension indicates that many of them must have survived the early Middle Ages, but on a reduced scale. The cluster of “rural villas” identified in ‘Ayn-Qadīb makes it a good example of an average middle-sized settlement. Khirbat al-Qurshiyya belonged to the large villages with widely dispersed villas on an extensive area, possessing several olive and wine presses and also having a clearly identifiable church building. Medieval pottery scatter is concentrated only in a limited zone of the site, not surprisingly close to the main water source.
Quarrying left markedly visible traces in Khirbat al-Qurshiyya. Blocks several metres long and wide, and tens of tons in weight, were cut from the horizontally bedded, compact Cretaceous limestone. This stone is suitable for monumental architecture, and the village was certainly a well-to-do community.
2.2.2 Failures
2.2.2.1 Fallen blocks in quarry
The quarries producing rectangular blocks several metres in length were clearly identified at the eastern end of the village and all along its southern periphery. The rock quarried is compact, homogeneous Cretaceous limestone, exposed in thick beds (1.4–2.6 m), separated by thin, lamellar limestone strata and by rare argillaceous intercalations a few centimetres thick. Stratification is nearly horizontal (there is a mere 3° dip to the north). Quarry walls are vertical, following the orientation of joints. These fractures are now very wide (up to several decimetres) caused by karstic dissolution. Blocks as thick as the beds were quarried, separated from the underlying layer along the bedding plane by wedges.
The largest block we found is 7 × 3 × 2 m, 2 m being the bedding thickness. It is more than 100 metric tons in weight. There are several blocks in the quarry yard, (dis)arranged in a spectacular array. There are blocks parallel to the bedding plane, blocks tilted (Fig. 4a, b), blocks with original bedding in vertical position, and overturned blocks. There are blocks supported by other blocks below them, forming a kind of bridge with two supports. Some blocks, being on top of the main quarry wall, extend into the air by more than a metre above the quarry yard.
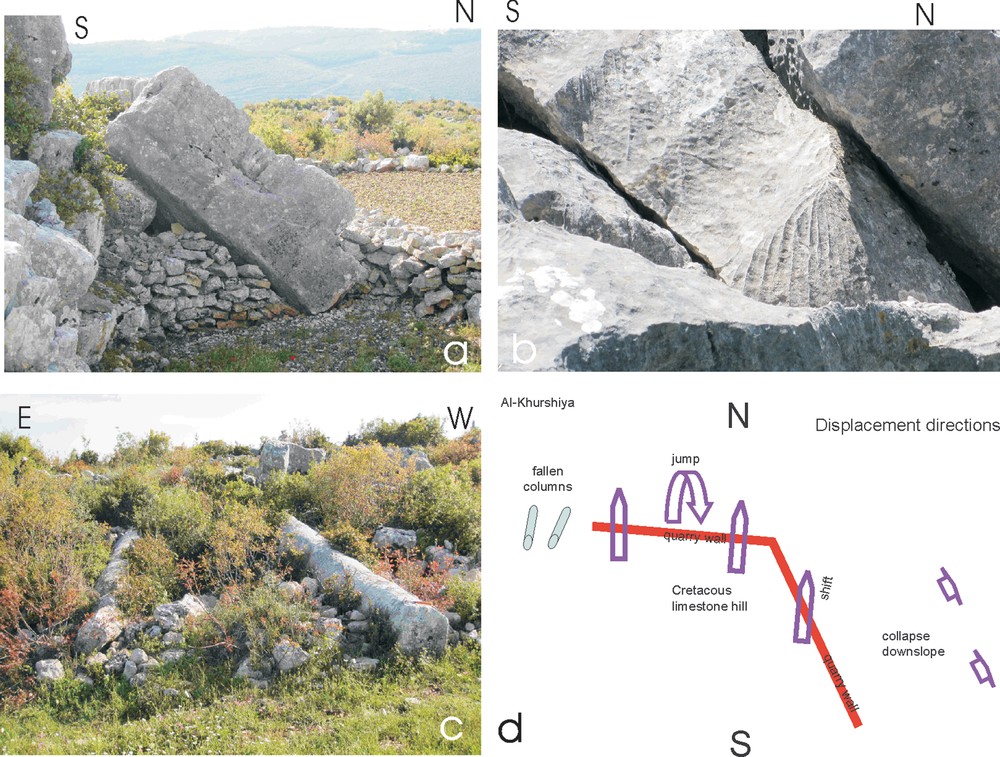
(Color online). Damages and directivity of strong motion in Khirbat al-Qurshiya: a: fallen >20 t block in front of the quarry face. Bedding thickness: ca. 1.4 m. Rubble walls are shepherds’ shelters; b: Karstic dissolution (karren) on fallen blocks. The 20–30-cm-wide furrows (tilted) were originally vertical karstic shafts produced under soil cover formed throughout many millennia. The 1–3-cm wide runnels (vertical) were formed after the rockfall by rainfall dissolution; c: two fallen columns of the village temple lying approximately in a north–south direction (north is towards the viewer); d: sketch of displaced objects: approximately north–south lying fallen columns in the village. Twenty-ton blocks—some overturned—in the quarry, displaced to the north. Blocks slid downhill on the eastern slope.
Karstified joints of cylindrical shape, originally vertical, 20–30 cm width, now tilted or horizontal, clearly indicate the original position of the blocks. Karstic dissolution did not stop after the rockfall: new runnels have been dissolved into the surfaces: these are vertical following the flow direction of rainwater (Fig. 4b). Runnels are 2–3 cm wide, 3–9 mm deep (Table 1).
Karren dimensions on fallen blocks in Khirbat al-Qurshiya. The age is based on a 18-μm/year average dissolution rate (after Furlani et al., 2009), and has to be understood as a rude approximation only.
Width (mm) | Depth (mm) | Age of rockfall | |
Based on an 8-μm/year average dissolution rate | Based on a 22-μm/year average dissolution rate | ||
28 | 8 | 1000 | 364 |
20 | 9 | 1125 | 409 |
28 | 7 | 875 | 318 |
10 | 5 | 625 | 227 |
19 | 6 | 750 | 273 |
25 | 6 | 750 | 273 |
20 | 6 | 750 | 273 |
29 | 3 | 375 | 136 |
19 | 4 | 500 | 182 |
37 | 7 | 875 | 318 |
Displacement directions were identified by fitting the outline of displaced and the underlying blocks: these unequivocally directed towards the north, with an estimated error of ±20°.
2.2.2.2 Fallen columns
The village buildings, including probably the largest building, the church, are all heavily damaged. No wall was left standing higher than 2 m, and most of them are just a low heap of dressed stones at grass level.
Two monolithic limestone columns, 74 cm in diameter, probably carved from local limestone, lie almost parallel to each other at the western end of the village. The western one fell towards 10°N, while the eastern one towards 24°N.
2.2.3 Rockfall in ‘Ayn-Qadīb
‘Ayn-Qadīb, a ‘dead city’ site near the ridge of the Jabal Ansāriyya, suffered from a rockfall. The largest block moved is 5.9 m long, 3.8 m wide and 2.6 m thick, its approximate weight is more than 130 metric tons (Fig. 5). It is overturned, lying on top of the masonry walls of a house. The original site of the block is easily identified: the direction of the fall is 10°N. Nearby north-facing slopes bear debris of extensive rockfalls (Fig. 6).
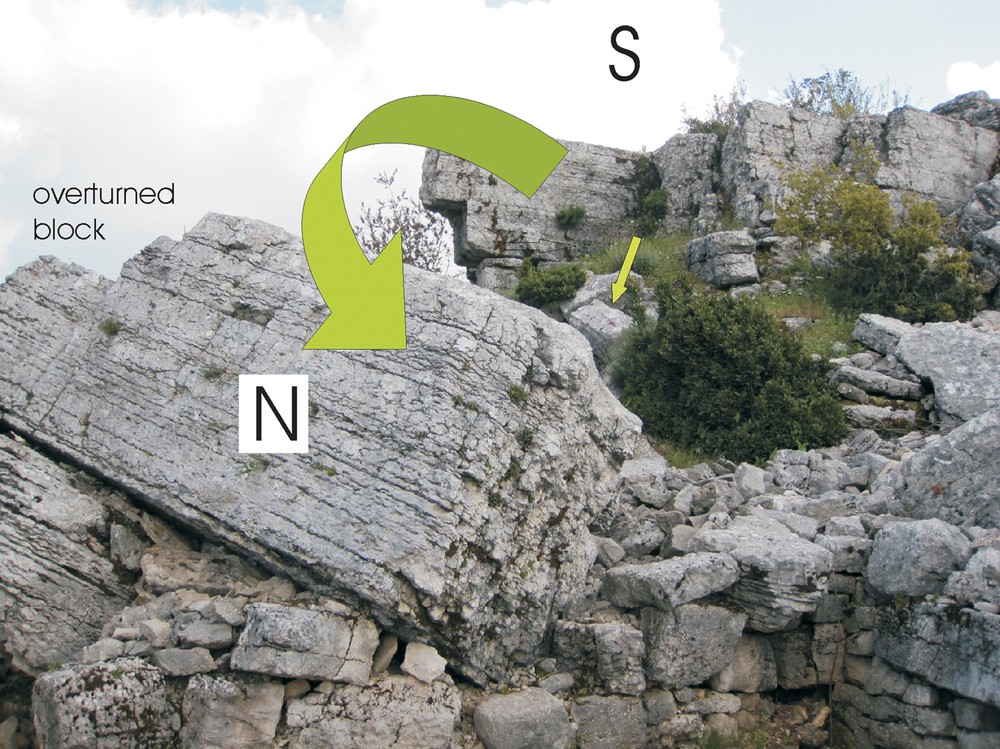
(Color online). Rockfall in ‘Ayn-Qadīb village destroyed a masonry house. The largest block (2.6 m thick, ∼50 t) fell to 10°N and overturned.
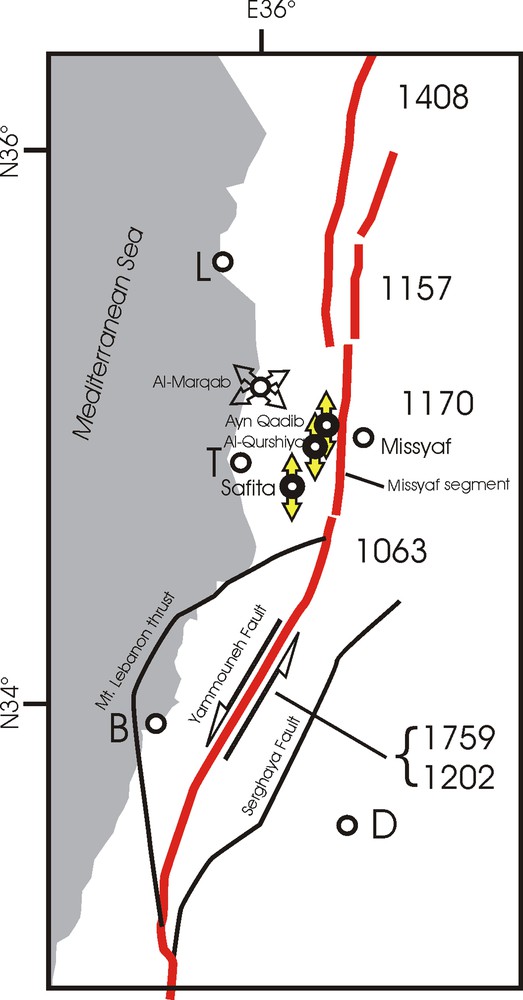
(Color online). Direction of deformation and displacement. Segments of the Levant Fault system after Meghraoui et al. (2003) and Daëron et al. (2007). Paired arrows indicate strong motion directions: north–south deformation in Sāfītā, and northwards displacement in al-Qurshiyya and ‘Ayn-Qadīb. Sāfītā is 19 km from the Levant Fault. Khirbat al-Qurshiyya and ‘Ayn-Qadīb are both less than 6 km from the Missyaf segment. Al-Marqab citadel bears damages oriented towards 240̊ caused by the AD 1202 earthquake and towards 130̊ caused by an earthquake after 1275 (Kázmér and Major, 2010). B: Beirut; D: Damascus; L: Lattakia; T: Tartūs.
3 Discussion
Archaeoseismology traditionally seeks answers to the questions: was there an earthquake and when (Ambraseys, 2005)? Here we seek information on an additional parameter of the earthquake: intensity, and put forward considerations about a potential causative fault.
3.1 Mechanism of damages
3.1.1 The donjon
Sāfitā donjon, having walls up to 4 m thick, is a robust structure. Its height/thickness ratio is h/t = 4 for the lower level and h/t = 5 for the upper level, indicating an extremely strong and earthquake-resistant construction (Lourenço et al., 2007). Several masonry blocks suffered minor displacements in each donjon wall; these belong to the earthquake archaeological effects listed by Rodríguez-Pascua et al. (2011). The dropped keystones in the arches are probably the best evidence of earthquake damage (Kamai and Hatzor, 2008; Marco, 2008).
The gently folded walls on the northern and southern sides of the donjon bend outwards: the 20–30-cm displacement at the top disappears downwards. Recorded by, e.g., Rodríguez-Pascua et al. (2011, their fig. 6c) and by Kázmér (2014, his fig. 2b), this feature is produced by a tall building swinging under seismic vibration.
We noted that the donjon stands on solid Cretaceous limestone beds, i.e. site amplification by soft subsoil can be excluded.
3.1.2 Rockfalls
These are special rockfalls at both sites: the hillslope is vertical, while the sliding plane is horizontal (3°N). Additionally, the sliding blocks are wider and longer than high, i.e. they are extremely stable (Figs. 4a, b and 5). Under normal conditions, affected by gravity only, these blocks do not dislocate. Under seismic excitation (while repeatedly pushed upwards and sidewards by the incoming seismic waves), these blocks can ‘walk’ along the bedding plane, finally falling to the foot of the vertical quarry face. The direction of ‘walk’ is affected by strong motion.
3.2 Dating
3.2.1 Historical dating
The main recorded earthquakes took place on 2 August 1157, on 29 June 1170 and on 20 May 1202, the latter two having explicitly been mentioned to have had serious effects on Sāfītā, especially the earthquake of 1202. As Robert d’Auxerre wrote in his chronicle on the events of 1202: “… Castrialbi maxima pars murorum et turrium in terram prostrate est.” [… the greatest part of the walls and towers of Castrum Album were thrown down to the ground] (Robert of Auxerre, Chronicon, xxvi, 261). Clearly this remark is not about the donjon, but about the surrounding walls and towers.
Assigning the major damage of the donjon to the 1202 earthquake is based on indirect reasoning:
- • arguments related to the history of art place the construction of the donjon into the 12th century (early Gothic architecture);
- • there was a great tower standing in 1202, which has been seriously damaged by the earthquake of the same year, as the letter of the Philippe de Plessis reported (Mayer, 1972, p. 309);
- • the tower was in good and strong condition in 1212 (Wilbrand of Oldenbourg, Itinerarium Terrae Sanctae, 210). This means two things: either the letter written and sent immediately after the earthquake by Philip de Plessis overestimated the damages, or these damages have been successfully restored by 1212;
- • there are Crusader-style repairs and modifications of the Gothic hall and installation of a window for the church bell;
- • there was no major earthquake reported until 1271, when the Muslim forces occupied Sāfītā. After the fortress changed hands, it lost its strategic importance. Probably no major repairs occurred until the 20th century;
- • no matter how scanty the written reports seem to be individually, together they allow us to suggest that the major damage to the Sāfitā donjon occurred during the 1202 earthquake.
There is no historical data on the destruction of Khirbat al-Qurshiyya and ‘Ayn-Qadīb. Archaeological evidence (pottery dating) suggests the existence of these villages already in the AD 3rd century, and also in the AD 13th century. Since quarrying was very important in the village of Al-Qurshiyya, and the earthquake certainly disrupted this activity, we tentatively suggest that the earthquake occurred in the 13th century. (One should note that dates based on the material, form, and decoration of pottery, rarely provide us with an accuracy better than ±100 years).
However, we cannot exclude that the destruction occurred in late Roman times. In this case, the medieval pottery indicates a re-settlement of the site, especially near the water sources.
3.2.2 Dating the rockfall
The surfaces of the fallen blocks are ornamented by karren. Karren ridges and furrows are produced by the dissolution effect of rainwater flowing down on a rock face. Old karren furrows, produced in original orientation in the cliff, are 20–30-cm wide and several centimetres deep. These are overprinted by the younger karren rill system (Fig. 4b). A new karren, formed after the rockfall event, encloses a ∼45° angle with the old karren. The new ones are up to 37 mm wide and up to 9 mm deep (Table 1).
Rate of karren formation (karst dissolution) on inland surfaces is about 10–13 μm/year (ranging from 9 to 38 μm/year) on sparitic limestone, up to 38 μm/year on micritic limestone. The mean value is 18 μm/year (Furlani et al., 2009). These values apply to the Karst mountains along the coast of the northeastern Adriatic Sea. The higher elevations in the Jabal Ansāriyya, where Khirbat al-Qurshiyya is located, have a wet, oceanic climate (Mediterranean climate) (Wirth, 1971), very similar to that of the Karst in the Dinaric Mountains, where the longest series of detailed surface lowering measurements are available (Table 2) (Furlani et al., 2009).
Climate parameters of the Karst Mountains in the northeastern Adriatic region and in coastal Syria (after Furlani et al., 2009 and Wirth, 1971).
Parameter | Karst | Coastal Syria |
Distance from the shore | 10 km | 35 km |
Altitude above sea level | 300 m | 900 m |
Annual precipitation | >1300 mm | 900–1200 mm |
Mean annual temperature | 12 °C | 15 °Ca |
Winter | Cool, rainy | Cool, rainy |
Summer | Hot, dry | Hot, dry |
a Average of annual minimum and maximum temperatures.
The average age of the rockfall, based on the minimum 8 μm/year dissolution rate, is 763 years, i.e. the middle of the 13th century; based on the maximum 22 μm/year dissolution rate, it is 277 years, i.e. the middle of the 18th century; based on the average 18 μm/year rate, it is 339 years, i.e. the late 17th century. The error margin is high. The measurements used for comparison were made in the modern Mediterranean in Karst, while we compare them to surfaces developed during the Late Medieval climate optimum and/or the early Little Ice Age in the Jabal Ansāriyya. Therefore none of the data listed above can be interpreted as real age. We can be sure only that the earthquake that destroyed Khirbat al-Qurshiyya occurred during the last millennium.
3.2.3 Intensity
Shaking intensity is assessed based on earthquake archaeological effects (Rodríguez-Pascua et al., 2011), and is correlated to the EMS98 (Grünthal, 1998) and ESI07 (Michetti et al., 2007) macroseismic scales in conformity with Rodríguez-Pascua et al. (2013) (Table 3). Most of the correlations we accept here are to the ESI07 intensity scales, except the intensity value for rockfalls, for which we prefer the minimum value of EMS98 instead of the value IV of the ESI07 scale.
Intensity of shaking in Sāfitā, Al-Qurshiya and Ayn Qadib. Intensity values are after Rodríguez-Pascua et al. (2013). Intensity data are minimum values only.
Feature | Location | Minimum intensity after Rodríguez-Pascua et al. (2011) | Suggested intensity range for Sāfitā | Remarks |
Dropped keystones | Sāfitā, Gothic hall | VI–VII | VII–XII | |
Rotated masonry blocks in walls | Sāfitā, western façade | VIII–IX | IX–XII | |
Displaced wall | Sāfitā, northern and southern façades | VII–VIII | VIII–XII | Extremely thick, 2.5-m wall, broken and displaced |
Rockfall | Al-Qurshiya Ayn Qadib |
III–IV | III–XII | |
Fallen and oriented columns | Al-Qurshiya | V–VI | VI–XII |
The strongest construction type considered by the EMS98 scale is masonry. Sāfītā—built by opus caementitium or Roman concrete, covered by dressed masonry—is certainly at the most earthquake-resistant end of the building material continuum. Roman concrete walls 2.5 m thick on the upper level certainly can bear higher seismic load than ordinary masonry, where stone is just fitted to stone, even if using mortar. Walls and roofs built of opus caementitium, several metres thick, should have a class of their own, to be included at the top end of the construction materials considered by the EMS98 scale.
Sāfītā was assigned an intensity VIII by Ambraseys and Melville (1988), and VIII–IX (Guidoboni and Comastri, 2005: 231) based on historical documents. We suggest a minimum archaeoseismic intensity IX for Sāfītā.
3.2.4 The causative fault
The three locations discussed here have a common element of seismic destruction: an approximately north–south direction of shaking (error ±20° at least).
Sāfītā is less than 20 km from the Dead Sea Fault. Al-Qurshiyya and ‘Ayn-Qadīb are a mere 5 km away from the Missyaf segment of the same fault. The Yammouneh segment of the Dead Sea Fault, the causative segment for the 1202 earthquake, is only 50 km to the south.
Meghraoui et al. (2003) identified the segments of the Dead Sea Fault. The closest one, the Missyaf segment fractured the last time in AD 1170, i.e. before the construction of the donjon as we see it today in Sāfītā. The Yammouneh segment faulted in AD 1759 for the last time. It was also responsible for the 1202 earthquake, the largest one in the Near East in historical times (Ambraseys and Melville, 1988; Daëron et al., 2005).
Historical data suggest that the AD 1202 earthquake caused the crosscutting damage of Sāfītā donjon as we see it today. This event is known to have had its epicentre along the Yammouneh fault (Daëron et al., 2007), ca. 50 km south of Sāfitā, not by the Missyaf segment of the Dead Sea Fault, a mere 5 km to the east. The latter slipped in AD 1170. Both seismic events had a similar magnitude, ∼7.5 (Meghraoui et al., 2003).
4 Conclusions
Three sites nearby the Dead Sea Fault in coastal Syria were studied. Sāfītā castle (built in the late 12th century), Khirbat al-Qurshiyya and ‘Ayn-Qadīb villages of Late Antique origin are located in close proximity to the Missyaf segment. However, both historical data on timing, and observations on the north–south direction of major damages, parallel to the fault, exclude this segment as the causative fault, where the last major earthquake was in AD 1170. The AD 1202 earthquake, fracturing the Yammouneh segment farther to the south seems to be the causative event.
Acknowledgements
The authors have been supported by Hungarian National Science Foundation grants K 67.583 and PD 77.585. This is Syro-Hungarian Archaeological Mission publication No. _XX_. This article is a contribution to the UNESCO-funded International Geoscience Programme IGCP 567, “Earthquake Archaeology: Archaeoseismology along the Alpine–Himalayan Seismic Zone”. We are grateful to two anonymous referees, whose criticism significantly improved the content and presentation of the paper. M.K. is grateful to Judit and István Dunkl for their hospitality in Göttingen while writing this paper. Yann Klinger and the Institut de physique du globe (Paris) are thanked for the possibility to attend the Tectonics of the Levant Fault and Northern Red Sea workshop, held in Paris on 8 and 9 July 2013.