1 Introduction
Bovine Spongiform Encephalopathy (BSE) was first identified in the United Kingdom (UK) in November 1986 〚1〛. By the beginning of 2001, over 177 000 cases of BSE had been confirmed in Great Britain (figure 1). Epidemics have also occurred in other European countries, including Northern Ireland, Republic of Ireland, Switzerland, Portugal and France (figure 1). The disease in cattle is thought to have originated from supplementary feed containing meat and bone meal (MBM) contaminated by a scrapie-like agent derived from sheep or cattle 〚2〛, although much debate continues over its origin. The disease was made notifiable in June 1988 and in July 1988 a ban on the use of ruminant-based MBM in cattle feed came into force in Great Britain 〚3〛. Although the ban did not prevent all infections and cases in animals born after 1988, the number of cases of BSE in Great Britain has been declining rapidly for the last few years.


BSE cases in Europe. a. Great Britain; b. Northern Ireland, Ireland, Switzerland, Portugal and France.
In 1996, the UK government announced a possible link between BSE and a new variant of Creutzfeldt-Jakob disease (vCJD) in humans. Since this time, scientific evidence in support of the hypothesis that vCJD is a direct consequence of exposure to the aetiological agent of BSE has been strengthened 〚4, 5〛. By the end of 2000, 84 deaths from vCJD had been reported, with a further 2 confirmed deaths to date in 2001, 4 probable cases awaiting confirmation, and a further 7 probable cases still alive. Predicting the size of any future epidemic of vCJD remains difficult because of the many uncertainties in the key biological and epidemiological processes that will determine the typical course of infection and transmission.
In this paper, we will give an overview of the main mathematical and statistical analyses that have been undertaken by our group to better understand the transmission dynamics of BSE and vCJD. This includes analysis of the extensive database of BSE cases maintained by the Central Veterinary Laboratory in Great Britain, analysis of BSE epidemics in Northern Ireland, Portugal, France, and the Republic of Ireland and projections of the future scale of the epidemic of vCJD in humans in Great Britain.
2 Modelling the transmission dynamics of BSE
2.1 Back-calculation model
The model developed by our group to study the transmission dynamics of BSE in cattle is based on the back-calculation models developed for AIDS 〚6–8〛. These specifically relate the incidence of new infections over time to the onset of symptoms for diseases in which the incubation period is long and variable.
The basic principle of back-calculation can be explained in the following simple example. If we let denote the cases at time u and the incidence of infection at time t, then the relationship between these two quantities is given by
In our model, the probability that an animal born at time t0 onsets at age u is given (ignoring saturation effects) by
Working from the inside bracket outwards, is the probability that an animal is maternally infected at birth and has an incubation period of length u. The second term inside the bracket is the probability that an animal is infected from contaminated feed. is the feed risk when the animal is aged a, denotes its age-dependent susceptibility to infection, and reflects the probability that its incubation period is length u-a. As for the simple back-calculation model, we then sum over all possible ages that they could have become infected up to age u, their age at onset of clinical signs. Finally, outside the bracket, denotes the probability that they would survive to age u (in the absence of disease) and is the probability that the case is reported at the calendar time of onset. For Great Britain and Northern Ireland, the model was fit to the age and time-stratified confirmed case data using maximum likelihood methods 〚9–11〛. For Portugal, France, and the Republic of Ireland a simplified version of this model was fit to the age- and time-stratified case data 〚12, 13〛.
2.2 Transmission routes
The main source of transmission of BSE from animal to animal is thought to have occurred through contaminated feed 〚2〛. The time-profile of the risk of infection to animals therefore varied in tandem with the epidemic of infected animals, peaking in 1988/89, some four to five years prior to case epidemic peak 〚9, 10〛. Whilst consumption of feed may vary by age and by herd-type, models of the transmission dynamics of BSE suggest that age-dependent changes in the susceptibility of cattle contribute far more to age variation than variation in feed intake 〚9, 10, 14〛.
A second possible route of transmission of BSE was direct maternal transmission from dam to calf. Several studies have been undertaken to test for the presence of this transmission route. The main study was a pair-matched cohort study undertaken by staff at the Central Veterinary Laboratory in the UK 〚15〛. The results of the study revealed an enhanced risk of disease of approximately 10 % in animals born to affected dams over those born to unaffected dams. However, during the study, both maternally exposed and unexposed animals were fed with potentially contaminated food. As a result, it was not possible to determine from the summary results whether the enhanced risk was due to direct maternal transmission of the aetiological agent, genetic predisposition to disease, or some combination of both factors. In an attempt to resolve this dilemma, detailed statistical analyses were undertaken using a variety of models 〚16–18〛, in particular examining the maternal risk enhancement in relation to the disease stage of the dam 〚16〛. The results demonstrated a greater enhanced risk in animals born to affected dams that were close to onset of disease over those born to affected dams that were early on in their incubation period, suggesting some component of direct maternal transmission. Similar estimates of the rate of direct maternal transmission were obtained in a second, independent analysis of the BSE case database 〚19〛.
2.3 Incubation period
Information on the incubation period of BSE was obtained from an experimental study on the oral dosing of cattle with brain from other cattle affected by BSE. Kaplan-Meier survival curves based on these data are given in Anderson et al. (1996) 〚9〛. The first animal (which received three doses of 100 g) showed clinical signs of BSE at 34 months, with the median incubation period in all groups between 40 and 50 months. A second independent source of information regarding the incubation period of BSE is the maternal cohort study 〚15〛. In this study, the mean age at onset of clinical signs in the BSE-affected animals was approximately 64 months. By assuming that these animals were infected close to birth, an upper bound for the incubation period length for these animals is approximately 5 years.
A number of functional forms were considered in the back-calculation models to describe the shape of the incubation period distribution 〚9, 10〛. The best fitting model had a mean incubation period of 5.0 years, with a variance of 1.60 years2. It is worth noting that no cases were observed in animals under 2 years of age, a characteristic delay shared with other transmissible spongiform encephalopathies 〚20〛.
2.4 Age-dependent susceptibility/exposure
Analysis of the BSE case database strongly suggests some age-dependency in susceptibility or exposure 〚14〛. The peak age at onset of clinical signs for most cohorts was approximately 5.5 years. Of several forms tested, the best fitting model gave an age-dependent susceptibility distribution strongly peaked between 6 and 18 months of age, with a rapid decline thereafter 〚9, 10〛.
2.5 Survival
Information on the survival pattern for British cattle was obtained from the National Milk Records for a subset of dairy herds 〚9, 14〛. These patterns ignore male animals (a tiny fraction of the population over 2 years of age) and do not include beef herds. The age distribution of breeding beef cattle give mean ages approximately 6 months older than for dairy cattle; however the majority of BSE cases have occurred within dairy herds. The survival curve shows a large drop in the number of animals surviving between 1–2 and 2–3 years old, reflecting the age at which a large number of animals are slaughtered for beef. A similar survival curve was independently obtained from the age structure of the Northern Ireland herd between April 1996 and December 1997 〚9, 14〛. From Portuguese data on the number of calves born each year and the age category of slaughtered animals, a slightly different survival curve was obtained, with the majority of cattle slaughtered approximately 1 year earlier than in Great Britain 〚12〛.
3 Estimates of infections and future cases of BSE in Great Britain and Northern Ireland
3.1 Future cases
By estimating the past incidence of infection of BSE, we are able to make short-term predictions of the future numbers of cases. The methods work by deriving information about the incubation period distribution from earlier in the epidemic. The incidence of infection close to the time of analysis is then estimated using information on those cases that onset with short incubation period distributions. This infection incidence can then be combined with the information on the incubation period distribution to predict case numbers in the following years. Because no cases of BSE were observed in animals under two years of age, it is impossible to obtain information on the infection hazard up to approximately two years prior to analysis. However, even in the initial analysis in 1996, the infection hazard was estimated to drop to very low levels by mid 1994.
Our model was originally fit to the BSE confirmed cases to the end of 1995 〚9〛. Figure 2a shows these predictions (with prediction intervals) and the actual case numbers confirmed up to the end of 1999. The results show that the model is able to accurately make short-term predictions. However, direct comparison of the predictions with the observed case numbers is complicated by the many additional control policies that have been initiated since this time. These include the selective cull agreed in the Florence agreement, which targets animals born in the same holding and cohort as BSE cases, and the offspring cull, in which animals born to (later confirmed) BSE cases are slaughtered.
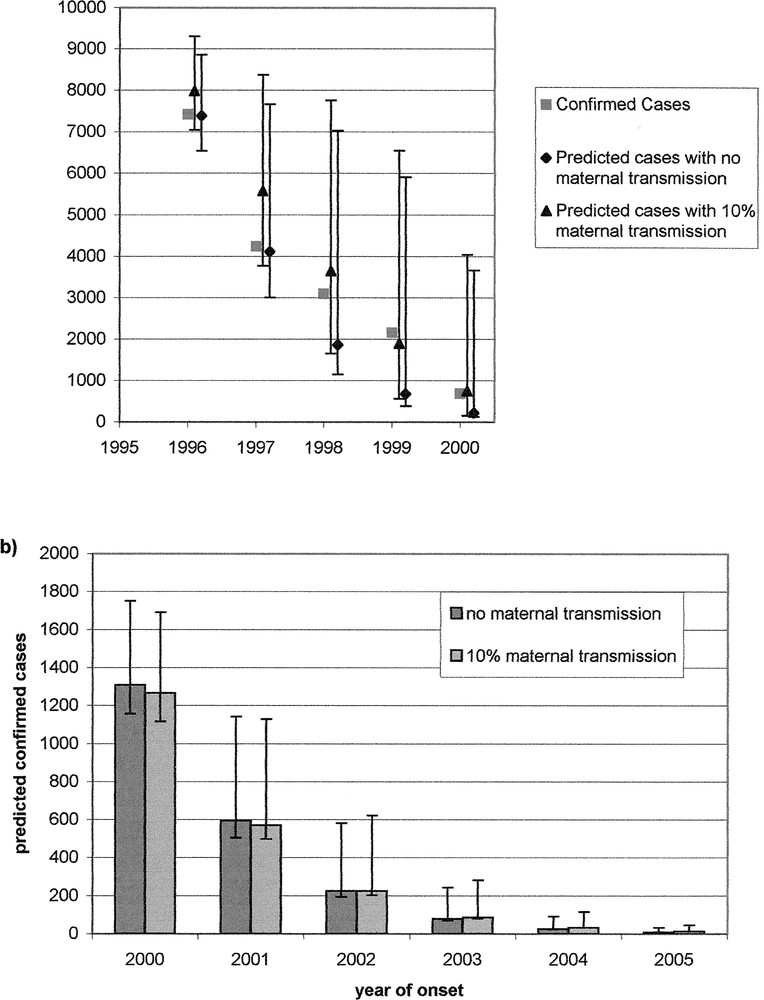
Projections of future case numbers in Great Britain. a. Projections made in 1996 based on case data to end of 1995 and the actual confirmed number of cases to end of 2000; b. Predictions for 2001-2005, based on cases confirmed by 1 August 2000.
Figure 2b shows updated projections of the future case numbers in Great Britain based on the confirmed cases to 1 August 2000. The results predict that the incidence of BSE will continue to decline in Great Britain. Since the estimated risk from contaminated feed has dropped to negligible levels from 1996 onwards, the majority of cases are now predicted to arise through maternal transmission from dam to calf.
3.2 Infected animals slaughtered for human consumption
Because of the long incubation period of the disease, many animals incubating the disease will have been slaughtered for human consumption prior to the onset of clinical signs. Whilst the numbers of cases arising over time is clearly of concern to the agricultural industry, the number of pre-clinical infected animals slaughtered for consumption must form the basis of any risk assessment of the exposure of the population to potentially infectious material. The back-calculation model allows estimates of the numbers of infected animals over time to be made from the BSE case data. We estimated that only roughly 1 in 5 infected animals is confirmed as a clinical case of BSE. This is because the majority of animals are slaughtered at between 2 and 2.5 years of age, whilst the mean incubation period of BSE is 5 years. We estimate that approximately 750 000 infected animals entered the human food supply in Great Britain 〚9, 10〛 and 9 500 in Northern Ireland 〚11〛.
Current estimates of numbers of infected animals slaughtered for consumption must include adjustment for the public health measures that are now in place. In particular, the Over-Thirty Months Scheme (OTMS) has removed animals over this age from entering the food supply. This is based on the observation that no BSE cases have been confirmed in animals under two years of age, and hence these animals will, if infected, be at an early stage in the incubation period and hence potentially less infectious. Furthermore, younger animals will now have been born after control measures were tightened in 1996, and hence should be at lower risk of being infected than older animals. Figure 3a shows our estimates of the numbers of late-stage infected animals entering the human food supply in Great Britain in 2000, stratified by the age of the animal. Confidence bounds for these estimates are not shown, but are wide due to the small numbers. The results demonstrate the impact of the OTMS scheme in reducing the risk from animals close to (within a year of) disease onset. These numbers represent a very small risk compared to the past risk experienced by the British population.
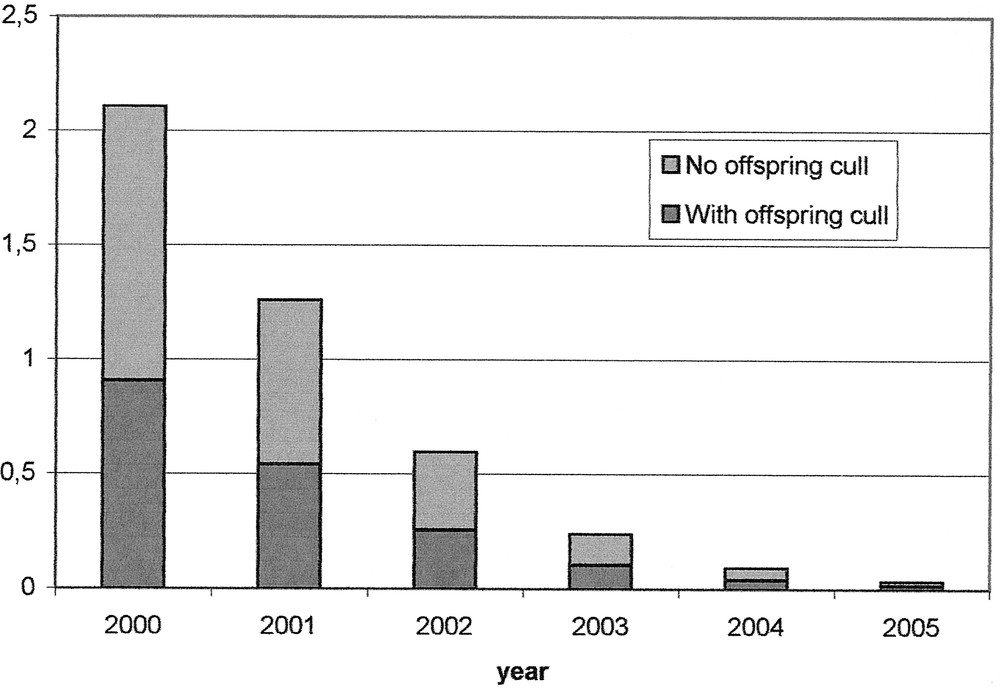
Estimates of numbers of infected animals under thirty months of age within a year of onset of clinical signs in Great Britain for 2001–2005, stratified by the age. Only those under thirty months of age are used for human consumption.
4 BSE in other European countries
Although BSE was first diagnosed in Portugal in 1993, attention focused on BSE in Portugal in 1998 when incidence increased dramatically from 1997 to 1998 〚21〛. In November 1998, the European Commission banned the export of meat and live cattle from Portugal. Using the maximum likelihood age-at-onset distribution estimated from the back-calculation analysis of the epidemic in Great Britain (the convolution of the age-dependent exposure/susceptibility distribution and the incubation period distribution) and independently estimated survival distributions, a simplified back-calculation model was fitted to the age- and time-stratified incidence data on Portuguese born cattle estimating Portugal-specific under-reporting parameters 〚12〛. On the basis of these model fits and sensitivity analyses, the analysis of BSE incidence prior to 1999 produced robust predictions that the incidence of BSE cases in Portugal would peak in 1999 with BSE case-incidence declining thereafter. The observed incidence in Portugal did peak in 1999, as predicted, with 170 confirmed cases, a 1999 annual incidence surpassed only by Great Britain.
More recently, the incidence of confirmed clinical cases of BSE has increased dramatically in both France and the Republic of Ireland (figure 1). Similar methods were applied to these stratified incidence datasets to estimate the past incidence of BSE infections, rather than cases, adjusting for under-reporting which was estimated independently in each case. It was estimated that roughly 7 300 French cattle (95 % confidence interval: 4 700–9 800) were infected with BSE since mid-1987 allowing for under-reporting 〚13〛. This estimate was reduced to 1 200 (900–1 400) if it was assumed that all BSE cases in France were reported and confirmed. However, the inclusion of under-reporting in the model significantly improved the fit of the model to the data (p << 0.001). Furthermore, it was estimated that 100 infected animals (80–120) were slaughtered for consumption during 2000 in France, with 52 (42–62) of these estimated to have been slaughtered within 12 months of the clinical onset of BSE. By comparison, the estimated number of infected animals slaughtered for consumption within 12 months of the clinical onset of BSE during 2000 in Great Britain was 1.2 (0–4). This number is so low, despite the higher case incidence of BSE in Great Britain compared to France, because British legislation bans the sale for consumption of beef from animals over 30 months of age.
A similar analysis of BSE in the Republic of Ireland suggested that roughly 10 000 Irish cattle had been infected. As in France, the estimated number of late-stage animals slaughtered for consumption during 2000 in Ireland was greater than in Great Britain (an estimated 150 BSE infected animals of which 72 were estimated to have been within 12 months of the clinical onset of BSE) 〚22〛. Furthermore, since the cattle population of the Republic of Ireland is roughly 7 million, compared to 21 million in France and 9.6 million in Great Britain, the population-adjusted risk in the Republic of Ireland in 2000 was roughly 230 times greater than in Great Britain, while the population-adjusted risk in France in 2000 was roughly 25 times greater.
5 Predicting the scale of the vCJD epidemic
By the beginning of April 2001, there had been 97 confirmed and probable cases of vCJD in Great Britain, 3 cases in France and 1 case in Ireland. The time series of these cases (3 deaths in 1995, 10 in 1996, 10 in 1997, 18 in 1998, 15 in 1999, 28 in 2000 and 6 to date in 2001 with 7 suspected cases still alive) show a statistically significant increase in the incidence of disease 〚23〛, as would be expected in the early phases of an epidemic 〚24〛. However, there remains great uncertainty in the future course of the epidemic, due to the many uncertainties in the epidemiology of the disease. In this section we give an overview of our work modelling the future course of the vCJD epidemic using scenario analysis.
5.1 Model structure
Our model uses estimates of the numbers of BSE-infected cattle that entered the human food supply as the risk profile for vCJD. Oral route transmission of vCJD, BSE and other TSEs between species has been demonstrated in a number of experiments 〚25, 26〛 and many TSEs appear to be able to cross host-species barriers with ease. Between 1980 and 1996, approximately three-quarters of a million cattle infected with BSE were slaughtered for human consumption in Great Britain 〚9〛. Whilst the possibility of other transmission routes (for example, vertical transmission or via blood or surgical instruments) cannot be excluded, we do not consider these routes in our analyses. The risk of infection over time is therefore determined by the pattern of exposure to infected cattle tissue, which in turn is determined by the past pattern of the BSE epidemic and the regulations governing which tissues can be used for human food.
Our model assumes that the probability that an individual develops clinical disease at time u and age a is
5.2 Epidemiological determinants of the epidemic
Our results demonstrate the importance of two key factors in determining the course of the vCJD epidemic. The first is the average number of cases, denoted r, arising from consumption of maximally infectious (in the last 6 months of the incubation period) animal. This figure incorporates many unknowns including consumption patterns, the relative infectiousness of different tissues at different cattle incubation stages, and the magnitude of the species barrier. As the ‘force of infection’ parameter in the model, it determines the overall magnitude of the epidemic. The second key factor is the incubation period distribution. If r is known, then the incubation period distribution determines only the time-course of the epidemic. However, since r is unknown and these two quantities are intrinsically related, the incubation period provides a proxy determinant of the magnitude of the epidemic. Figure 4 shows the relationship between the mean incubation period and r for approximately 15 000 epidemic scenarios that are consistent with the case data to the end of 1999 (as reported by March 2000). Small epidemics tend to occur with short incubation periods (with small variance) and lower values of r, whilst larger epidemics arise from long and variable incubation periods and larger values of r 〚29〛.

Relationship between mean incubation period and the average number of cases arising from consumption of one late-stage animal (r). Each point represents an epidemic scenario that is consistent with the vCJD case incidence data to the end of 1999 as reported by 1 March 2000.
To reduce the current uncertainty in the future course of the epidemic, some information is required on either of these two key determinants. Whilst information on the incubation period of other human TSEs can inform the incubation period of vCJD, there are many differences (including routes of transmission, dose and the effect of the species barrier) that limit such comparison. Furthermore, until the epidemic has conclusively peaked, it is impossible to infer information on the incubation period distribution from the case numbers alone. In contrast, it is theoretically possible to bound r by the number of individuals who consume meat or meat products derived from one animal. Unfortunately, such data are difficult to obtain given the complexity of the food production process.
5.3 Age structure
A particular characteristic of vCJD is the age at onset of the disease. In contrast to classical forms of CJD, which typically affect those over 60 years of age 〚30–32〛, the median age at death from vCJD is 29 years 〚23〛, with the majority of cases arising in young individuals. Such a distribution could not arise without some form of age-dependency in the infection process. There are three possible causes; a) some form of age-dependent susceptibility to infection (as was observed for BSE in cattle); b) age-dependent exposure, for example, to riskier foods; and c) an age-dependent incubation period, whereby younger infected individuals onset earlier than older infected individuals. It is impossible in epidemiological modelling to distinguish between age-dependent susceptibility and/or exposure to infection. However, for an age-dependent incubation period one would expect to see a shift in the age distribution towards older individuals as the epidemic progresses. In the first 5 years of the vCJD epidemic, the age distribution has remained remarkably constant. This now suggests that the age distribution of the cases must, at least in part, be due to some form of age-dependent susceptibility or exposure 〚29〛. However, the age range of those most susceptible/exposed to infection remains wide, with 95 % bounds on the distribution in scenario analyses ranging from 0 to approximately 50 years 〚29〛.
5.4 Genetics
To date, all tested vCJD cases have been in individuals who are methionine homozygous (MM) at codon 129 of the PrP gene. Approximately 40 % of the Caucasian population have this trait, with approximately 10 % valine homozygous (VV) and the remaining 50 % heterozygous (MV) 〚33〛. Homozygosity at this gene is known to be a risk factor for other human TSEs, including sporadic CJD and kuru 〚34–37〛. However, cases of sporadic CJD and kuru have also arisen in heterozygotes 〚34–37〛. Thus it is likely that MM-homozygotes are either more susceptible to vCJD infection and/or have shorter incubation periods than other genotypes.
To make any sensible predictions of the future course of the epidemic, it is necessary to restrict the generated scenarios to those consistent with the current vCJD case data. As cases have only been observed in MM-homozygotes, our analyses are restricted to this population, as we cannot constrain epidemic scenarios in other genotypes. Hence, all figures are based on the assumption that only 40 % of the population are susceptible to infection. However, it is worth noting that the epidemic scenarios in other genotypes are likely to result in a lower per capita disease incidence. In the simplest case, if individuals in these genotypes are less susceptible to infection, the resulting per capita infection (and hence disease) incidence will be lower. If individuals with these genotypes have longer incubation periods, then we must consider the scenarios in which the epidemic size will be greatest. These scenarios tend to be the result of long incubation periods where very large numbers of cases do not arise because many infected individuals die of other causes. In these other genotypes with lengthened incubation periods relative to MM-homozygotes, the effect of mortality from other causes becomes greater, and hence the per-capita incidence of disease will also be less than that observed in MM-homozygotes.
5.5 Future course of the epidemic
Our original analyses, undertaken using the vCJD case data to the end of 1997 〚20〛, showed a wide range of possible epidemic scenarios, with total cases to 2040 ranging from very small numbers to over a million. More recently, our updated analyses 〚29〛 have shown that very large epidemic scenarios are no longer consistent with the case data. Based on the vCJD cases to the end of 1999 as reported by March 2000 (which did not include 1 case in 1998 and 2 cases in 1999 that were reported after this time), our analyses suggested a reduction in the upper bound on epidemic size to approximately 140 000 〚29〛. This reduction in the upper bound on epidemic size arose because the rate of increase of cases observed was not as great as would be required for very large epidemics. However, much of the reduction was also obtained from the stable age structure that has been observed, with the majority of cases arising in young individuals. This stable age structure suggests some degree of age-dependent susceptibility/exposure and hence limits the size of the population that are at highest risk of infection. Much concern has arisen at the rise in cases reported in 2000, in particular, given that this is the first point at which is has been possible to demonstrate a statistically significant increase in cases 〚23〛. The majority of the epidemic scenarios contained in our last analysis are consistent with this number of cases, and hence addition of these case numbers is not expected to dramatically alter current bounds on epidemic size.
Studies are currently underway to test tonsil and appendix material for the presence of the abnormal prion protein (PrPSc). Interpretation of these results is complicated by a lack of knowledge of when in the incubation period tests are able to detect infection 〚38〛. Appendix material removed prior to the onset of symptoms has been tested from two patients with vCJD. In the first, PrPSc was detected in material removed 3 years prior to the onset of symptoms 〚39〛. In the second, PrPSc was not detected in material removed approximately 10 years prior to the onset of symptoms 〚40〛. These data give the only indication of when in the incubation period it is possible to detect infection in appendix material. Whilst it is possible to detect PrPSc in all tonsil material tested from symptomatic vCJD patients 〚41, 42〛, no comparable data are available on pre-clinical infection. Furthermore, no data exist on the sensitivity and specificity of these tests in pre-clinical infection.
Our model can be used to explore the relationship between the sensitivity (and specificity) of tests throughout the incubation period and epidemic size, and hence can be used to interpret the results from these screening studies 〚43〛. Preliminary results testing mostly appendix tissues revealed a negative result (0 infections detected in 3 170 tissues) 〚40〛. From our model results, if tests were able to detect infection in the last 75 % of the incubation period (with 100 % sensitivity and specificity), then these results reduce the upper bound on epidemic size from approximately 140 000 to 80 000 cases 〚29〛. However, if tests were only able to detect infection in the last 50 % of the incubation period, the results from the preliminary screening data do not provide any additional information.
6 Conclusions
Although BSE has continued to appear in native-born animals in previously unaffected countries (for example, Denmark and Italy), the declining incidence in confirmed cases worldwide is encouraging. However, there is no room for complacency in European Member States in the face of BSE. New measures that came into force in the EU on 1 January 2001 require the testing for BSE infection of all cattle over 30 months of age slaughtered for human consumption (Cattle over 30 months of age can be consumed in Member States other than the UK provided they have tested negative for BSE infection.). If the testing is performed according to standard protocols and ages of tested animals are recorded, the data collected from the screening of animals over 30 months of age should provide a valuable source of information for future risk assessment. While debates continue about the level of reporting of clinical BSE cases in various countries, the testing data could facilitate an independent assessment of BSE reporting levels and assessments of the relative risks in Member States.
The future of the vCJD epidemic is much less certain. Regulations in place in the UK and throughout Europe mean that there is virtually no risk of ongoing feed-borne transmission of BSE to cattle. However, the large numbers of infected animals consumed throughout the epidemic, combined with the remaining uncertainties in the epidemiological parameters relating to cattle-to-human transmissibility and the human incubation period, mean that the possibility of tens of thousands of clinical vCJD cases cannot be ruled out. Screening programmes and epidemiological research will continue, but for the time being the consequences of past consumption of meat from infected animals remain unknown.
Acknowledgements
This work was supported by The Royal Society (ACG, NMF) and The Wellcome Trust (CAD, RMA).