1 Introduction
In the tropical Atlantic, important tuna concentrations are found seasonally (from November to February) in an oligotrophic area off the African Coast (2–5°N; 10–20°W) out of the productive system related to the equatorial divergence. In this area, from June to August, satellite observations and modelling have demonstrated the existence of long instability oscillations along the thermal front between the south equatorial current, the north-equatorial counter current and the under-equatorial current. Together with the latitudinal shear between these currents, are associated anticyclonic eddies 〚1, 2〛 and vertical movements. As observed in the Pacific 〚3〛, these vertical movements could bring nutrients and a subsequent local enrichment that could enhance phytoplankton and zooplankton production and sustain the main tuna prey, a small planktophagous mesopelagic fish, Vinciguerria nimbaria 〚4〛.
To try to understand how oceanic processes affect the ecosystem and cascade through the food web to the higher levels, the ‘Institut de Recherche pour le Développement’ (IRD) set up a multidisciplinary program ‘Production induite en zone de convergence par les ondes longues océaniques’ (PICOLO) including five cruises performed at different hydrological seasons.
In this article, we analysed the relationships between sestonic particles and zooplankton and their variations with hydrological factors. Due to the quantitative importance of the copepods (often > 80% of total mesozooplankton) in surrounding areas of the Gulf of Guinea and equatorial waters 〚5–7〛 and their importance in the diet of tuna preys 〚8,9〛, we focused our study on copepod ingestion and metabolic rates during two cruises performed at two different hydrological seasons.
2 Material and methods
PICOLO 1 cruise (P1), in winter (January–February 1997) at the end of the tuna fishing period, was characterized by a marked thermocline and a weak divergence south of 0°30 N. PICOLO 2 cruise (P2), in summer (June–July 1997), was characterized by a strong equatorial divergence and a marked tropical instability wave north of the equator. Sampling and on board experiments were carried out at stations located at different latitudes on north–south repeated transects at 15°W and 20°W during P1 and P2 respectively.
Every 20 miles, salinity and temperature were measured with a CTD probe (Sea Bird 911). Chlorophyll a was measured fluorimetrically after methanol extraction 〚10〛 on samples collected with a rosette at different depths (10, 20, 30, 40, 50, 60, 70, 80, 90, 100, 125, 150 m) in the euphotic zone.
At each hydrological station, mesozooplankton was sampled with a WP 2 standard net 〚11〛 fitted with a 200 μm gauze, hauled vertically between 0 and 100 m. Each station was sampled at least once at day and at night. Half-sample was preserved in buffered formaldehyde for taxonomic determinations. The other half was collected on a pre-weighed 60 μm gauze, rinsed with distilled water, dried at 60 °C during at least 24 h, and re-weighed to estimate the dry weight biomass 〚12〛.
On board, ingestion and metabolism experiments were carried out on zooplankton freshly collected between 0 and 100 m with the WP2 net. Immediately after the haul, sets of 10 to 50 (according to their size) copepods 〚13〛 were sorted and put in 600 ml incubation bottles filled with 0.2 μm-filtered water for metabolism experiments and with 60 μm-filtered water for grazing experiments. In both types of experiments, flasks without animals were used as control. The water collected with the rosette at the different depths was mixed in equivalent proportions and used as experimental water. The bottles were attached on a rotating wheel (1 rpm) to avoid particle sedimentation, and incubated during 12 h in the dark at the in situ temperature.
After incubation, a water sub-sample was gently siphoned with a small rubber tubing tipped with a 60 μm gauze to retain copepods and poured into a 50 ml BOD flask to measure the oxygen concentration with an YSI 58 oxygen probe compensated for temperature and salinity. A 10-ml sub-sample, collected in the same way, was used for N-NH4 measurements 〚14〛. A 150 ml sub-sample was preserved with filtered (0.2 μm) buffered paraformaldehyde and stored in the dark in a cold room until analyses with a Coulter Counter multisizer equipped with a 70 μm orifice to measure numbers and volumes of sestonic particles. Copepods were then collected from the experimental bottles by sieving on a 200 μm gauze; they were identified and weighed (dry weight). Carbon weight was estimated assuming a carbon/dry weight ratio of 0.45 〚15〛.
Respiration, excretion and ingestion rates were calculated from the difference between control and experimental bottles, according to the incubation time, the bottle volume, and the copepod weight. Results are expressed in μl O2 mg–1 h–1, μM mg–1 h–1 and μm3 mg–1 h–1 for respiration, excretion and ingestion respectively. Ingestion rates were expressed as carbon unit using Strathmann 〚16〛 equations for the relationships between carbon content and volume of phytoplankton cells. Respiration rates were also expressed as carbon assuming a respiratory quotient (RQ) of 0.97 〚17〛.
The coulter counter method is subjected to several biases, the main of which being a re-introduction of particles within the counted particle size range through feces and cell breakage 〚18〛. However, in our study, there was no evidence of such phenomena, for we never found significant negative difference between experimental and control flasks in any considered channel. Moreover, it must be emphasized that our experiments were run over a short incubation time (12 h) and with a relatively low animal density (17 to 80 ind l–1), which are conditions to obtain reliable electronic data, as argued by Paffenhofer 〚19〛. Indeed, in this experiments, most differences between microscopic and electronic counting were quite insignificant after 12 h, but occurred after 24 h. In our experiments, the copepod density in the experimental jars was sufficient to ensure a significant but not drastic decrease in particle volume, the percentage of reduction of particles during incubation time being always < 10%.
3 Results
Surface temperatures increased regularly from the equator (26.2 °C) to the convergence (28.1 °C) during P1 (Fig. 1a). They were lower during P2 (23.5 °C at 0°; 26.3 °C at 4°N) and increased markedly between the equator and the convergence except a slight decrease from 2° to 3°N. Integrated chlorophyll values were maximal at 2°N in P1, due to a deep maximum at 50–60 m. During P2, they were maximal at 0° and decreased northward. In both cruises, zooplankton biomasses were maxima near the divergence, and decreased sharply between 0 and 1°N and between 3 and 4°N. Moreover, a strong decrease also occurred between 5 and 6° N during P2. At the same latitudes, mean zooplankton biomass were always higher during P2 than during P1. These biomass followed the same latitudinal variations as chlorophyll in P2 (Spearman rank correlation coefficient, Rs = 0.8, p = 0.02) but not in P1 (Rs = 0). In both cruises, in situ zooplankton was dominated by omnivorous suspension feeders (60–80% of the zooplankton biomass) with the preponderance of the copepods Undinula darwini (P1), Clausocalanus furcatus, C. pergens and C. arcuicornis (P2) in most samples and Eucalanus crassus, E. mucronatus, Rhincalanus nasutus, Macrosetella sp. and Miracia efferata in a few samples. Experimental animals were in the same proportions.

Latitudinal variations of temperature (T), integrated chlorophyll (Chl) and zooplankton biomass (Zpk) (a) and of specific ingestion (I), respiration (R) and ammonia excretion (ENH4) rates (b) during PICOLO 1 (P1) and PICOLO 2 (P2) cruises.
Ingestion rates were always higher in P2 than in P1 (Fig. 1b). Ingestion and chorophyll peaked at the same latitude (2°N) during P1 but not during P2. Excretion rates and ingestion rates followed approximately a similar pattern of variations at P1 but not at P2. During P2, ingestion rates increased from 1°N to 4°N and decreased northward.
In both cruises, ingestion and food concentrations, expressed as particle volumes, displayed linear relationships, with significantly (p < 0.001) higher slope and intercept in P2 than in P1 (Fig. 2a). However, no correlation was found between the ingestion rates and in situ chlorophyll concentrations. A multiple correlation analysis between specific ingestion rates (I), particle concentration (C), average particle-size expressed as equivalent spherical diameter (ESDm) and individual weight (W) gave the following equation:
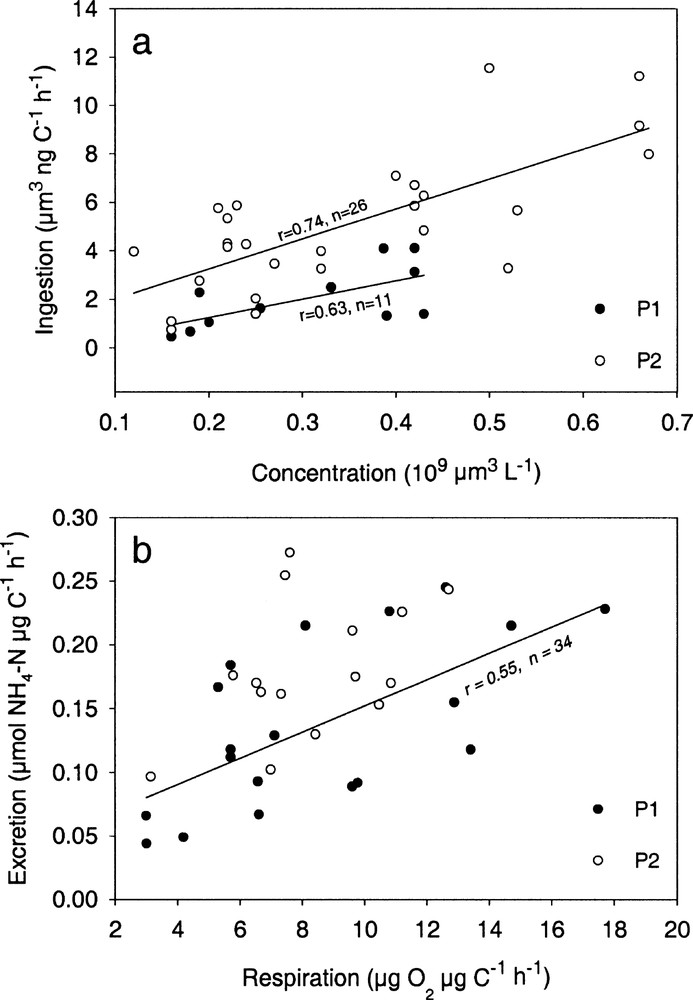
Plots of specific ingestion rates vs available particle concentration in feeding experiments (a) and plots of specific excretion rates vs specific respiration rates in metabolism experiments (b) during PICOLO 1 (P1, black dots) and PICOLO 2 (P2, white dots) cruises.
Respiration and NH4–N excretion displayed linear relationship with no difference between the two cruises (Fig. 2b). Oxygen/ammonia metabolic ratios (O/NH4–N) were generally low (4.8 ± 2.3 for P1 and 3.2 ± 1 for P2) and not significantly different between P1 and P2 (t-test, p < 0.05). Respiration, excretion and O/NH4–N ratio were not significantly correlated to particle size or concentration and to individual weight.
During P1 specific ingestion rates on seston particles, expressed as percentage of body carbon were low. Moreover, they were generally lower than respiration rates expressed as the same unit except for one experiment performed at 1°N (Fig. 3). During P2 in most cases, copepod suspension feeders displayed carbon ingestion rates higher than carbon respiration rates.
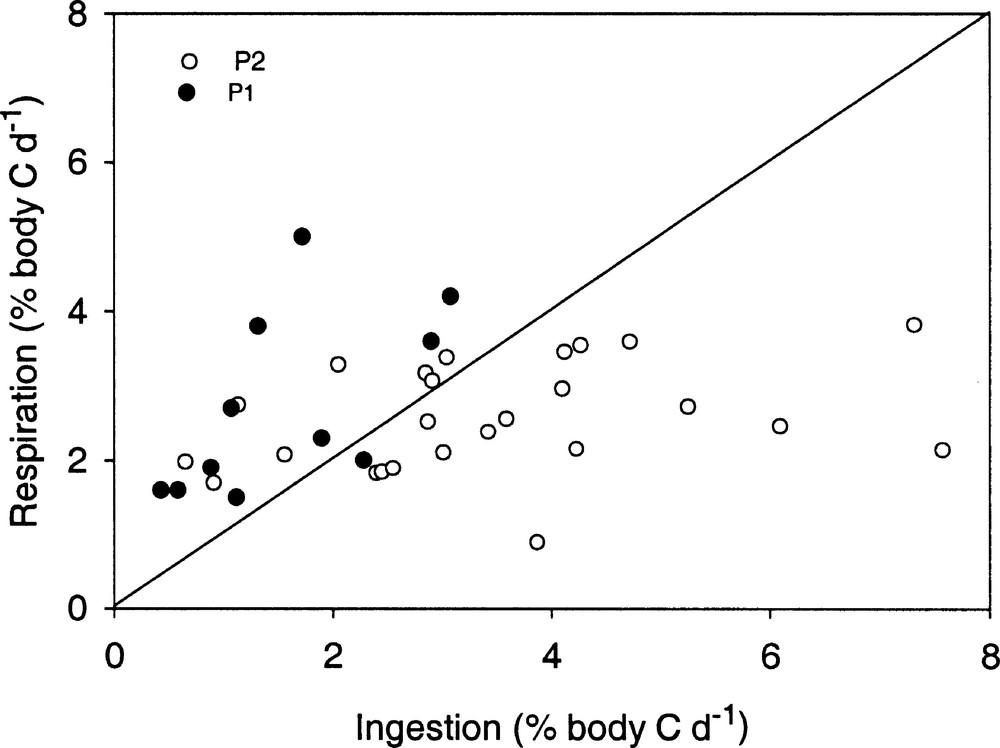
Plots of specific respiration rates vs specific ingestion rates during PICOLO 1 (P1, black dots) and PICOLO 2 (P2, white dots). The continuous line represents the equality line between ingestion and respiration.
4 Discussion
During P1, in boreal winter, a typical tropical structure 〚20〛 was observed between 1°N and 4°N. It was characterised by a strong thermocline and low surface chlorophyll but a deep chlorophyll maximum (DCM) between 1 and 3°N 〚4〛. In this area, we observed low zooplankton biomasses (10–20 mgdry weight 〚DW〛 m–3) similar to those observed previously in the tropical Atlantic 〚5,21〛. At 0°, despite a weak upwelling demonstrated by low surface temperatures, surface and integrated chlorophyll were low. However, high zooplankton biomass (twice to three folds higher than northward) suggests a previous and more or less continuous enrichment as observed in other upwelling areas 〚22,23〛.
During P2, in boreal summer, a strong equatorial upwelling was characterised by low surface temperatures (23 °C) around 0°N and high chlorophyll and zooplankton biomasses. From 1°N to 6°N, together with an increase of surface temperature, we observed a parallel decrease of chlorophyll and zooplankton biomass as observed in a north–south transect in the Gulf of Guinea 〚24〛. A marked tropical instability wave was evidenced at the north of the divergence from a temperature decrease between 2 and 3°N. This tropical anticyclonic vortex detected by satellite sensor was about 500 km in diameter and moved westward (35 cm s–1) during the campaign, transporting rich waters and plankton from the equator northward by horizontal advection. Moreover, this advected water downwelled (50 m day–1) in a frontal zone around 3°–4°N 〚25〛.
During both seasons, mean variations of ingestion and metabolic rates of copepods were not correlated to in situ chlorophyll or zooplankton biomass, suggesting lag responses and/or space and time heterogeneity related to water movements, as observed in the equatorial Pacific 〚26〛. However, the highest ingestion rates were observed at the latitude of the DCM during P1 and in the frontal area during P2.
Compared to literature data for marine copepods 〚13〛, ingestion rates observed during the two cruises were low (between 1 and 8% of body carbon day–1). However, they were always higher and appeared often sufficient to equilibrate metabolic expenditure during the instability period (P2) contrarily to what was observed during the stratified period (P1). The difference in ingestion rates between the two periods cannot be explained by temperature, which was lower during P2 than during P1. It could be partly explained by average individual weight of copepods, which depends upon the community structure. This factor, included in a multiple regression model for ingestion, was significantly lower (p < 0.001) at P2 than at P1. From the regression model, the role of food concentration on ingestion was highly significant and the slope of the ingestion–concentration equation was higher in P2 than in P1 experiments. The intercept was significantly lower in P1, showing that animals had lower feeding rates at comparable concentration. Moreover, there was no significant difference in experimental particle volume (p = 0.45) between the two periods, so that the role of food quantity to explain the difference in food acquisition is not evident. In contrast, the positive role of particle size on ingestion appears in the regression model, showing the advantage of large particles. Actually, although no significant difference appeared in the average particle size between the two periods, peaks of large particles (15–20 μm ESD) were more often observed during P2 than during P1, where 3–6 μm particles prevailed and were ingested. Recent findings 〚27〛 show that tropical copepods are capable of consuming seston particles < 8 μm in size. In our study, these small particles are perhaps partly undetected by the Coulter Counter (lower limit: 1.4 μm ESD), explaining the very low measured ingestion rates on seston, particularly in P1. They could correspond to bacteria, microheterotrophs or small size phytoplankton cells. Ingestion on heterotroph is more realistic than ingestion on picophytoplankton given (1) the absence of relationships between ingestion and in situ chlorophyll and (2) the very low values of metabolic O/NH4–N ratios observed in both cruises. Indeed, low values of O/NH4–N ratios express inclusion of proteinic substrate in the assimilated food 〚28〛. Thus, our results agree with recent studies proving the importance of the microbial web in oligotrophic waters 〚29,30〛 and the existence of changes from the classical phyto-zooplankton web to the microbial food web, in particular from eutrophic to oligotrophic waters in tropical and temperate zones 〚31–33〛.
In summary, our results suggest a similar trophic pathway during the instability period and the stratified period. In this pathway, microheterotrophs feeding on bacteria or small-size phytoplankton serve as main food for copepods. However, our results also showed differences between the two hydrological seasons, in particular a positive effect of the tropical instability wave on the secondary production. Nutrients brought by the vertical movements and the advected equatorial upwelled waters enhance the production of more diversified and small-sized phytoplankton accessible to microheterotrophs, which serve as main food for copepods. Then, the hydrodynamic event seems to have induced a quick cycling food chain. In contrast, during the typical stratified period, the same pathway likely occurs with lower fluxes.
Through the physiological response of copepods, our results show that physical processes affect the biological production. Indeed, the enrichment due to the instability wave, together with that resulting from the equatorial upwelling, could induce a change in the ecosystem functioning from an oligotrophic to a more productive one, capable to sustain terminal production.
Version abrégée
1 Introduction
Au large des côtes africaines, dans une zone particulière située à proximité de l’équateur (0–5°N, 10–20°W), sont pêchés à la fin de l’automne de grandes quantités de thons dont l’alimentation principale est constituée par un poisson pélagique, Vinciguerria nimbaria. Cette zone, située hors des zones de production classiques liées à l’upwelling équatorial, présente une dynamique particulière, liée à un système d’instabilité d’ondes équatoriales. Ces ondes sont à l’origine de tourbillons susceptibles d’entraîner dans la couche euphotique des concentrations importantes de sels nutritifs et un développement particulier de la chaîne trophique. Pour tenter de comprendre l’origine de la concentration des thons, l’IRD (Institut de recherche pour le développement) a mis en place le programme Picolo (Production induite en zone de convergence par les ondes longues océaniques), destiné à étudier les caractéristiques de l’écosystème et du réseau trophique de la zone. Plusieurs campagnes ont eu lieu en saison de pêche et hors saison de pêche.
Dans cet article sont analysés l’alimentation sur des particules sestoniques et le métabolisme (respiration, excrétion ammoniacale) des copépodes planctoniques (qui représentent plus de 80% du zooplancton et près de 90% de la nourriture des V. nimbaria) et leur relation avec les fluctuations des phénomènes physiques.
2 Matériel et méthodes
Le travail présenté concerne le zooplancton récolté au cours de deux campagnes :
- • Picolo 1 (P1), en janvier–février 1997, caractérisée par une faible divergence au sud de 0°30N ;
- • Picolo 2 (P2), en juin–juillet 1997, caractérisée par une forte divergence et une onde d’instabilité tropicale très marquée, au nord de l’équateur.
L’échantillonnage et l’expérimentation ont été effectués à des stations séparées de 20 milles, situées le long de radiales nord–sud répétées, à 15°W (P1) et 20°W (P2).
La température et la salinité ont été enregistrées à l’aide d’une sonde CTD ; la chlorophylle a a été mesurée par fluorimétrie sur des échantillons d’eau prélevés à la rosette. Le zooplancton a été prélevé entre 0 et 100 m à l’aide d’un filet WP standard.
Les expériences d’ingestion et de métabolisme ont été effectuées à bord sur des copépodes (17 à 80 selon leur taille) triés immédiatement après la pêche et placés pendant 12 h dans des flacons d’incubation contenant de l’eau filtrée sur 0,2 μm pour les expériences de métabolisme et sur 60 μm pour les expériences de broutage. Après incubation, la teneur en oxygène des flacons est mesurée à l’aide d’une sonde YSI 58 et l’excrétion par la méthode de Koroleff. Le nombre et le volume des particules sont estimés à l’aide d’un compteur de particules. À l’issue de l’expérience, les copépodes sont déterminés et pesés. Les taux métaboliques et les taux d’ingestion sont calculés à partir des différences entre les flacons expérimentaux, contenant les animaux, et les flacons témoins, sans copépodes ; ils sont exprimés en μl O2 mg–1 h–1, μM mg–1 h–1 and μm3 mg–1 h–1 respectivement pour la respiration, l’excrétion et l’ingestion.
3 Résultats
Les températures de surface sont plus faibles pendant P2 que P1. Elles augmentent régulièrement de l’équateur (P1 : 26,2 °C ; P2 : 23,5 °C) à la convergence (P1 : 28,1 °C ; P2 : 26,3 °C à 4°N) mais diminuent légèrement entre 2°N et 3°N pendant P2. Les concentrations en chl a sont maximales à 2°N (maximum profond vers 60 m) pendant la campagne P1, et à proximité de la divergence pendant la campagne P2. Durant P1 et P2, les biomasses zooplanctoniques sont maximales à proximité de la divergence équatoriale et diminuent de façon marquée entre 0° et 1°N, puis entre 3° et 4°N. Aux mêmes latitudes, les biomasses zooplanctoniques sont toujours plus élevées pendant P2 que pendant P1. Undinula darwini (P1), Clausocalanus furcatus, C. arcuicornis et C. pergens (P2) sont les espèces les plus abondantes dans la plupart des échantillons, Eucalanus crassus, E. mucronatus, Rhincalanus nasutus, Macrosetella sp. et Miracia efferata, dans quelques échantillons.
Les taux d’ingestion sont toujours plus élevés à P2 qu’à P1. Pendant P1, ils sont maximums dans la zone du maximum profond de chl a. Les taux d’excrétion et d’ingestion varient dans le même sens pendant P1 mais pas pendant P2 au cours de laquelle l’ingestion augmente de 1°N à 4°N où elle est maximale, puis diminue fortement.
Au cours des deux campagnes, on note une relation entre l’ingestion et la concentration en particules sestoniques, avec, toutefois, une pente plus forte pendant P2 que pendant P1. L’analyse de corrélation multiple, réalisée entre les taux d’ingestion spécifiques (I), les concentrations en particules sestoniques (C), la taille des particules exprimée en diamètre équivalent sphérique (ESD) et le poids des individus (W) peut s’écrire sous la forme :
Il n’y a pas de relation entre les taux métaboliques et les concentrations ou les tailles des particules mais une relation linéaire entre la respiration et l’excrétion est observée. Au cours de P1, les taux spécifiques d’ingestion (en pourcentage de C corporel) sont faibles et souvent plus faibles que les taux respiratoires. Au cours de P2, au contraire, les taux d’ingestion sont plus élevés que les taux de respiration.
4 Discussion
L’absence de corrélation entre les variations moyennes de l’ingestion et du métabolisme, et celles de la chl a et de la biomasse zooplanctonique, témoignent d’un décalage temporel et/ou spatial pouvant être lié à la dynamique particulière de la zone. Toutefois, les taux d’ingestion les plus élevés ont été observés à la latitude du maximum profond de chlorophylle pendant P1 et dans la zone frontale pendant P2.
Comparés aux valeurs de la littérature, les taux d’ingestion sont assez faibles (de 1 à 8% du taux de carbone corporel). Cependant, ils sont plus élevés pendant la période d’instabilité (P2) que pendant la période stratifiée (P1), où ils sont insuffisants pour équilibrer les dépenses métaboliques.
La corrélation multiple entre les taux d’ingestion spécifiques, la concentration en particules, la taille des particules et le poids des copépodes montre le rôle positif évident de la concentration et de la taille des particules sur l’ingestion. La pente de la relation ingestion–concentration en seston est plus forte et l’ordonnée à l’origine plus élevée pendant la période d’instabilité que pendant la période de stabilité, ce qui montre que les taux d’ingestion, à concentration identique, sont plus forts pendant l’onde d’instabilité.
La taille des particules disponibles n’est pas significativement différente entre les deux saisons, mais les pics d’ingestion sur de grandes particules (15–20 μm) sont plus fréquents pendant l’été que l’hiver où l’ingestion sur les petites particules (3–6 μm) prévaut. Il est possible que les taux d’ingestion très faibles soient en partie liés à une mauvaise détection des très petites tailles (picophytoplancton) par le compteur de particules. Toutefois, l’absence de relation entre l’ingestion et la chlorophylle in situ ainsi que les faibles valeurs des rapports O/NH4-N suggèrent une ingestion sur des microhétérotrophes plutôt que sur du picophytoplancton.
L’ensemble de nos résultats suggère une chaîne trophique analogue pendant la période de stabilité et d’instabilité. Toutefois, pendant l’été boréal, l’enrichissement de la zone euphotique lié aux tourbillons et à l’advection des eaux de l’upwelling équatorial semble favoriser un recyclage rapide et une production accrue de cellules diversifiées de petites tailles accessibles aux microhétérotrophes. Ces résultats s’intègrent dans les récentes découvertes montrant l’importance de la boucle microbienne dans les eaux oligotrophes.