There is a growing concensus that loss of biological diversity is reaching crisis proportions [1,2]. The 1992 Convention on Biological Diversity (http://www.biodiv.org/convention/articles.asp) recognized this problem, and it provided a mandate to participating states to preserve their biodiversity. It also provided a mandate to scientists: to “Identify processes and categories of activities which have or are likely to have significant adverse impacts on the conservation and sustainable use of biological diversity” (Article 7c).
Which processes and human activities, then, are the most important causes of loss of biodiversity? Again, there is a general consensus among ecologists: “In general, the loss of biodiversity is caused by habitat loss” [3]; see also [4–6]. There is also little debate about the mechanism: “The effect of losing habitat is obvious: when habitat is lost from the landscape, the animals that are subsequently displaced may also be lost, ...” [7]. This consensus is reflected in the economic and applied literatures, which estimate species loss as a direct function of loss of forested habitat [8].
The evidence for this consensus comes in part from studies of species currently on red lists. Such studies cite many factors as contributors to species decline, but habitat loss is the most frequently cited factor [9,10]. When forests are fragmented by deforestation, smaller fragments typically have fewer species than large fragments [11,12]. And, clearly, when a species' habitat is completely destroyed, the species is unlikely to persist. As a popular t-shirt slogan put it: “When you cut down all the trees, the birdies have no place to sit”.
The implication of this argument is that, to protect biodiversity, one should protect habitat. This is the goal of legislation such as the Endangered Species Act in the United States, and the very recently enacted Species at Risk Act in Canada. It is also the strategy of many non-governmental organizations, which attempt to protect the habitat of endangered species, or sometimes more broadly, “endangered spaces” (e.g. the World Wildlife Fund, http://www.worldwildlife.org/global200/).
Yet, the logic of the argument above is faulty. The fact that imperiled species have often experienced habitat decline does not logically imply that reducing habitat loss will significantly reduce loss of biodiversity. Imperiled species have also experienced other aspects of global change: climate change, exposure to xenobiotics, etc. There is no evidence of which I am aware that habitat loss is the most important (as opposed to the most common) factor contributing to species decline. Further, not all species that have experienced habitat loss are imperiled, and areas of highly modified habitat may still maintain high diversity (e.g., the birds of the Netherlands [13]).
Thus, the appropriate scientific question is not: what processes are likely to have adverse affects on the conservation and sustainable use of biodiversity? The object of this question is mechanisms, which may or may not be relevant in practice. Rather, the correct scientific question is: species losses are most strongly associated with which characteristics of organisms' habitat? The object of this question is the dependent variable that was defined at the outset as being of concern: species decline.
I propose that much of the failure of current conservation biology to provide proactive solutions to the problem of imperiled species stems from a patently inappropriate model of the behavior of natural systems. Consider the following models.
1 A universe of processes
When the ancient Greek astronomers observed the motions of heavenly bodies, they proposed an entirely mechanical model of universe [14]. The universe, in essence, was a clockwork, with heavenly bodies attached to crystal spheres turning around the earth. The triumphs of science in the sixteenth to eighteenth centuries built upon this model of nature as a clockwork. Copernicus modified the heliocentric clockwork of the ancient Greeks and put the Sun at its center. Kepler proposed laws of planetary motion, describing the movement of individual planets around the Sun. In his studies of living organisms, Galileo represented their parts as series of pulleys and levers. Classical mechanics describes the interactions between pairs of bodies on frictionless planes. Optics describes light moving from one uniform substance to another. Basic chemistry describes the interactions among small sets of elements under defined conditions in test tubes.
Descartes, regarded by many as the founder of modern philosophy and much of modern science [15], described the laws of nature and the laws of mechanics as one and the same. In Descartes's view, the connection between cause and effect was direct and necessary. Causation is the push of one piece of the clockwork on another [16]. In this context, it makes sense to study processes – the interactions between elements of the clockwork.
Much of contemporary ecology is based on the same model of interacting cogs. Charles Elton described food webs. Bormann and Likens [17] describe nutrient cycling in forests as the transfer of nutrients among pools, where each step is mediated by a specific functional groups of organisms. They depict the removal of a group of organisms (e.g. cutting trees) as the shutting of a single valve. Chitty [18] describes the dynamics of small mammal populations very similarly, with the abundance of mammal depending upon growth rate, which depends upon food supply, which depends upon foraging rate, which depends upon snow cover, which depends upon , etc. In each case, nature is viewed as a set of discrete entities that interact with other entities through identifiable, namable processes, just as the gears and pulleys and escapements of a clockwork interact with each other and have identifiable functions. “Our traditions of analysis in theoretical and empirical ecology have been largely inherited from developments in classical physics and its applied variants” [19].
2 A universe of propensities
Yet, by the mid-twentieth century, it had become clear that nature does not necessarily behave like a clockwork for several reasons. First, many processes are simply not deterministic in nature. Certain phenomena are simply probabilistic, such as radioactive decay, spontaneous mutations, physical disturbances such as fires, etc. Heisenberg showed that certain subatomic phenomena are simply unknowable when viewed from the classical clockwork point of view. Nobel Prize winning chemist Kenichi Fukui showed that this also applies to many chemical reactions [20]. Heisenberg [21] pointed out that it no longer made sense to ask whether a theory could be developed to describe the processes occurring in particular experimental situations. Rather, he reversed the question: can only certain situations be observed in nature, as described by a mathematical formalism? Possible states of systems are simply expressed by probability functions.
Further, as ecologists readily agree, real ecosystems are complicated assemblages of many, many components, with many, many interactions, direct and indirect [22]. Even the most complicated experimental systems (e.g. [23,24]) have only a handful of components. Classical physics alone cannot get a rocket from the Earth to the Moon based upon underlying processes, because the interaction among three bodies is analytically intractable. Natural systems have innumerably more components. Moreover, the components are not static: biological systems have elements that evolve, new ones invade, some go extinct.
How, then, does one study complex natural systems? Karl Popper, arguably the pre-eminent epistemologist of the twentieth century, argued shortly before his death that nature in general does not act like a clockwork. Not only subatomic phenomena are indeterministic, macroscopic ones are as well. Except in isolated systems, strictly deterministic laws are not found. In the real world, nature shows “propensities”: probabilistic properties of the whole physical situation. Moreover, “these propensities, like Newtonian attractive forces, are invisible and, like them, they can act: they are actual, they are real” [16]. Propensities determine possible states of states of the universe in complex ways that cannot necessarily be explained mechanistically, any more than the behavior of subatomic particles can. Moreover, Popper realized that, when one experimentally isolates particular parts of natural systems, one excludes interactions that otherwise affect propensities. His examples were biochemical, but this is clearly even truer in ecological systems.
3 A methodology for applied ecology and conservation
The application of Popper's proposal to ecology suggests the following methodology:
- (1) Identify the properties of systems that are of genuine concern – species loss in this case.
- (2) Determine the propensities of those properties.
- (3) Test the robustness of those propensities, often by experimentally modifying them.
- (4) Investigate hypothesized mechanisms as a means of identifying new propensities.
4 Example: imperiled species in Canada
What environmental characteristics are associated with species endangerment? Several studies have examined “hot spots” of endangered species with a view to designating areas of conservation interest [25–29]. However, it is not obvious whether areas with high concentrations of endangered species are good (because local conditions have allowed rare species to persist) or bad (because local conditions have led to species becoming endangered).
Mackey and Currie (in review; also [30]) asked a different question: what is the difference between places where imperiled species used to occur, but occur no longer, versus places where those imperiled species persist today. They examined the distributions of species designated by the Committee on the Status of Endangered Wildlife in Canada as extirpated, endangered, threatened or vulnerable in Canada (collectively, “imperiled species”). Of these, they identified 62 species of terrestrial vertebrates for which historical and current spatial distributions could be reconstructed. They calculated numbers of species lost in each of the 217 ecoregions (regions of similar physical, climatic and biotic characteristics: [31,32]) that cover Canada.
Mackey and Currie hypothesized that these losses might depend upon: (1) the amount of habitat lost (as postulated by the literature at large), (2) human population density, (3) rates of use of agricultural pesticides (as postulated, arguably, by [33]) and (4) the numbers of species present in the region (because where there are more species present, more can be lost). They found that the number of imperiled species lost per ecoregion was weakly related to the remaining area of natural (versus human-dominated) habitat. In contrast, the number of species lost was strongly related to both the area of agricultural land and the area of land treated with pesticides (Table 1). Multiple regressions showed that species losses were related to the use of pesticides even after statistically controlling for the amount of agricultural land, but not vice versa.
Simple Pearson correlations between the numbers of species of imperiled birds and mammals and habitat characteristics. Extracted from Mackey and Currie (in review)
Birds | Mammals | |
Total species richness | 0.70 | 0.63 |
Natural area | −0.16 | −0.22 |
Area of agricultural land | 0.77 | 0.71 |
Area treated with herbicides | 0.80 | 0.70 |
Human population density | 0.62 | 0.56 |
This result shows propensity, versus process. The process of species loss as a result of habitat loss is well understood, yet the propensities of species loss are not consistent with the hypothesis that habitat loss is the major driver of loss of imperiled species in Canada.
5 Example: patterns of species richness and habitat loss in southern Ontario
Two centuries ago, southern Ontario, Canada, was covered mainly by wetlands and forest. Subsequent agricultural and urban development was intense in the southwest, moderate in the southeast, and light in areas that were topographically complex, or situated on poor soils (e.g. south-central Ontario; Fig. 1). Thus, a natural gradient of conversion of forested landscapes to human-dominated landscapes exists.

Landcover in southern Ontario, Canada, as determined by AVHRR (advance very high resolution radiometry) satellite imagery. Landcover has been aggregated into agriculture, forested and urban categories.
If habitat loss were the primary cause of species loss, then there should be a strong propensity for there to be fewer species in areas with less remaining natural habitat. To test this hypothesis, Muller [34] tallied the number of species of breeding birds in 100 km2 quadrats covering the area, based on the five year censuses reported in the Atlas of Breeding Birds of Ontario [35]. He also used AVHRR satellite images to determine the proportion of each quadrat that is forested. Muller found that there was very little relationship between breeding bird species richness and forest cover (Fig. 2). There was similarly little relationship between the richness of any particular guild (e.g., guilds based on habitat preference such forest-interior birds, guilds based on migratory status, dietary preference, etc.). Muller's study examined only presence/absence, not abundance, so it is impossible to say anything about the abundance based on his work. However, the propensity for bird species to be present is virtually unrelated to remaining natural cover. We are now testing whether these patterns of species richness are related to patterns of pesticide use. Our initial results (D. Currie and L. Pépin, unpublished data) suggest that this may be the case.
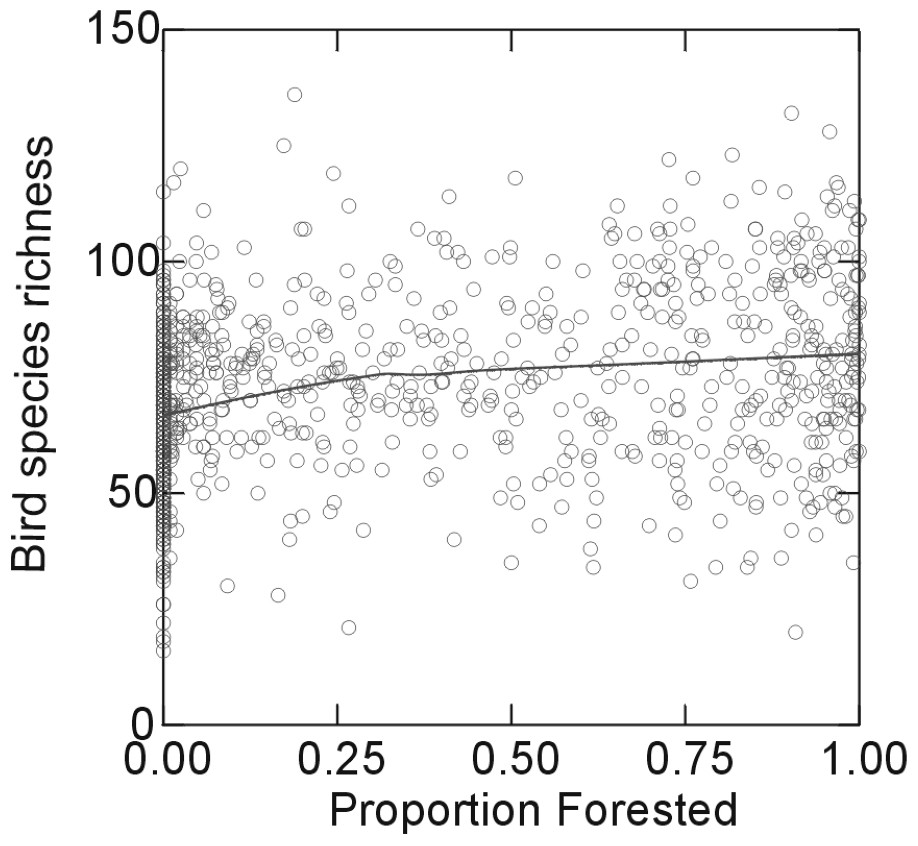
The relationship between the number of species of breeding birds in 100 km2 quadrats covering southern Ontario and the percentage of forest cover in the quadrats.
6 Conclusion
“Predictive power [is] the strongest evidence that the natural sciences have an objective grip on reality” [36]. Conservation biology has based its principle strategy on the premise that habitat loss is the most serious threat to species persistence. Unquestionably, habitat loss can be a cause of species loss. However, patterns of species loss cannot be predicted from patterns of habitat loss, at least in the case of Canada. This is not consistent with the hypothesis that habitat loss has been the major cause of species loss. Rather, recently observed propensities of species loss suggest that, as Rachel Carson warned forty years ago, pesticide use, or agricultural practices associated with it, may be the main culprit. The next steps to test this hypothesis further would be to determine if the propensities holds in other areas where gradients of pesticide use exist, and the observe whether changes in the use of pesticides change the frequency of species loss.