1 Introduction
Germ cells are potentially immortal, since they provide the link between all generations. The fusion between eggs and sperm gives rise not only to a new individual, but also, theoretically at least, to an endless series of generations. Since germ cells are totipotent, they also exhibit unique properties [1]. Thus, analysis of the germ cell lineage is highly relevant for the elucidation of the uniqueness of pluripotent stem cells.
An egg or oocyte is probably the most complex and extraordinary cell, because this single cell can potentially develop into a whole organism. William Harvey was the first to recognise this in 1651, when he claimed that ‘all things come from an egg’, although it was nearly 200 years later that Bischoff and Van Beneden first observed fertilisation. Harvey thought that development of the embryos and foetus from an egg occurred gradually. Thus, Harvey thought of development in terms of epigenesis, which is a gradual emergence of the embryo and foetus from an egg, rather then endorsing the concept of preformation. Waddington later depicted this concept in his famous ‘epigenetic landscape’, in a symbolic representation of the developmental potential of an egg. Epigenesis is thus the formation of entirely new structures during the development of the embryo. A relatively recent concept of epigenetics refers to the study of mitotically or meiotically heritable changes in gene function that cannot be explained by changes in DNA sequences. Indeed, the derivation of all differentiated cell types from pluripotent stem cells occurs without changes in DNA sequences, thus emphasising the importance of epigenetics in these processes.
2 Mammalian germ line cycle and parthenogenesis
The germ cell cycle is repeated in every generation (Fig. 1), which also shows the relationship between early development and derivation of pluripotent embryonic stem cells (ES) and pluripotent embryonic germ cells (EG), from blastocysts and primordial germ cells, respectively. Fully mature gametes or sperm and egg may be considered as the end-products of the germ cell cycle. The oocyte (Fig. 2) contains critical information necessary for development from this single cell into an adult [1]. The oocyte contributes genetic information in the form of approximately 20,000 genes, which constitute the genetic blueprint for generating the organism. There are also maternally inherited factors that are synthesised and stored during the maturation of the oocyte, and play an essential role in regulating early development. Finally, there is epigenetic information, which defines the maternal origin of the oocyte genome in mammals, which is distinct from the paternal epigenetic information contributed by the sperm.
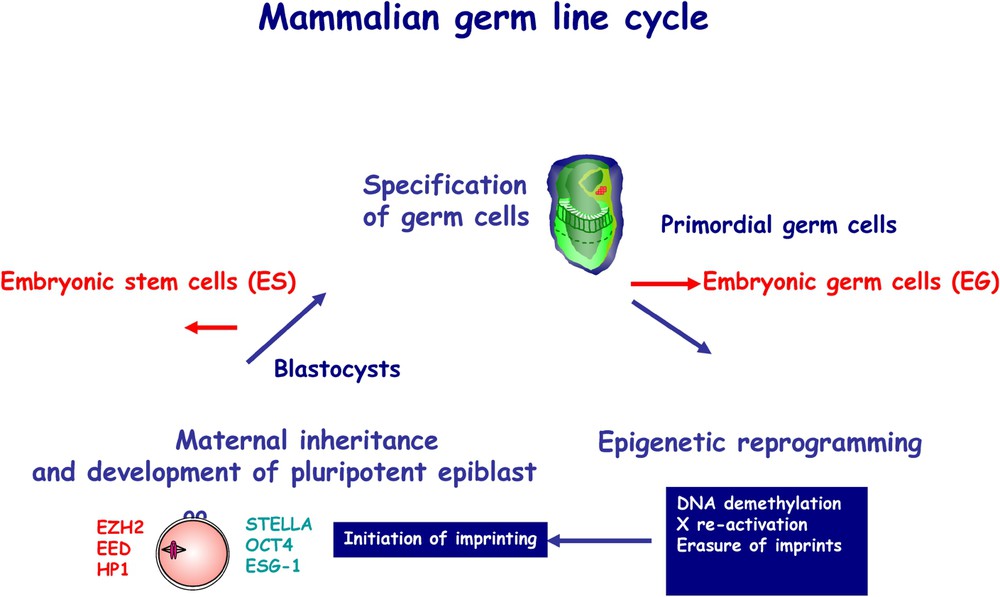
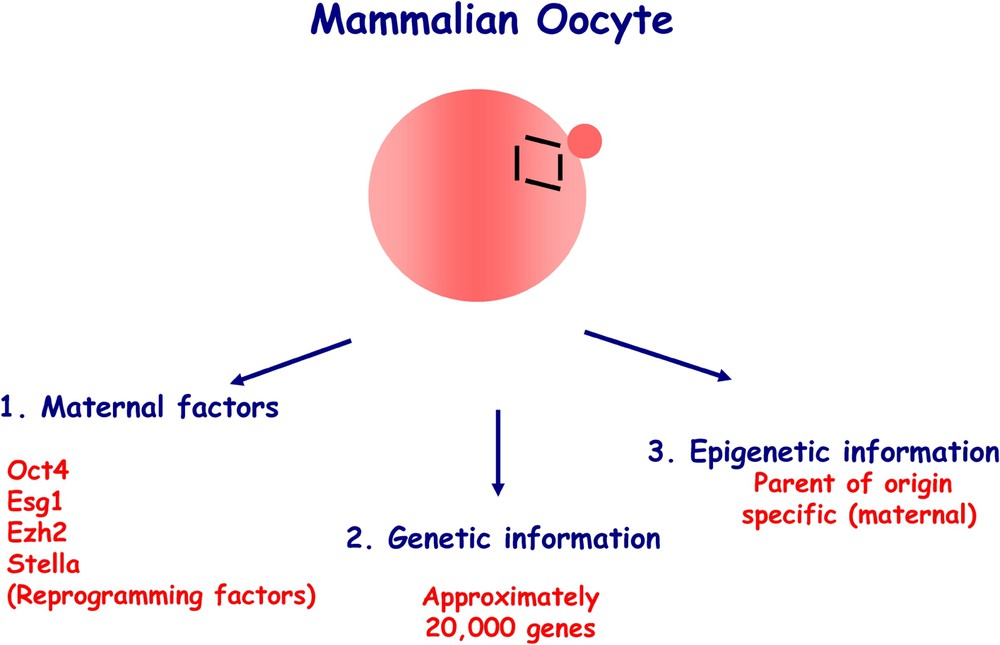
Since the oocyte seems to contain the necessary information for development, there have been many studies to determine the development potential of this cell in the absence of fertilisation. Such development is referred to as parthenogenesis. Richard Owen first used this term in 1849 to describe “procreation without the immediate influence of a male”. His work on frogs showed that artificially activated eggs in the absence of any paternal contribution could develop into tadpoles and adults, although at a low frequency. Mouse eggs can also be similarly activated artificially, for example, by simply incubating them in an alcohol-containing medium. Such parthenogenetic mouse eggs can develop at a high frequency to the preimplantation blastocyst stage, consisting of about 40–60 cells, a stage from which pluripotent ES cells are normally derived. If transplanted into recipient mothers, parthenogenetic embryos can develop to an advanced 25-somite stage, but they never reach adulthood [2]. However, when parthenogenetic embryos are combined with normal embryos to make chimeras, parthenogenetic cells can contribute to many tissues in adult animals [3]. It was intriguing why parthenogenetic embryos failed to develop to term. Further investigations into this question led to the discovery of the phenomenon of genomic imprinting, suggesting that parental genomes are functionally non-equivalent, and therefore that both are required for development to term [4–6].
3 Genomic imprinting
Using micromanipulation techniques, it is possible to make zygotes consisting of two maternal genomes called gynogenones (which are similar to parthenogenones), and zygotes that contain two paternal genomes, called androgenones. Neither of these two types of zygotes can develop to term. More importantly, at the most advanced stage of their respective postimplantation development at midgestation, they exhibit distinct and opposite characteristics. The former has better embryos and the latter, better development of placental tissues. This observation suggested that the parental genomes are functionally non-equivalent, despite the fact that they have equivalent genetic information [6,7]. The term ‘genomic imprinting’ is used in this context to describe the functional differences between parental genomes during mammalian development, and both are crucial for development to term. Much work has been carried out to look at the mechanism of genomic imprinting. From these studies, it emerges that there are a number of genes whose expression is strictly dependent on their parental origin. Some are expressed only when inherited from the father and others are expressed only when inherited from the mother [7]. A major growth factor, the insulin-like growth factor 2 (Igf2), for example, is only expressed when inherited from the father and, therefore, this gene is repressed in parthenogenetic embryos. Currently, approximately 50–100 imprinted genes have been identified in the mouse and human genomes. The activity of these genes explains the necessity for both sets of parental genomes for full development in mice, and probably in all mammals.
Since both parthenogenones (or gynogenones) and androgenones have the potential to develop to the blastocyst stage, it has been possible to derive pluripotent Es cells from them. While they have many of the attributes of normal pluripotent ES cells, they show differences in their potential to differentiate into a variety of somatic cell types. Parthenogenetic ES cells have a greater potential for differentiation into neuronal cells, while androgenetic ES cells can differentiate more readily into mesodermal tissues, such as skeletal muscles.
4 The origin of the germ cell lineage
Eggs and sperm are the products of the germ cell lineage. It is important to consider the origin of this lineage during development. Although the establishment of germ cell lineage is one of the fundamental necessities in all living organisms, it is striking that the mechanism by which this is achieved is not conserved amongst different model organisms. Broadly speaking, there are two major ways used to establish the germ cell lineage. The first mechanism is referred to as preformistic, where only those cells that eventually form the founder germ cells inherit the germ cell determinants that are already present in the egg. The second mechanism involves a stem cell model, where development first leads to the establishment of pluripotent stem cells. These cells then respond to signalling molecules to acquire germ cell competence, and eventually germ cell fate. In both cases, however, the germ cell lineage is amongst the first to be established during development. A key requirement for germ cell specification is to have a mechanism that prevents germ cells from acquiring somatic cell fate. Thus, repression of the somatic cell fate is a key event during germ cell specification, which involves epigenetic mechanism for repression of genes that confer somatic cell fate on neighbouring cells [7,8].
In mice, germ cell competence is induced in pluripotent proximal epiblast cells of the early postimplantation embryos [9,10]. Signalling molecules, such as BMP4, are critical for conferring germ cell competence on pluripotent epiblast cells. Germ cells are specified from amongst these competent cells by mechanisms that remain to be fully elucidated. The neighbouring cells that share common ancestry acquire somatic cell fate. Germ cells continue to express some of the key genes associated with pluripotency, particularly Oct4. However, germ cells do not behave as pluripotent ES cells. Unlike ES cells, which can differentiate into all the different cell types when introduced into blastocysts, germ cells fail to respond in any way at all, and they eventually die without undergoing differentiation. It would be informative to know why germ cells are refractory to differentiation under these conditions.
5 Programming the germ cell lineage
Following the establishment of the germ cell lineage, there follows an extensive reprogramming of the genome [1,11]. This process involves a cycle erasure and re-initiation of epigenetic information, which may be essential for restoring totipotency to this lineage. Some aspects of the mechanism involved at this time may be important for understanding many aspects of how epigenetic information can be removed and re-introduced, which affects the properties of the cells. Such a mechanism presumably also operates during differentiation of diverse cell types from pluripotent stem cells. Germ cells also subsequently inherit epigenetic information concerning their parental origin. Finally, during oocyte development, there is inheritance of maternal factors that are synthesised and stored in a fully mature oocyte. These factors play a critical role during early development. Amongst them must also be molecules that have the potential to restore totipotency to a somatic nucleus when transplanted into the oocyte. Since fusion between somatic cells and stem cells also results in the restoration of pluripotency in the somatic nucleus of hybrid cells, it follows that stem cells must also contain key factors that are capable of genomic reprogramming [1].
6 Germ cells and pluripotent stem cells
There are two aspects of particular interest when considering the relationship between germ cells and pluripotent stem cells. The first is the observation that germ cells under specific conditions in culture can change and acquire properties similar to those of pluripotent ES cells. These cells generated from primordial germ cells are referred to as embryonic germ cells (EG). Pluripotent ES and EG cells are very similar, but they are not identical [1]. Nevertheless, EG cells can differentiate into all the different cell types, as seen with ES cells. The mechanism, which leads to the development of pluripotent stem cells from the highly specialised germ cells, the precursors of sperm and eggs, is unknown, but an understanding of this process would add to knowledge concerning the pluripotent state.
It is also known that pluripotent stem cells express certain key genes to maintain this state. Apart from Oct4, there is Nanog, which is essential for the retention of pluripotency. It is interesting to note that the gene Nanog is closely linked in both mouse and humans genomes to a gene called stella, which is expressed specifically in founder germ cells in mice [12]. It will be informative to explore the reasons for this close linkage between those genes that show expression in pluripotent stem cells and germ cells.
7 From pluripotent stem cells to primordial germ cells, sperm and oocytes
Recent work has shown that it is possible to derive primordial germ cells as well as sperm and eggs from pluripotent mouse ES cells [12–15]. Sperm cells generated from ES cells undergo meiosis [13]. When injected into the oocyte, these spermatogenic cells derived from ES cells apparently participated in the development at least up to the blastocyst stage. In another set of experiments, it was found that it is also possible to generate oocytes in culture from pluripotent ES cells. These oocytes were able to activate and develop spontaneously into blastocysts [14]. This is the first demonstration that ES cells can develop into early embryos via the development of eggs.
While the generation of germ cells from stem cells is still a relatively unrefined process, further work may improve the efficiency of this process. Such a system would be of great benefit if human pluripotent ES cells could be used to generate human germ cells. This would allow detailed studies of the human germ cell lineage, which is currently difficult if not impossible, and consequently, we know relatively little about the human germ cell lineage. If it becomes possible to generate human oocytes from the existing human ES cells, this would also allow detailed investigations on the properties of human eggs. In addition, such oocytes generated from ES cultures could be used to study genomic reprogramming following transplantation of somatic nuclei. Recent studies have shown that it is possible to generate pluripotent stem cells from somatic cells following transplantation of somatic nuclei into human oocytes. This procedure may be possible to carry out using human oocytes generated in culture from human ES cells. If so, it would allow generation of additional lines of human ES cells, thus providing a larger repertoire of these cells than currently available. Furthermore, human ES cells may also be generated by this procedure using somatic cells from patients. Following their differentiation into, for example, cardiac or neural cells, they can be used for transplantation to alleviate diseases. More importantly, it may also become possible to generate ES cells from various patients with complex diseases. These cells could provide a means to study how various disease states arise, which may serve to develop therapeutic agents to alleviate many human diseases [16].
8 Conclusion
An investigation of the germ cell lineage is important for a variety of reasons. Firstly, these studies are informative concerning the pluripotent state and, therefore, they will help to increase our knowledge of pluripotent stem cells. Germ cell lineage has many unique properties including extensive epigenetic reprogramming of the genome. A greater understanding of the underlying mechanism may allow development of methods to restore pluripotency to somatic cells without the use of oocytes. Alternatively, it may become possible to generate human germ cells from the existing human ES cells. This will have a significant impact on our understanding of the human germ cell lineage. It may also pave the way towards the development of human oocytes from human ES cells. Recent studies showed that it is possible to derive human ES cells from somatic cells following somatic nuclear transplantation into human oocytes [17]. If oocytes generated from ES cells could be used for this purpose, it would obviate the need for oocyte donors. More importantly, such oocytes would facilitate generation of a larger repertoire of human pluripotent ES cells through somatic nuclear transplantation. These cells would have the potential for use in cell therapy to alleviate various diseases. In addition, they could also be used to study the mechanisms underlying complex human diseases, and for the development of therapeutic agents to combat such diseases. The latter could turn out be the most significant use of advances in stem cell research in human medicine [16].