1 Introduction
Piper (P.) nigrum L. is the first economically important species in the genus Piper in the Piperaceae family [1]. Besides its use in food substances, P. nigrum L. has well-reputed medicinal importance due to the presence of biologically active piperine and other compounds [2,3].
P. nigrum plants are conventionally propagated through stem cuttings, but this traditional method could not prove efficacious to multiply the plant proportionately to the escalating demand. Lower seed viability is another limiting factor in getting higher biomass production of healthy plants. For the last few years, a substantial degree of decline (from 35 to 27 million tons) has been noticed in its production due to several factors, including pathogens like mycoplasma, bacteria, virus, fungus, pests, and poor crop management practices [2,4]. To overcome these limiting factors, modern techniques of propagation such as in vitro regeneration are required, with modified factors (photoperiod) to enhance the production of this important species. Tissue culture techniques are widely used to produce healthier biomass from a single plant in a short span of time and provide opportunities for germplasm conservation and for regeneration of plants from somatic tissues bypassing the sexual barrier, and may result in genetically stable and useful genotypes [5].
Periodic application of light to in vitro cultures is one of the important steps that might modify the regeneration potential in many elite plant species. Light factors have a direct relationship with chlorophyll content and any change in light intensity makes the internal pathways fluctuate. Sometimes, light fluctuation enhances the production of secondary metabolites [6–8]. The effect of reverse photoperiods on explant response, callus and regeneration in P. nigrum are not reported yet. However, the effect of photoperiod on embryogenic calli, protoplast regeneration, shoots induction, in vitro tuberization and flower formation in Oryza sativa, Solanum pennellii, melon, tobacco and Xanthosoma sagittifolium are widely reported [9–13].
Naturally, plants accumulate and release different active metabolites, including essential amino acids [14]. Some of these amino acids are called “essential”; human beings intake these amino acids directly from plants or plant-based products which are further organized into proteins, lipoproteins, glycoprotein, macromolecules, oxidized into urea and CO2 as energy sources. During incomplete metabolism in the body, free radicals are released in large quantities, which initiate a chain reaction and ultimately damage the cells or tissues [15]. Different plant species release active defense compounds such as phenols, an active member of natural antioxidants [16]. Hence, plant-based antioxidants are a hunter of toxic free radicals, chain breakers and scavengers of singlet O2 formation such as ROS, which mutate and inhibit DNA molecule, proteins and lipids [17].
Plant-based products need qualitative and quantitative data analysis for acceptance, and worldwide recognition for safety and efficiency to meet the general criteria of food products and medicine. High performance liquid chromatography (HPLC) is a trustworthy technique for detection and resolution of active metabolites [18]. Most of the HPLC studies had been focused on the field plants and fruits of P. nigrum L. We established a reliable and simple HPLC method for the determination of piperine contents in regenerated tissues of P. nigrum L.
The main objective of this study was to investigate the effect of reverse photoperiods on healthy biomass production, piperine production, amino acid biosynthesis, total phenolic, flavonoid content and antioxidant activities (DPPH, ABTS, PoMo, chelating activity on Fe2+) in P. nigrum L. for industrial applications. These in vitro tissues accumulate and release high-quality, safe, effective, and clean desirable metabolites in optimum quantities, which are free from microbial contaminants and have a positive impact on different industries. Micropropagation under reverse photoperiod is a novel approach for healthy biomass and biologically active metabolites production like piperine for commercial usage.
2 Materials and methods
2.1 Seed germination
The seed was germinated on Murashige and Skoog (MS) basal medium for selection of explant. Seeds were surface decontaminated according to the modified method of Abbasi et al. [4]. Ethanol (75%) and mercuric chloride (2%) were used as surface sterilants for 2 min followed by three-fold washing with double autoclaved distilled H2O. Seeds were carefully dried inside a laminar flow bench and placed on an MS-basal medium with or without plant growth regulators at 32 °C for 2–8 weeks.
2.2 In vitro cultures
Seed-derived plants are used as explants for overall in vitro culture establishment. We used the modified method of Ahmad et al. [2] for surface sterilization. Uncut leaves were placed in a mercuric chloride (HgCl2; 0.05%) and ethanol solution (70%; v/v) for less than 1 min. Treated leaves were then washed 3–4 times with autoclaved double-distilled H2O. For in vitro culture establishment, the MS-medium was selected as the basic medium and fortified with 30 g/L sucrose (MERK), 8 g/L agar (SIGMA) and different concentrations of PGRs (auxins, cytokinins and TDZ) and finally the pH was adjusted (∼ 5.8) by using weak HCl or weak NaOH. All media were autoclaved at 121 °C for 20 min and stored under controlled conditions. Leaf portions of reasonable size were used for callus induction under reverse photoperiod (16hD/8hD). For callus induction and shoot regeneration, the MS-basal medium was augmented with BA alone (0.5–2.0 mg L−1) or in combination with various concentrations of gibberellic acid (GA3) or BA with 2,4-dichlorophenoxyacetic acid (2,4-D) and α-naphthalene acetic acid (NAA), IBA (indole butyric acid) alone in various concentrations or in combination with NAA and IAA (indole acetic acid) or GA3 with or without BA and various concentrations of TDZ (0.5–4.0 mg L−1). The MS-medium without PGRs (MS0) was used as a control for the whole regeneration under normal (16hL/8hD) and reverse photoperiod (16hD/8hL). After 4 weeks of explant culturing, data was collected for callus induction (%). A little green callus was shifted into a new medium, fortified with similar PGRs for regeneration and the data on different parameters were taken after 4 weeks under reverse photoperiod. Elongated shoots with mature stems were transferred into rooting medium augmented with different concentrations (0.5–2.0 mg L−1) of IBA alone or with NAA (0.25–1.5 mg L−1) and IAA, or NAA with BA and 2,4-D. After complete rhizogenesis, the medium particles were carefully removed, washed several times with autoclaved double-distilled water, and transferred to soil, sand and manure (2:1:1) mixture for further growth.
2.3 Amino acid analysis
In vitro cultures (callus, in vitro-grown shoots, plantlets and peppercorn) of P. nigrum L. were analyzed for amino acids accumulation. Fresh samples were dried at 45 °C for 24 consecutive hours and then powdered, from which 0.5 g was vortexed with 5 mL (0.1%) HCl for 2 min and finally exposed to centrifugation (10,000 rpm) for 15 min. The supernatant was collected and 2–3 mL were filtered through a 0.45-μm membrane filter. The amino acid-containing solution was first diluted five times (0.2 mL to 1.0 mL), and 20-μL samples of each tissue were then injected into an amino acid analyzer. A system used for amino acid analysis consists of a sample pretreatment oven (CTO-20AC) with a temperature ranging from 60 to 80 °C at maximum. Data acquisition includes sampling frequency (2 Hz), start time (0) and end time (72 min). The pump consists of pump mode (binary gradient), pump A (LC-20AD), pump B (LC-20AD), total flow (0.5 mL/min), press-Max (110 kgf/cm2), solenoid A (FCV-11AL), solenoid valve pump A (A–A–A), solenoid B (FCV-11AL). The detector contains an RF-10AXL (model), response (1.5 seconds), excitation wavelength (350 nm), emission wavelength (450 nm), gain (×4) and recording range (1).
2.4 Total phenolic and flavonoid contents determination
The total phenolic contents (TPC) were determined using the method of Singleton and Rossi [19]; the flavonoid content was determined by using the method of Kosar et al. [17]. Two hundred microliters of each concentration were added to 10 mL of Folin–Ciocalteu's reagent (1:10). The mixture was mixed with 7 mL of Na2CO3 (0.115 mg/mL), incubated (2 h), and absorbance was taken at 765 nm. Gallic acid (1.0–10 mg/mL) was used for the calibration curve. The results were expressed as Gallic acid equivalent (GAE) mg/g of dried concentration. The flavonoids content was expressed as rutin equivalent (RE) mg/g-DW of extracts. In vitro culture extracts in methanol (1 mL) and AlCl3 in ethanol were mixed and diluted to 25 mL with pure ethanol. Blank samples also contained 1 mL of in vitro culture extract with a single drop of CH3COOH and diluted to 25 mL. A similar procedure was used for rutin calibration curve. The absorbance of these samples was checked at 20 °C after an incubation period of 40 min.
2.5 Antioxidant activities
The antioxidant (DPPH-free radical scavenging) activity was carried out according to the method of Ahmad et al. [2]; 1.0 mL of the ethanolic tissue extract (5 mg/20 mL) was mixed carefully with 2.0 mL of DPPH solution (0.159 mg/20 mL × 4). After incubation (30 min) in the dark, the absorbance of the solution was measured at 517 nm at room temperature. The phosphomolybdenum (PoMo) activity was determined according to the methods of Khan et al. [20]. Each sample was mixed with sulfuric acid (600 mM), ammonium molybdate (4 mM) and sodium phosphate (28 mM) in diluted methanol. After mixing, these samples were incubated for 90 min at 90 °C. The absorbance of each green complex was checked at 695 nm.
ABTS radical cation-antioxidant activity was determined by using the recent method of Khan et al. [20]. Briefly, free radicals are produced by treating ABTS (7.4 mM) with potassium persulfate (2.45 mM). First, 3.0 mL of reagent were poured into the cuvette and the absorbance was checked at 414 nm. Pure ethanol without reagent was run as a blank. Three different concentrations of each tissue extracts were used for ABTS radical cation-antioxidant activity. Accurately, 100 μL of each sample was shifted into the cuvette containing the reagent. The mixture was kept for incubation (90 min) and the absorbance was measured at 414 nm on an UV spectrophotometer.
The chelating activity on Fe2+ was investigated according to the current method of Khan et al. [20]. The reactions of each extract with ferrozine for iron (II) ions were assessed in free solution. Three extract concentrations (2.5 mL) were mixed with 0.05 mL of a solution of FeCl2·4 H2O (2 mM). For the initiation of the reaction, 0.2 mL of 5 mM ferrozine was added and incubated for 10 min at room temperature with periodic shaking. The absorbance of sample concentrations was measured at 562 nm along with that of a blank solution using similar FeCl2 and water. For positive control, EDTA was applied and samples without the addition of the extract or EDTA were used as negative controls.
The following equation was used for the estimation of antioxidant activities:
2.6 Samples preparation for High Performance Liquid Chromatography (HPLC)
Piperine detection and determination in different P. nigrum L. tissues were investigated according to the method of Liu et al. [18]. A Soxhlet system was used for extraction; the final extract was filtered through a 45-μm membrane for High Performance Liquid Chromatography (HPLC) analysis. HPLC equipment (Shimadzu Lc8A, Japan) set with a variable wavelength (λ) detector (UV; 254 nm), a solvent vacuum degasser, a binary pump, an injection (10 μL) and a C18 column (25 cm), a mobile phase (methanol; 75: buffer; 25) with a flow rate of 1.5 mL per min. 10 μL of standard piperine (25 mg) were injected in the HPLC device for retention time determination and chromatogram development. Similarly, 10-μL samples were injected and compared with the retention time of piperine (Fig. 1).
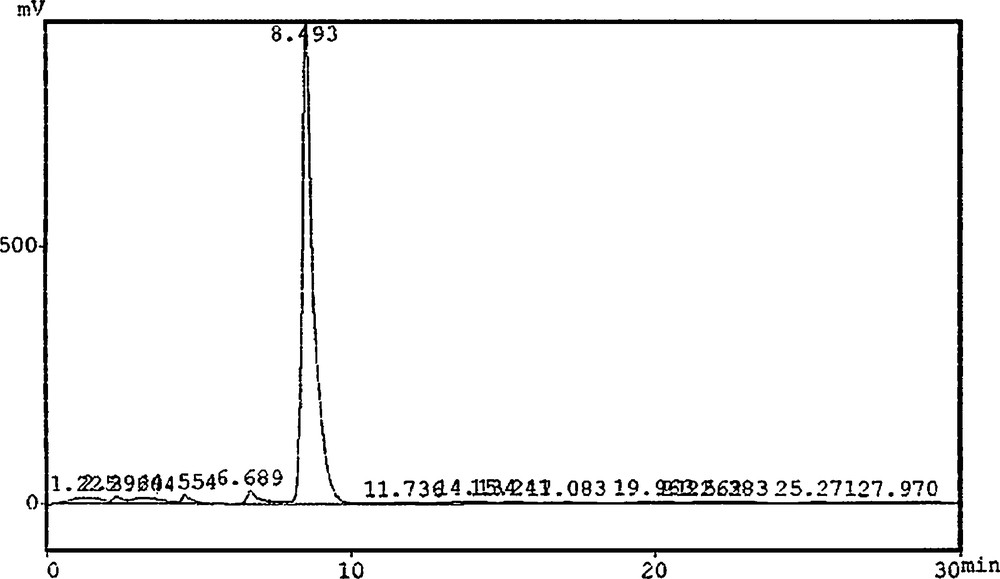
Chromatogram of standard piperine.
2.7 Statistical analysis
Five or six explants were incubated in a 100-mL Erlenmeyer flask, containing approximately 25 to 35 mL of the medium. For each treatment, we used 27 flasks and the data for percent callus induction and the data for different parameters of organogenesis were taken from three independent experiments. Statistical analysis of mean values with standard deviation (±) and least significant difference (LSD) at probability level P < 0.05 were done by using Statistix software (8.1). For graphical presentation, Origin Lab software (8) was used.
3 Results and discussion
3.1 Micropropagation
All PGRs used in this study enhanced callus induction and organogenesis under reverse photoperiod (Fig. 2). The effect of various PGRs under the influence of reverse photoperiods such as BA with 2,4-D (0.25–1.5 mg L−1) and NAA or various concentrations of BA alone or 1.0 mg L−1 constant BA in combination with GA3 or GA3 alone, or IBA alone or in combination with either IAA (0.25–1.5 mg L−1) or NAA, and different concentrations of TDZ on indirect organogenesis were evaluated (Fig. 2). The callus induction response was observed to be 100% under reverse photoperiod, when the MS-medium was incorporated with either 1.5 mg L−1 BA or BA (1.0 mg L−1) in combination with 0.5 mg L−1 of GA3 (Fig. 2). However, the maximum callus response of 95% was recorded for 4.0 mg L−1 of TDZ under the effect of a 16hD/8hL photoperiod. It has been observed that 1.0 mg L−1 of BA alone or 1.0 mg L−1 of BA and GA3, and 3.0 mg L−1 of TDZ induced an 85% callus induction from leaf explants in P. nigrum under a similar photoperiod. Presently, 0.5 mg L−1 of IBA in combination with IAA and NAA (0.25 mg L−1) or 1.0 mg L−1 IBA along with 0.5 mg L−1 IAA and NAA produced an 80% callus response. Specific reports on the effect of reverse photoperiod on callus induction in Piper species are not available in the cited literature, but the effect of similar photoperiod on embryogenic calli was reported in other plant species [9]. However, Ahmad et al. [3] in the previous report observed a lower callus response under the influence of similar PGRs and normal photoperiod (16hL/8hD). Abbasi et al. [5] reported consistent results evidencing that BA in combination with NAA produced maximum callus in Brassica rapa species. Madhusudhanan and Rahiman [21] reported that the addition of activated charcoal in the medium induced callus but failed to produce regenerants from leaf explants of P. nigrum.
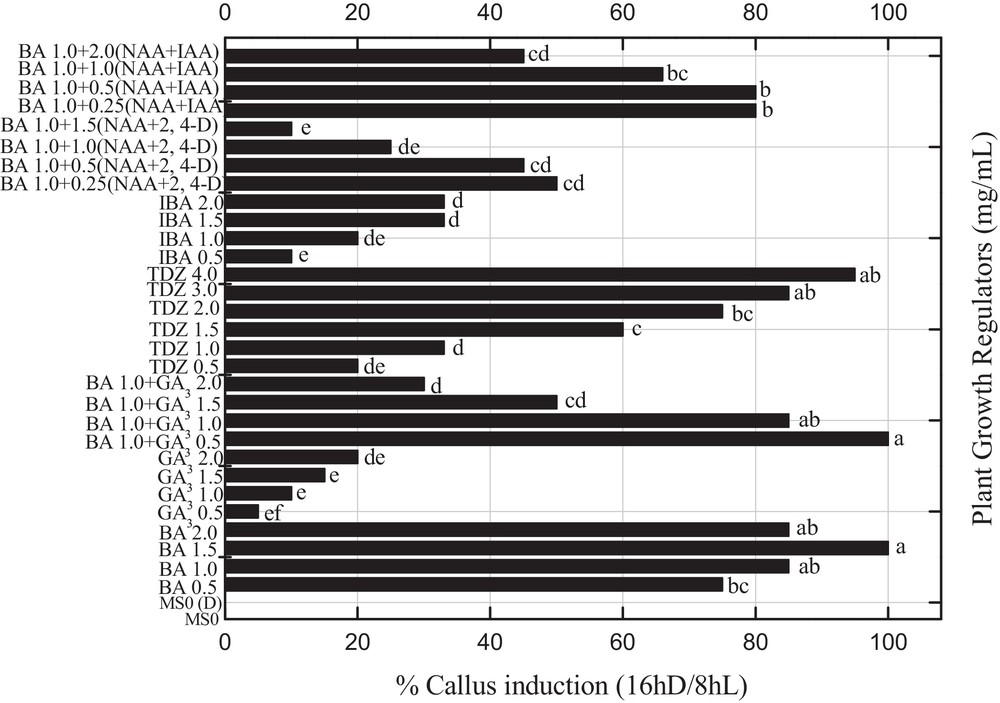
Effects of various concentrations of 6-benzyladenine with 2,4 dichlorophenoxyacetic acid (0.25-1.5 mg L−1) and α-naphthalene acetic acid or various concentrations of 6-benzyladenine alone or 1.0 mg L−1 constant 6-benzyladenine in combination with gibberellic acid or gibberellic acid alone, indole butyric acid alone in different concentration or in combination with indole acetic acid (0.25–1.5 mg L−1) and α-naphthalene acetic acid, and different concentrations of thidiazuron on the percent callus induction in Piper nigrum from seed-derived explants under reverse photoperiod. Data were collected after 4 weeks of culture. Values are means of three replicates. Columns with common letters are not significantly different at P < 0.05.
A mature callus was transferred into shoot organogenesis medium and the data on shoot organogenesis was determined after 30 days of sub-culturing under the influence of reverse photoperiods. Maximum shoot induction was observed for two concentrations (1.5 and 2.0 mg L−1) of indole butyric acid (IBA), which produced a more than 80% shooting response with a mean shoot length of 3.73 cm and 6.67 shoots per explant, which is significantly (P < 0.05) similar to 0.5 mg L−1 of TDZ (Fig. 3; Table 1). Sharma et al. [22] and more recently Kumar and Reddy [23] also reported that TDZ significantly enhance the regeneration efficiency in Jatropha curcas. All other combinations of PGRs were less effective under the influence of dark incubation to produce shooting response. In the previous experiment, Ahmad et al. [3] reported a maximum shooting response by using a similar set of PGRs and normal photoperiod. The elongated shoots collected from shooting media were transferred into the rooting medium. An optimum percent rooting (95%) was recorded for 1.5 mg L−1 IBA with mean root length (< 6.0 cm) and number of roots/plantlet (5.0) under reverse photoperiod (Table 2). Moreover, greater than 80% rooting was recorded for 2.0 mg L−1 IBA or IBA in various combinations with NAA and IAA. A similar root induction response was also reported by Sharma et al. [22] and Thiyagarajan and Venkatachalam [24] by using IBA, IAA, and NAA. Conversely, Philip et al. [25] obtained rooted plantlets from shoot tip cultures of P. nigrum by using 1 mg L−1 of NAA under normal photoperiod. In another study on other Piper spp., rooting was achieved on the IAA induced medium [26]. In our experiments, we obtained healthy rooting by using different PGRs. It has been concluded from the present studies that reverse photoperiods influence rooting response and callus induction as compared to normal photoperiods. P. nigrum is acclimatization friendly, therefore can easily fit in both pot and field soil. Rooted plantlets were successfully transferred into pots, which were maintained in controlled conditions, for further growth.
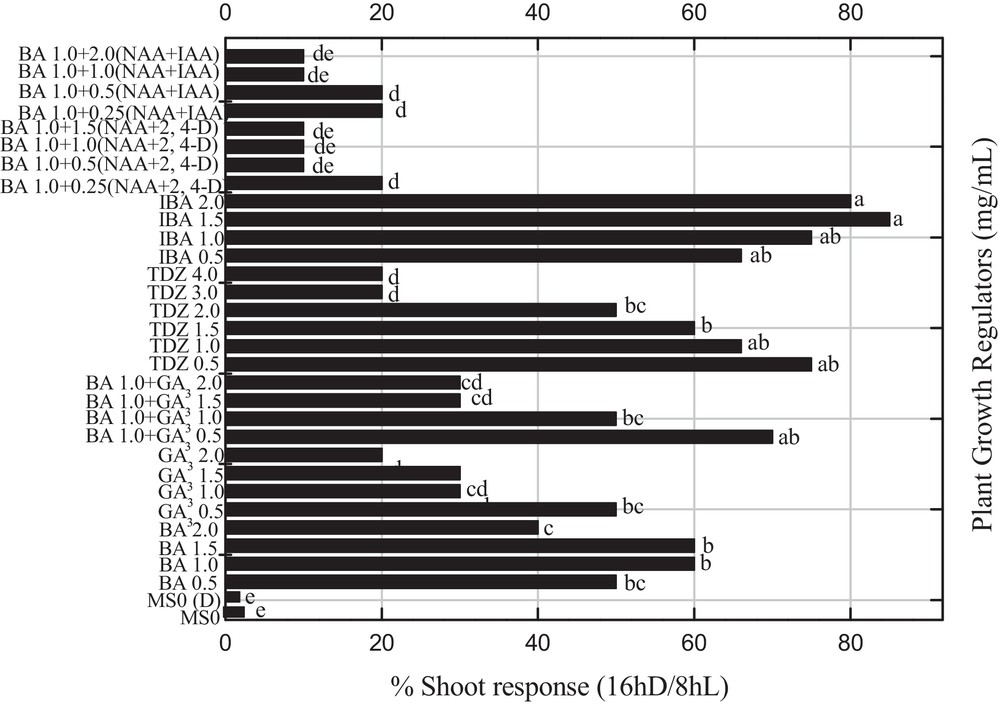
Effects of various concentrations and combination of 6-benzyladenine, 2,4 dichlorophenoxyacetic acid, α-naphthalene acetic acid, gibberellic acid, indole acetic acid, α-naphthalene acetic acid and thidiazuron on the percent shoot induction in Piper nigrum under the influence of reverse photoperiod from leaf explants of seed-derived plants. Data were collected after 4 weeks of culture. Values are means of three replicates. Columns with common letters are not significantly different at P < 0.05.
Effect of various concentrations of BA along with 2,4-D and NAA or BA alone or BA in combination with GA3 or GA3 alone, IBA alone or IAA and NAA, and different concentrations of TDZ on shoot organogenesis in P. nigrum.
Shoot organogenesis | |||||
S. No | MS + PGRs (mg−1) | Mean shoot length (cm) Mean ± SD |
No. of shoots/explant Mean ± SD |
Min. SL (cm) | Max. SL (cm) |
1 | BAP (0.5) | 1.58 ± 0.63a,b,c,d* | 2.83 ± 1.04b,c,d,e* | 1.00 | 2.25 |
2 | BAP (1.0) | 2.22 ± 1.10a,b,c,d | 6.83 ± 1.61a | 1.00 | 3.15 |
3 | BAP (1.5) | 2.33 ± 2.31a,b,c,d | 5.93 ± 3.52a,b | 1.00 | 5.00 |
4 | BAP (2.0) | 2.23 ± 2.40a,b,c,d | 1.33 ± 0.58d,e | 0.70 | 5.00 |
5 | GA3 (0.5) | 2.23 ± 1.37a,b,c,d | 1.50 ± 0.50d,e | 1.00 | 3.70 |
6 | GA3 (1.0) | 0.67 ± 0.29d | 1.00 ± 0.00d,e | 0.50 | 1.00 |
7 | GA3 (1.5) | 1.53 ± 1.29a,b,c,d | 1.00 ± 0.00d,e | 0.60 | 3.00 |
8 | GA3 (2.0) | 1.27 ± 0.67c,d | 1.00 ± 0.00d,e | 0.70 | 2.00 |
9 | BAP (1.0) + GA3 | 3.33 ± 3.21a,b,c | 5.17 ± 2.47a,b,c | 1.00 | 7.00 |
10 | BAP (1.0) + GA3 | 0.80 ± 0.10d | 1.50 ± 0.50d,e | 0.70 | 0.90 |
11 | BAP (1.0) + GA3 | 0.90 ± 0.10d | 1.67 ± 0.29c,d,e | 0.80 | 1.00 |
12 | BAP (1.0) + GA3 | 0.80 ± 0.00d | 1.17 ± 0.29d,e | 0.80 | 0.80 |
13 | TDZ (0.5) | 3.50 ± 3.91a,b | 6.47 ± 4.64a,b | 1.00 | 8.00 |
14 | TDZ (1.0) | 1.77 ± 1.94a,b,c,d | 4.33 ± 4.16a,b,c,d,e | 0.50 | 4.00 |
15 | TDZ (1.5) | 1.90 ± 1.25a,b,c,d | 3.33 ± 4.04a,b,c,d,e | 0.60 | 3.10 |
16 | TDZ (2.0) | 1.03 ± 0.68d | 2.27 ± 1.55c,d,e | 0.50 | 1.80 |
17 | TDZ (3.0) | 0.87 ± 0.32d | 1.00 ± 0.00d,e | 0.50 | 1.10 |
18 | TDZ (4.0) | 0.83 ± 0.38d | 1.00 ± 0.00d,e | 0.40 | 1.10 |
19 | IBA (0.5) | 1.47 ± 0.80b,c,d | 4.00 ± 3.61a,b,c,d,e | 0.70 | 2.30 |
20 | IBA (1.0) | 2.40 ± 0.95a,b,c,d | 4.33 ± 4.04a,b,c,d,e | 1.80 | 3.50 |
21 | IBA (1.5) | 3.73 ± 4.00a | 6.67 ± 5.69a | 0.70 | 8.00 |
22 | IBA (2.0) | 1.40 ± 0.46b,c,d | 4.53 ± 3.93a,b,c,d | 1.00 | 1.90 |
23 | BAP (1.0) + NAA + 2,4-D (0.25) | 0.83 ± 0.15d | 1.17 ± 0.29d,e | 0.70 | 1.00 |
24 | BAP (1.0) + NAA + 2,4-D (0.5) | 0.80 ± 0.17d | 1.00 ± 0.20d,e | 0.70 | 1.00 |
25 | BAP (1.0) + NAA + 2,4-D (1.0) | 0.67 ± 0.15d | 0.83 ± 0.29e | 0.50 | 0.80 |
26 | BAP (1.0) + NAA + 2,4-D (1.5) | 1.33 ± 0.58b,c,d | 0.92 ± 0.32d,e | 1.00 | 2.00 |
27 | IBA (0.5) + IAA + NAA (0.25) | 0.90 ± 0.10d | 1.02 ± 0.26d,e | 1.00 | 2.00 |
28 | IBA (1.0) + IAA + NAA (0.5) | 0.73 ± 0.12d | 1.00 ± 0.00d,e | 0.80 | 1.00 |
29 | IBA (1.5) + IAA + NAA (1.0) | 1.13 ± 0.51c,d | 1.27 ± 0.64d,e | 0.60 | 0.80 |
30 | IBA (2.0) + IAA + NAA (2.0) | 0.73 ± 0.25d | 1.00 ± 0.00d,e | 0.70 | 1.00 |
31 | MS0 (dark) | 0.80 ± 0.20d | 1.00 ± 0.00d,e | 0.50 | 1.00 |
32 | MS0 | 0.93 ± 0.06d | 1.00 ± 0.00d,e | 0.90 | 1.00 |
Effect of different concentrations (0.5–2.0 mg L−1) of IBA alone or IBA with different concentration of NAA (0.25–1.5 mg L−1) and IAA, BA with NAA and 2,4-D (0.25, 0.5, 1.0 and 1.5 mg L−1) on root organogenesis in P. nigrum L.
Root organogenesis | |||||
MS + PGRs (mg L−1) | Rooting (%) Mean ± SD |
Mean root length (cm) Mean ± SD |
No. of roots/plantlet Mean ± SD |
Min. RL (cm) | Max. RL (cm) |
IBA (0.5) | 55 ± 1.0e* | 2.02 ± 1.08b,c* | 2.00 ± 1.00b,c | 1.00 | 3.16 |
IBA (1.0) | 78 ± 2.0c | 4.47 ± 3.21a,b | 3.82 ± 2.37a,b | 1.00 | 7.32 |
IBA (1.5) | 95 ± 3.0a | 6.60 ± 5.46a | 5.00 ± 2.67a | 2.90 | 10.00 |
IBA (2.0) | 85 ± 3.0b | 4.63 ± 3.83a,b | 3.07 ± 1.42a,b,c | 2.89 | 8.00 |
BAP (1.0) + NAA + 2,4-D (0.25) | 10 ± 6.0g | 0.63 ± 0.32c | 1.23 ± 0.25c | 0.40 | 1.00 |
BAP (1.0) + NAA + 2,4-D (0.5) | 8 ± 4.0g | 0.90 ± 0.17c | 1.00 ± 0.00c | 0.80 | 1.10 |
BAP (1.0) + NAA + 2,4-D (1.0) | 10 ± 6.0g | 0.70 ± 0.20c | 1.23 ± 0.23c | 0.50 | 0.90 |
BAP (1.0) + NAA + 2,4-D (1.5) | 7 ± 4.0g | 4.00 ± 3.00a,b,c | 2.66 ± 0.99b,c | 2.00 | 7.00 |
IBA (0.5) + IAA + NAA (0.25) | 80 ± 4.0b,c | 3.87 ± 2.31a,b,c | 3.00 ± 1.32a,b,c | 2.20 | 6.50 |
IBA (1.0) + IAA + NAA (0.5) | 80 ± 3.0b,c | 2.60 ± 2.14b,c | 2.27 ± 1.36b,c | 0.89 | 5.00 |
IBA (1.5) + IAA + NAA (1.0) | 66 ± 1.0d | 1.50 ± 1.32b,c | 1.94 ± 0.87b,c | 0.43 | 2.97 |
IBA (2.0) + IAA + NAA (2.0) | 45 ± 2.0f | 1.23 ± 0.81b,c | 2.03 ± 0.12b,c | 0.50 | 2.10 |
MS0 (dark) | 0g | 0d | 0d | 0 | 0 |
MS0 | 0g | 0d | 0d | 0 | 0 |
3.2 Amino acid analysis
In current experiment, callus, in vitro-grown shoots, plantlets and peppercorn were investigated for amino acid biosynthesis. Callus accumulated THR in higher quantities (C4H9NO3 = 3.715%) than SER (C3H7NO3 = 3.574%) and ARG (C6H14N4O2 = 2.092%) under light photoperiod (Table 3). Callus culture under a 16hD/8hL photoperiod also showed higher accumulations of THR (2.0%), SER (2.4%), LEU (1.0%), and ARG (1.007%), but in lower quantities than tissues incubated under light photoperiod. In vitro-grown shoots showed the best potential for THR (C4H9NO3 = 4.978%) accumulation. Similarly like callus culture, shoots also accumulated suitable amounts of SER (C3H7NO3 = 3.154%) under light photoperiod (Table 3). However, regenerated shoots accumulated THR (2.33%), SER (1.98%), lysine (LYS; 1.01%) and ARG (1.11%) under reverse photoperiod (16hD/8hL), but in lower amounts than regenerated shoots incubated under a 16hL/8hD photoperiod. In vitro-grown plantlets showed the best potential for THR (C4H9NO3 = 3.022%) and SER (C3H7NO3 = 2.704%) accumulation under 16hL/8hD (Table 3). But in vitro plantlets incubated under reverse photoperiod showed lower accumulation of THR (1.22%), SER (1.0%) and TYR (1.09%) than under normal photoperiods. Peppercorn showed the lowest potential for amino acids accumulation but ARG (C6H14N4O2 = 4.494%) production was higher than other in vitro cultures (Table 3). It has been concluded from these results that amino acids accumulation and biosynthesis are correlated with photosynthetic activity. Higher accumulation of amino acids was observed in regenerated tissues incubated under light photoperiod. This is the first report on amino acid biosynthesis in P. nigrum L. in vitro cultures.
Amino acid biosynthesis in different regenerated tissues and peppercorn of P. nigrum L. incubated under reverse and light photoperiods.
Amino acids | 16hL/8hD photoperiod | 16hD/8hL photoperiod | ||||||
Callus (%) | Shoots (%) | Plantlets (%) | Fruits (%) | Callus (%) | Shoots (%) | Plantlets (%) | Fruits (%) | |
ASP | 0.452d* | 0.617c,d | 0.287e | 0.139d | 0.222d | 0.33c | 0.069c | 0.09d |
THR | 3.715a | 4.978a | 3.022a | 0.541c,d | 2.0a,b | 2.33a | 1.22a | 0.21d |
SER | 3.574a | 3.154a,b | 2.704a,b | 0.615c,d | 2.40a | 1.98a,b | 1.01a,b | 0.29d |
GLU | 0.215d | 00.00 | 0.395e | 0.052d | 0.11d | 0.133c | 0.09c | 0.055d |
PRO | 00.00 | 0.208d | 0.161e | 00.00 | 0.11d | 0.122c | 0.09c | 0.04d |
VAL | 0.062d | 00.00 | 0.046e | 0.393d | 0.08d | 0.055c | 0.104c | 0.19d |
MET | 0.108d | 0.380d | 0.316e | 0.344d | 0.90c | 0.123c | 0.19c | 0.18d |
ILE | 0.135d | 0.266d | 0.732d | 0.324d | 0.91c | 0.098c | 0.40b,c | 0.15d |
LEU | 1.530b,c | 0.652c,d | 1.871c | 0.696c,d | 1.00c | 0.33c | 0.60b | 0.40cd |
TYR | 1.162c | 0.118d | 2.224b | 1.474c | 0.98c | 0.091c | 1.09a,b | 1.10c |
PHE | 0.303d | 0.290d | 0.072e | 0.022d | 0.23d | 0.111c | 0.00c | 0.04d |
HIS | 0.332d | 0.376d | 0.183e | 0.073d | 0.19d | 0.201c | 0.077c | 0.055d |
LYS | 0.176d | 1.573b,c | 0.828d | 3.136a | 0.09d | 1.001b | 0.20c | 1.99b |
AMO | 0.593c,d | 0.546c,d | 0.234e | 1.136c | 0.28d | 0.288c | 0.20c | 1.088c |
ARG | 2.092b | 2.249b | 0.201e | 4.494a | 1.00c | 1.111b | 0.09c | 2.786a |
GLY | 0.265d | 0.508c,d | 00.00 | 0.076d | 0.18d | 0.277c | 0.02c | 0.044d |
ALA | 0.462d | 0.110d | 00.00 | 0.095d | 0.24d | 0.095c | 0.045c | 0.065d |
3.3 Determination of total phenolic and flavonoid content
In this experiment, TPC and flavonoids were investigated in regenerated tissues of P. nigrum incubated under 16hD/8hL and 16hL/8hD photoperiods (Table 4). TPC was determined in different concentrations (1.0–10 mg) of each tissue extract. Callus under light photoperiod produced higher TPC (1.86–9.91 mg/g-DW) than cultures incubated under reverse photoperiod (1.6–9.8 mg/g-DW). Similarly, a maximum flavonoid content (7.38 mg/g-DW) was observed when callus was incubated under normal photoperiod. However, callus incubated under reverse photoperiod showed a lower production of flavonoid (6.3 mg/g-DW). A maximum TPC (1.13–7.16 mg/g-DW) was observed in regenerated shoots incubated under normal rather than reverse photoperiod (0.9–6.7 mg/g-DW), but it was lower than callus cultures. It shows that light fluctuations not only disturb photosynthetic activity, but also play a role in the regulation of biochemical processes. Similarly, a higher flavonoids content (6.3 mg/g-DW) was observed in regenerated shoots incubated under normal photoperiod than under reverse photoperiod (4.63 mg/g-DW). Plantlets showed a lower production of TP content under normal (1.79–5.05 mg/g-DW) and reverse photoperiod (4.5 mg/g-DW) than callus and regenerated shoots. Like TPC, plantlets showed a lower production of flavonoid content under normal and reverse photoperiods (4.89 and 4.64 mg/g-DW), as shown in Table 4. Specific reports on the production of TPC and flavonoids in P. nigrum L. in vitro cultures are not available, but different scientists reported similar total phenolic contents and flavonoids in in vitro cultures of other plants using different extraction solvents [27,28]. Naz et al. [29] reported higher amount of TPC in callus cultures of Cicer arietinum, and Kokotkiewicz et al. [30] recently reported the production of TPC in Cyclopia subternata Vogel in vitro cultures.
Total phenolic (TP) and flavonoid content in callus, in vitro-grown shoots and plantlets of P. nigrum L., after the application of reverse and light photoperiods in comparison with gallic acid (GAE) and rutin (RE), expressed in mg/g-DW.
In vitro cultures | Incubation | Spectrophotometric results | |||||
Total phenolic contents | Flavonoids | ||||||
(A) | (B) | (C) | (D) | (E) | (F) | ||
Callus | 16hD/8hL | 1.86 ± 0.3a* | 5.87 ± 1.20a | 7.88 ± 0.04a | 9.69 ± 1.6a | 9.91 ± 0.3a | 7.38 ± 2.5a |
In vitro shoots | 16hD/8hL | 1.13 ± 0.89a | 2.96 ± 2.19b | 5.11 ± 1.08b | 6.37 ± 1.6b | 7.16 ± 1.3b | 6.3 ± 2.4a,b |
In vitro plantlets | 16hD/8hL | 1.79 ± 2.03a | 2.33 ± 0.91b | 2.84 ± 1.89c | 3.49 ± 2.1c | 5.05 ± 2.9c | 4.89 ± 1.7b |
Callus | 16hL/8hD | 1.60 ± 0.81a | 2.90 ± 0.36b | 7.30 ± 0.09a | 9.50 ± 0.4a | 9.80 ± 0.3a | 6.3 ± 0.3a,b |
In vitro shoots | 16hL/8hD | 0.90 ± 0.44b | 1.33 ± 0.14c | 2.90 ± 0.38c | 5.1 ± 0.3b,c | 6.7 ± 0.9b,c | 4.63 ± 0.3b |
In vitro plantlets | 16hL/8hD | 1.40 ± 0.65a | 1.60 ± 0.36c | 1.70 ± 0.08d | 1.80 ± 0.5d | 4.50 ± 0.7c | 4.64 ± 0.2b |
3.4 Antioxidant activities
Higher DRSA (DPPH radical scavenging activity; 56%) was observed in callus culture incubated under normal photoperiod than 16hD/8hL photoperiod (18%; Table 5). But regenerated shoots obtained from callus cultures showed higher DRSA (92.8%) if incubated under 16hD/8hL than if incubated under 16hL/8hD (91%), which is in agreement with the observations of Pérez-Tortosa et al. [31]. This shows that when undifferentiated cells enter the differentiation phase, they produce higher active defense compounds under stress conditions. After the development of roots, the plantlets showed higher DRSA (85%; 16hD/8hL) than plantlets incubated under light (16hL/8hD) photoperiod (Table 5). However, the commercial peppercorn, purchased from the local market, showed a 72% activity, which is lower than in vitro shoots and plantlets, but higher than callus cultures. The fluctuation in data may be due to the fractional distribution or explant types and culture conditions. These in vitro cultures can be used as a natural source of antioxidants.
Antioxidant activities in 100, 250 and 500 μg concentrations of P. nigrum L. in vitro culture extracts incubated under reverse/light photoperiod. The activity was determined by using 1,1-diphenyl-2-picrylhydrazyl (DPPH), phosphomolybdenum (PoMo), ABTS and Fe2+ as free radicals.
Plant tissues | Callus | In vitro shoots | In vitro plantlets | Peppercorn |
% DPPH (16hD/8hL) |
||||
(A) | 08 ± 0.382c* | 83 ± 2.084b | 36.7 ± 1.15b | 33 ± 0.143c |
(B) | 12 ± 0.710b | 90 ± 5.440a,b | 37.3 ± 1.05b | |
(C) | 18 ± 1.566a | 92.8 ± 3.29a | 57 ± 2.760a | |
% DPPH (16hL/8hD) |
53 ± 0.987b | |||
(A) | 53 ± 0.658a | 87 ± 0.560b | 36 ± 0.585c | 72 ± 0.897a |
(B) | 54 ± 0.878a | 89 ± 0.878b | 56 ± 0.097b | |
(C) | 56 ± 0.975a | 91 ± 0.219a | 85 ± 0.365a | |
% PoMo (16hD/8hL) |
||||
(A) | 07 ± 0.566c | 07 ± 2.330c | 12 ± 3.349b | 23 ± 0.782c |
(B) | 23 ± 2.770b | 15 ± 1.109b | 12 ± 2.966b | |
(C) | 37 ± 1.943a | 18 ± 0.966a | 21 ± 2.966a | |
% PoMo (16hL/8hD) |
33 ± 0.450b | |||
(A) | 49 ± 0.120c | 29 ± 0.120c | 47 ± 0.180b | 62 ± 0.198a |
(B) | 63 ± 0.230b | 33 ± 0.730b | 38 ± 0.340c | |
(C) | 78 ± 0.089a | 48 ± 0.054a | 52 ± 0.391a | |
% ABTS (16hD/8hL) |
||||
(A) | 45 ± 4.780c | 62 ± 0.856c | 71 ± 5.380c | 18 ± 0.437c |
(B) | 65 ± 1.779b | 78 ± 2.598b | 79 ± 1.476b | |
(C) | 73 ± 0.854a | 86 ± 1.213a | 87 ± 3.653a | |
% ABTS (16hL/8hD) |
23 ± 0.870b | |||
(A) | 32 ± 0.730c | 28 ± 0.732c | 63 ± 0.980c | 44 ± 0.973a |
(B) | 49 ± 0.420b | 59 ± 0.721b | 71 ± 0.230b | |
(C) | 63 ± 0.120a | 73 ± 0.320a | 85 ± 0.345a | |
% Fe+2 (16hD/8hL) |
||||
(A) | 17 ± 3.398c | 29 ± 0.743c | 61 ± 0.298c | 08 ± 0.7431c |
(B) | 32 ± 2.750b | 41 ± 3.700b | 69 ± 1.094b | |
(C) | 44 ± 4.221a | 54 ± 1.822a | 77 ± 0.846a | |
% Fe+2 (16hL/8hD) |
13 ± 0.1874b | |||
(A) | 12 ± 0.543c | 18 ± 0.673c | 43 ± 0.9781c | 24 ± 0.4537a |
(B) | 29 ± 0.742b | 39 ± 0.752b | 51 ± 0.2536b | |
(C) | 43 ± 0.232a | 53 ± 0.342a | 65 ± 0.7540a |
Higher PoMo-AA (phosphomolybdenum antioxidant activity) of 78% was observed in higher concentration (500 μg) of the callus culture extract when incubated under normal (16hL/8hD) than when incubated under reverse photoperiod (16hD/8hL; 37%) as shown in Table 5. The PoMo-A activity was also found higher in regenerated shoots and plantlets (48% and 52%) at higher concentrations (500 μg) under normal photoperiod than under reverse photoperiod (18% and 21%). The current data are in agreement with the results of Grzegorczyk et al. [32] for Salvia officinalis L. Lower concentrations of each tissue showed lower activities. However, 62% of PoMo-AA was recorded in peppercorn.
In this study, callus exhibited higher ABTS activity (73%) in higher concentration (500 μg) under reverse photoperiod than normal photoperiod (63%). Similarly, in vitro shoots also showed higher activity (86%) under reverse photoperiod than normal (73%). During complete organogenesis, again higher activity (87%) was observed in plantlets incubated under reverse photoperiod than normal photoperiod (85%) as shown in Table 5. Peppercorn showed lower ABTS activity (44%) than callus, in vitro shoots and plantlets. The current studies are in agreement with the results of Pérez-Tortosa et al. [31] that in vitro-grown shoots of Thymus membranaceus possess potent ABTS activity as compared to wild plant.
Callus culture extract exhibited higher Fe2+ chelating-based antioxidant activity (44%) when incubated under reverse photoperiod. Significantly, a similar activity was observed in callus culture induced under light photoperiod. Regenerated shoots collected from reverse photoperiod showed slightly higher activity (54%) than light photoperiod (53%). Moreover, in vitro plantlets showed elevated activity (77%) incubated under reverse photoperiod as compared to light incubation (65%). Peppercorn showed lower Fe2+ chelating-based antioxidant activity (24%) than regenerated tissues (Table 5).
3.5 Piperine determination through HPLC
In this study, in vitro-grown shoots produced 1.57 mg/g-DW piperine contents (Fig. 4). In vitro plantlets of P. nigrum L. showed a slightly higher amount of piperine (1.889 mg/g-DW) production than in vitro shoots. In the previous report, Ahmad et al. [3] reported higher piperine production in different tissues incubated under normal photoperiod. Interestingly, pepper berries showed 10 times more piperine (11.42 mg/g-DW) production than in vitro shoots and plantlets. The active principle “piperine” was selected as reference compound for fingerprint development in P. nigrum L. in vitro cultures. Physiologically active piperine production in these cultures showed an ascending variation from shoot culture to plantlets development and peppercorn maturation. These findings can support the use of chromatographic analysis in authentication of in vitro cultures of P. nigrum L. and also for other valuable plant species. For quality control and chromatographic fingerprint analysis, a simple HPLC protocol is summarized along with the required parameters including injection volume, flow rate, mobile phase, column and detector types.
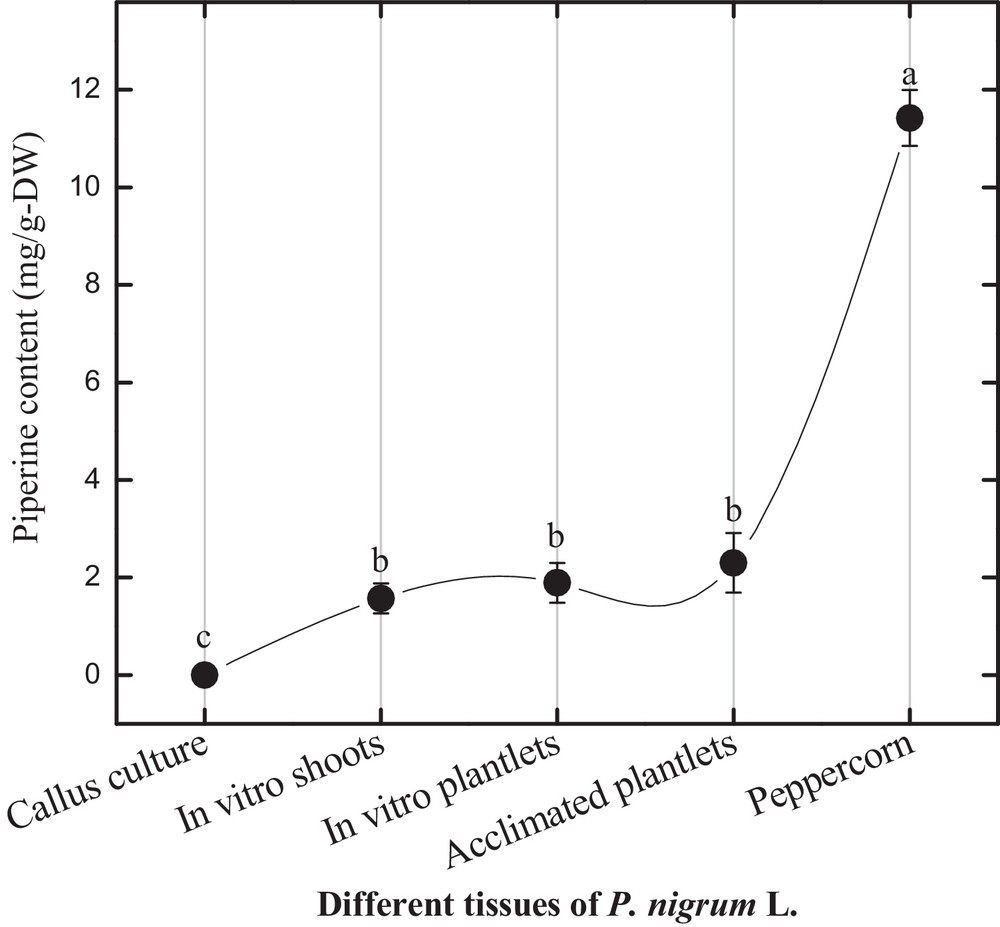
Piperine content in different tissues of Piper nigrum L.
4 Conclusion
In this experiment, we have studied the effect of reverse photoperiod on different growth phases and consistent production of biologically active compounds under in vitro conditions. We have concluded that reverse photoperiod is a good approach for callus induction and root development. Such approaches can be further used for consistent production of piperine in bioreactor for commercial applications. Quality control in large-scale in vitro cultures of medicinal plants plays a significant role in ensuring the safety and efficacy of the raw materials before it is converted to the final product. Fingerprint analysis has been introduced and accepted by World Health Organization (WHO) as a strategy for the assessment of plant products. Quality control for in vitro cultures of medicinal plants focuses mainly on bioactive components, while fingerprinting offers an integral characterization of a complex system with a quantitative degree of reliability. Therefore, these results may ensure a better understanding of active metabolites production for application in food and pharmaceutical industries.
Disclosure of interest
The authors declare that they have no conflicts of interest concerning this article.
Acknowledgements
We are thankful to Higher Education Commission (HEC, NRPU) of Pakistan for financial support to complete this research work.