1 Introduction
The Colorado potato beetle (Leptinotarsa decemlineata Say, CPB) is the major defoliator of potato throughout the northern hemisphere [1–5]. Both larvae and adults feed on plants in the Solanaceae family (Solanales, Solanaceae), including potato (Solanum tuberosum L.), tomato (S. lycopersicum L.), eggplant (S. melongena L.) and nightshade (S. nigrum L.), causing damage to fields and financial losses to farmers [6,7]. The beetle was native of Mexico and southeastern USA [2], spread rapidly throughout North America, Europe, and parts of Asia [8], and invaded China in the 1990s from Kazakhstan. From then on, the CPB has spread eastward and is presently distributed in most of the northern Xinjiang Uygur Autonomous Region [9]. Foliage damage caused by the CPB is a severe threat to potato crops in northern Xinjiang. Unlike in the other areas, the CPB only feed on potato crops in this region [10]. The beetles can eat almost all of the leaves of potato plants, which seriously affect photosynthesis. It is well-known that potato is a typical C3 plant and that the Calvin cycle only occurs in the mesophyll cells, so there are not enough synthetic organic compounds to maintain the normal growth, development and reproduction. Every year, CPB could cause up to 50% harvest reduction, or no harvest at all in some fields. Many pest management methods, such as insecticides, biological control, crop rotation, traditional breeding, and space isolation have been used in this region to reduce the damage to potato crops and the spreading speed of the CPB. So far, because of environmental conditions, labor and other limiting factors, spray pesticides are the most effective way in CPB control. However, there are still many problems with them. On the one hand, as a kind of alien species, the CPB has few nature enemies in this area. On the other hand, the CPB has always adapted and developed resistance to the insecticides [1,9]. Moreover, the third-instar larvae pupate in the soil and back to the ground as fourth-instar larvae to complete their life cycle. So, the insecticides do not inhibit CPB raging. Meanwhile, long-term use of insecticides causes much serious harm to ecology [11,12]. An environmentally sound strategy for the integrated pest management of important agronomic insect pests might be to take advantage of host–plant resistance [13].
Bacillus thuringiensis (Bt) is the most important viable biological agent for the control of CPB [14,15], the insecticidal activity on which depend insecticidal proteins secreted during plant growth (vegetative insecticidal proteins, called Vip proteins), as well as parasporal crystalline protoxins (Cry and Cyt proteins) [16–18]. Cry toxins have been successfully used as conventional sprayable bioinsecticides or engineered in transgenic plants [19,20]. Once ingested by susceptible insects, Cry proteins would have been proteolytically activated by gut proteases and the active toxin would have bound to receptors located on the brush border midgut epithelium to exert their toxicity, and finally broken down the gut epithelium of insects, leading to their death [21]. A pore formation mechanism is involved in the target membrane or an alternative cell death process involving the adenylyl cyclase/PKA signaling pathway [22,23].
According to previous reports, there are biotechnological and genetic engineering ways to improve the resistance of potatoes to the CPB [24,25]. These transgenic potatoes contained the cry3A toxin encoded by cry3A gene, which has been cloned and expressed in potatoes to specifically target CPB. Since 1996, potatoes expressing cry3A protein, which are resistant to the CPB, have been available commercially in the USA. However, because of low sales and buyer focus on genetically modified organisms, those potato varieties containing cry3A gene had been withdrawn from the market in 2001 [26]. There are less reports about the resistance of transgenic potato plants to CPB in China. Furthermore, the spreading speed of CPB is fast in the Xinjiang Uygur Autonomous Region. There is an urgent need to develop new transgenic insect-resistant potato varieties to reduce the potential damage by CPB. It is hoped that the new insect-resistant potato varieties will be suitable for cultivation in the Xinjiang Uygur Autonomous Region and that there will be a low adaptation of the CPB to cry3A protein.
The CaMV 35S promoter is a distinguished example and is most widely used in transgenic plants [27,28]. There are potential biosafety (in agriculture and human health) and environmental hazards involved as well [29]. With the CaMV 35S promoter, the foreign gene is expressed in all tissues during plant growth and development [30]. There is no effective temporal or spatial regulation of target gene expression, which requires consumption of excessive matter and energy within the cells [31]. Potato leaf and stem-specific promoter ST-LS1 is a light-inducible, single-copy gene from potato that is expressed only in photosynthetic tissues (leaf/stem) [32,30,33]. We are studying the resistance in transgenic potato expressing cry3A to CPB driven by the CaMV 35S and ST-LS1 promoters to find the difference of expression of toxicity and expression organization. At the same time, the transgenic potatoes driven by ST-LS1 promoter cannot express cry3A protein in tubers, which is safer for use as food.
The goal of the study is to develop CPB-resistant transgenic plants to reduce damage to potato crops and halt the spread of CPB eastward into Gansu Province and the Inner Mongolia Autonomous Region of China.
2 Materials and methods
2.1 Plants and insects
The potato cv. “Atlantic” was propagated in vitro by subculturing single-node cuttings on MS medium supplemented with 3% sucrose and 0.45% agar. Plantlets were grown in 150-mL flasks under a 16:8-h white fluorescent light/dark cycle at (24 ± 2) °C. Microtubers were induced under dark conditions at (24 ± 2) °C on a MS medium supplemented with 8% sucrose and 0.45% agar [34]. The CPB came from the Institute of Plant Protection, Academy of Agricultural Sciences of the Xinjiang Uygur Autonomous Region, and reared on light-incubator-grown cv. “Atlantic” potatoes at (25 ± 2) °C under a 14:10-h light/dark photoperiod for insect resistance assays.
2.2 Construction of the plant expression vector
The cry3A gene nucleotide sequence was re-modified by using the codon usage bias of potato according to pooled sequences of potato genes from GenBank, and was chemically synthesized based on the sequence of the cry3A gene (GenBank accession No. M84650) [35]. The fragment of synthesized cry3A gene was digested with BamH I and Sal I from the clone vector and ligated into a binary vector pBin438 [36]. The re-modified cry3A gene was separately driven by a strong constitutive CaMV 35S promoter and a stem and leaf-specific ST-LS1 promoter. The resulting vectors, called pBI121-CaMV35S-CryIIIA and pBI121-ST-LS1-CryIIIA, were respectively transformed into Escherichia coli DH5α, which was further verified by performing the same restriction endonuclease digestion assay. After confirmation, pBI121-CaMV 35S-CryIIIA and pBI121-ST-LS1-CryIIIA were transformed into Agrobacterium tumefaciens LBA4404 using the freeze-thaw method [37,38].
2.3 Transformation of potato and PCR detection
Successful transformation of potatoes was performed according to the method proposed by Si et al. [39]. New shoots were first derived from the center of microtubers of potato slices that had been infected with the Agrobacterium culture. Then, the putatively transformed shoots took roots on the solid medium containing MS salts, 100 mg/L kanamycin and 200 mg/L cefotaxime. About 100 plants were regenerated with kanamycin resistance and transferred into the MS medium. High-quality DNA was isolated from the leaves of putatively transformed and untransformed control potato plants for PCR according to the methods proposed by Edwards et al. [40]. The part of the coding sequence of the cry3A gene was amplified using a PCR Screening Kit (GenStar, Beijing, China) with forward primer (5′-CAGAAGATTGCCGATTACG-3′) and reverse primer (5′-GAGTCGTTACCGTAGTATCCTG-3′). Amplification was performed in a thermal cycler (T100TM, BIO-RAD) programmed for one cycle of 4 min at 94 °C followed by 35 cycles of 1 min at 94 °C, 1 min 30 s at 55 °C, and 1 min at 72 °C. A final extension step was performed for 10 min at 72 °C. The amplification products with 709 bp in length were separated by electrophoresis on 1.0% agarose gels treated with GoodView II staining.
2.4 Gene expression analysis by qRT-PCR
Total RNAs were isolated from the transgenic lines and control plants using RNAsimple Total RNA Kit (TIANGEN, lot#N2822) following the manufacturer's instructions. Reverse transcription was performed in 20-μL reaction mixtures with the RevertAid First Strand cDNA Synthesis Kit (Thermo, 3K1622) and qRT-PCR amplification was performed in 20-μL reaction mixtures with the SuperReal PreMix Plus (SYBR Green) (TIANGEN, lot#N3113), 10 μM of each primer (ef1a as an internal control gene and forward and reverse primers: 5′-CAAGGATGACCCAGCCAAG-3′ and 5′-TTCCTTACCTGAACGCCTGT-3′, and the gene-specific forward and reverse primers: 5′-TCTACAGAGCCGTGGCAAAC-3′ and 5′-CTGGGATGGTTCCTCTGC TAC-3′). Reactions were conducted on the Mxpro (Applied Stratagene Mx3005p Real-Time PCR) System using the default cycling conditions (30 s at 95 °C and 40 cycles of 95 °C for 5 s, 60 °C for 34 s, 15 s at 95 °C, 1 min at 60 °C and 95 °C for 15 s). Each experiment was repeated three times independently. After each reaction, which included a no-template control, a dissociation curve analysis was carried out to verify the specificity of the amplification. 2−ΔΔCT was used to calculate relative expression levels.
2.5 Evaluation of resistance of transgenic plants to CPBs in the laboratory
The insect resistance bioassays were performed according to methods described by Cooper et al. [26], with minor modifications [10,41]. The potato plants in vitro, grown for about 25 days, were detached from the top of the transgenic and control plants with about 75 mm high. The wound of the stem was wrapped in a wet tampon, which was placed in a Petri dish (90 mm diameter). A dish was put about five detached plants, which were inoculated with five heads of first-instar larvae of the CPB and weighed on a laboratory balance (Sartorius, Model Number: BSA). The consumption of foliage and insect biomass accumulation was recorded on the fifth day, and the mortality of larvae was investigated from the third to the fifth day after inoculation. Five replicates were carried out in all groups. The insect resistance bioassays were conducted in Urumchi, the Xinjiang Uygur Autonomous Region in August 2014. Leaf consumption, insect biomass accumulation, and mortality of the CPB were investigated in all experiments. The data sets were analyzed by one-way ANOVA using the SPSS 17.0 and Duncan's multiple-range test.
2.5.1 Mortality
The numbers of deaths were recorded on the third, fourth, fifth day, and the mortality on the third to fifth days was worked out.
2.5.2 Insect biomass accumulation
The weight of 30 first-instar larvae was recorded before inoculation, and the weight of larvae in every dish on the fifth day was investigated. Then, the weight per larva was calculated before inoculation and on the fifth day after inoculation. Insect biomass accumulation was estimated as: MRGR = (lnW2 – lnW1)/DD [42], where MRGR: mean relative growth rate; W1: weight of per larva before inoculation; W2: the weight per larva on the fifth day after inoculation; DD: development days.
2.5.3 Consumption
The weight of foliage before inoculation and on the fifth day was investigated. Depending on if it were the third/fifth or the fourth/fifth day of insect larva biomass accumulation in NT, we obtained two constants: 0.3029 and 0.8951. Foliage consumption (mg/larva/day) = (M1–2)/5(N3 × 0.3029 + N4 × 0.8951 + N5), where M1: the weight of foliage before inoculation by larvae; M2: the weight of foliage on the fifth day after inoculation with larvae; N3: the number of the dead larvae within three days; N4: number of dead larvae on the fourth day; N5: number of living larvae on the fifth day.
3 Results
3.1 Potato transformation and identification of the transgenic plants
Microtuber slices of potato cultivar “Atlantic” were co-cultured (media: MS + 1 mg/L, IAA + 0.2 mg/L, GA3 + 0.5 mg/L, 6-BA + 2 mg/L ZT) for two days with A. tumefaciens LBA4404 containing the plasmid pBI121-CaMV 35S-CryIIIA and pBI121-ST-LS1-CryIIIA respectively, then transferred into the selected medium supplemented with 50 mg/L of kanamycin. After three weeks, green buds sprouted directly from the surface of the slices (Fig. 1A). When the green shoots reached a length of 1 cm, they were transferred to a selective rooting medium containing 100 mg/L of kanamycin. Roots were formed in about 10 days (Fig. 1B). Plantlets with well-developed roots were propagated for further molecular analysis. Genomic DNA indicating the presence of the cry3A gene in the putative transformed plants was extracted and confirmed by PCR assay. The result showed that the transformed plants had a 709-bp amplification product, which was missed in control plants (Fig. 2). qRT-PCR analysis showed that cry3A gene expressed in roots, stems and leaves, except the roots contained the ST-LS1 promoter and the whole tissue in the control plants, and had higher expression in leaves than in stems and roots (Fig. 3).
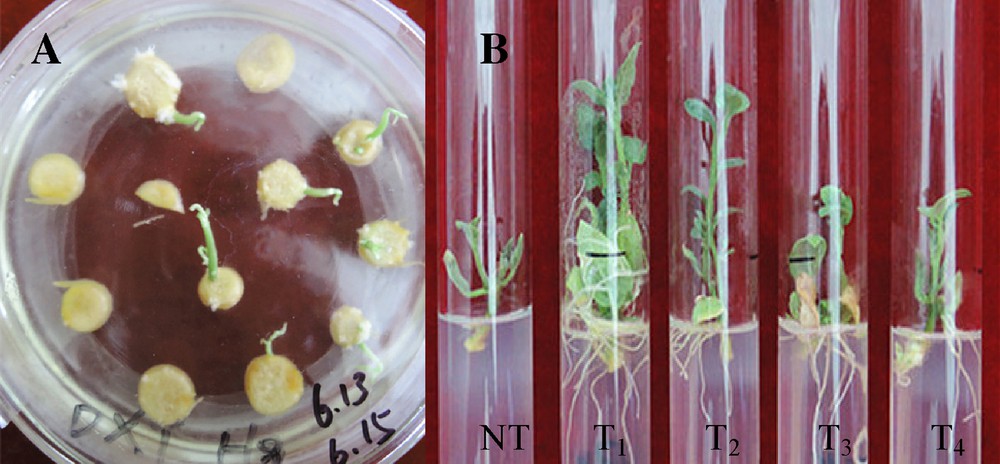
(Color online.) Potato transformation. A. Shoots formation directly from transgenic microtuber discs of the potato cultivar “Atlantic” after three weeks of culture in the selective medium (MS + 1 mg/L, IAA + 0.2 mg/L, GA3 + 0.5 mg/L, 6-BA + 2 mg/L, ZT + 50 mg/L, kanamycin + 200 mg/L carbenicillin) and incubated under a photoperiod with 16 h light/8 h dark cycles at 24 °C. B. The roots were formed in about 10 days when green shoots were transferred to the selective rooting medium (MS + 100 mg/L, kanamycin + 200 mg/L carbenicillin). NT: untransformed potato plant as negative control; T1–T4: the transgenic potato plant lines.
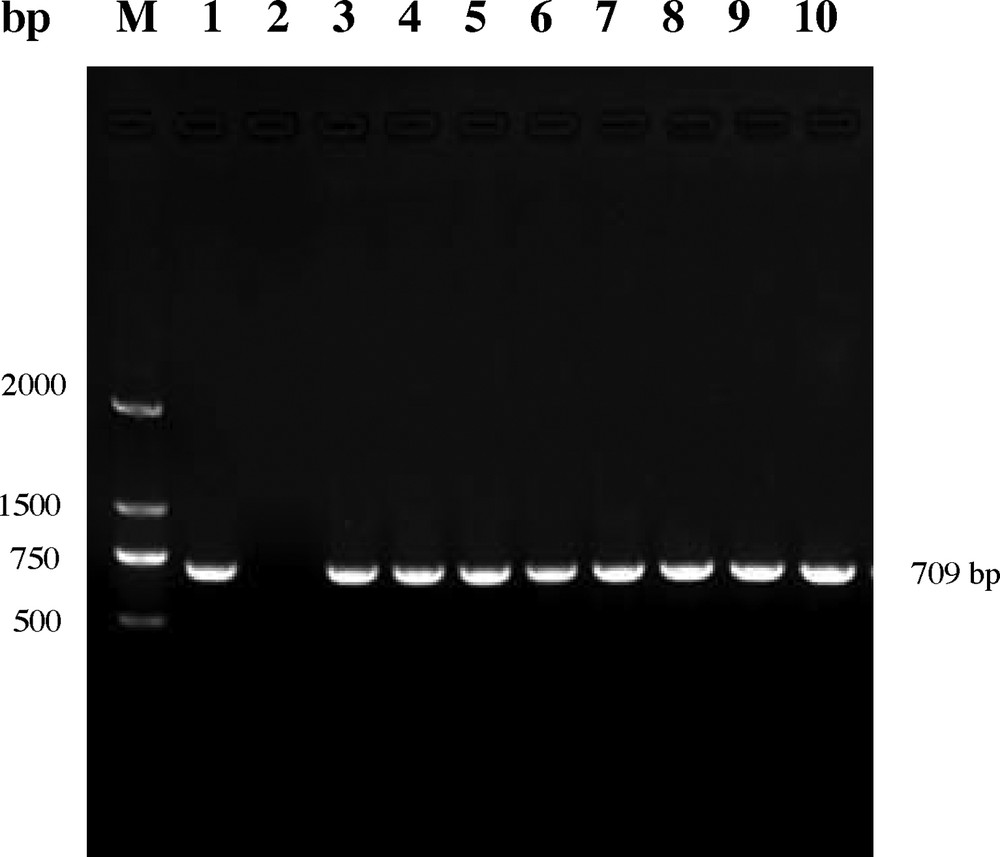
Verification of the transgenic plants by PCR assay. M. DL2000 marker (TaKaRa); 1, plasmid pBI121 as a positive control; 2, untransformed potato plant as a negative control; 3–10, transgenic potato plants.
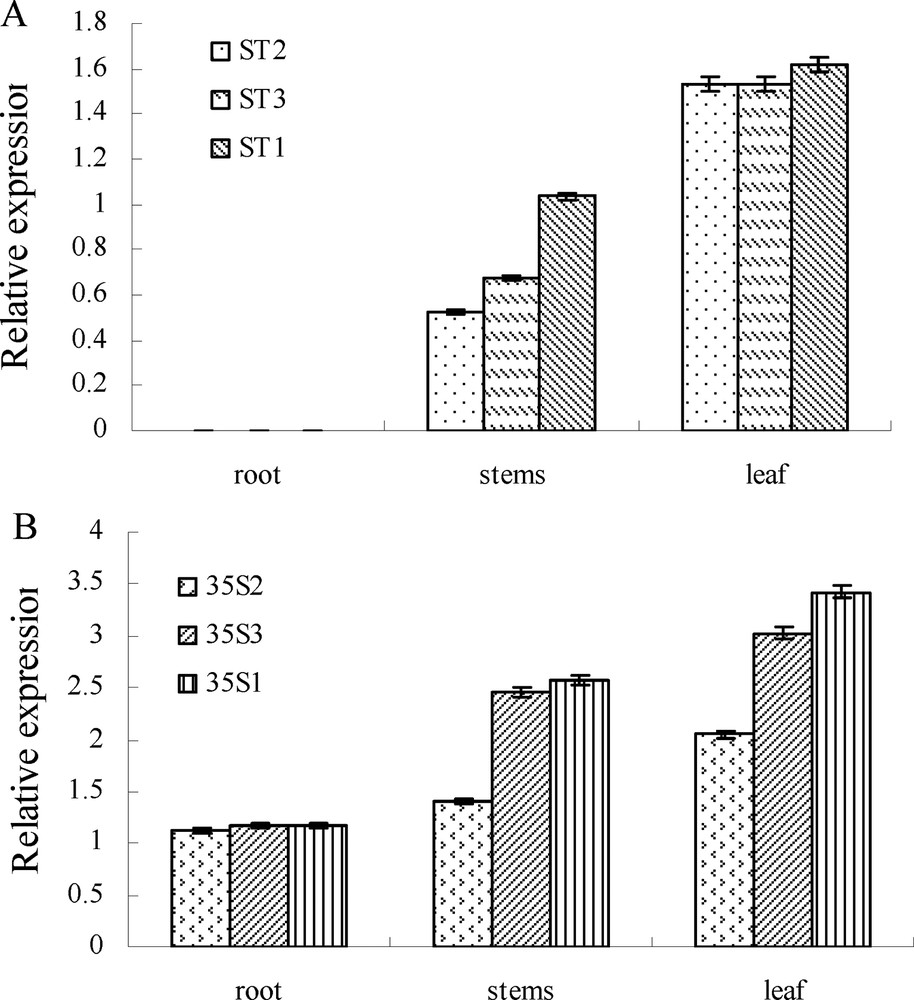
Tissue-specific expression assay of the transgenic plants by qRT-PCR. A. The transgenic plants transformed with pBI121-ST-LS1-CryIIIA. B. The transgenic plants transformed with pBI121-CaMV 35S-CryIIIA.
3.2 Bioassay of resistance of the transgenic lines to CPB
In order to investigate the effect of cry3A on the survivability of CPB, the insect resistance was carried out in Petri dishes (Fig. 4) over a five-day period using the transgenic lines.
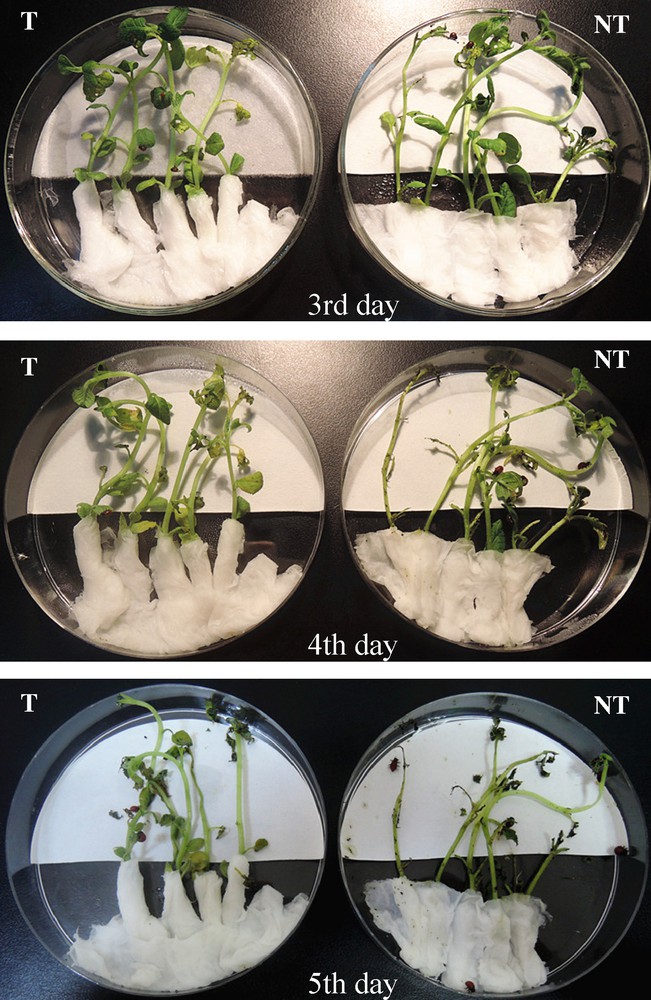
(Color online.) Transgenic and untransgenic control plants on the third and fifth day after inoculation with first-instar larvae. NT: untransformed potato plant as a negative control; T: transgenic potato plant line 35S1.
The variation of mortality about first-instar larvae fed on the control and transgenic plants in three to five days is shown in Fig. 5. Firstly, the total mortalities of the larvae on the transgenic lines were higher than those on the control, and they increased from the third to the fifth days. But the mortalities of the larvae on the control were unchanged and maintained at 0%. On the third and fourth days, the mortality of the larvae was lower, without significant difference except on the plant line 35S1 (28% and 36%). On the fifth day, the mortality of the larvae on plant line ST3 (48%) was higher than that on the other transgenic lines, with significant difference. Combined with the mortality on the third to the fifth day, the mortality of larvae fed on 35S1 and ST3 lines was higher.
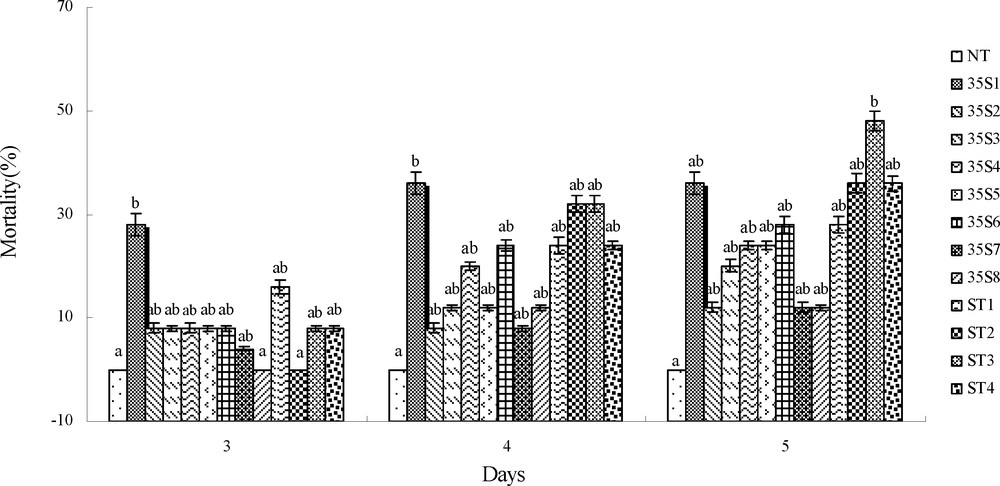
Mortality of first-instar larvae fed on untransgenic control and transgenic plants on the third to fifth days. NT: untransformed potato plant; 35S1-35S8: the transgenic plant lines are driven by CaMV 35S promoter; ST1-ST4: the transgenic plant lines are driven by ST-LS1 promoter.
The larvae on the transgenic plant foliage showed some significant differences in insect biomass accumulation over the five-day period (P < 0.05). The biomass accumulation of all instars was lower on the transgenic foliage than on the control (Fig. 6). Compared with the control foliage, insect biomass accumulation on 35S1, 35S2 and ST3 transgenic foliage was significantly lower (0.42%, 0.43% and 0.42%). Insect biomass accumulation on 35S3, 35S5 and ST4 transgenic foliage was significantly lower (0.49%, 0.42% and 0.52%), which indicated that different transgenic foliage had varied larval growth inhibition, largely due to little or no growth of surviving larvae and the presence of dead individuals. The survivors on the transgenic foliage (35S1, 35S2, 35S3, 35S6 and ST3, ST4) were obviously smaller than their original size, extremely weak. However, on the transgenic foliage (35S5, 35S7, 35S8 and ST1, ST2), many survivors of the instars still grew, albeit their development had been inhibited compared with those on the control foliage.
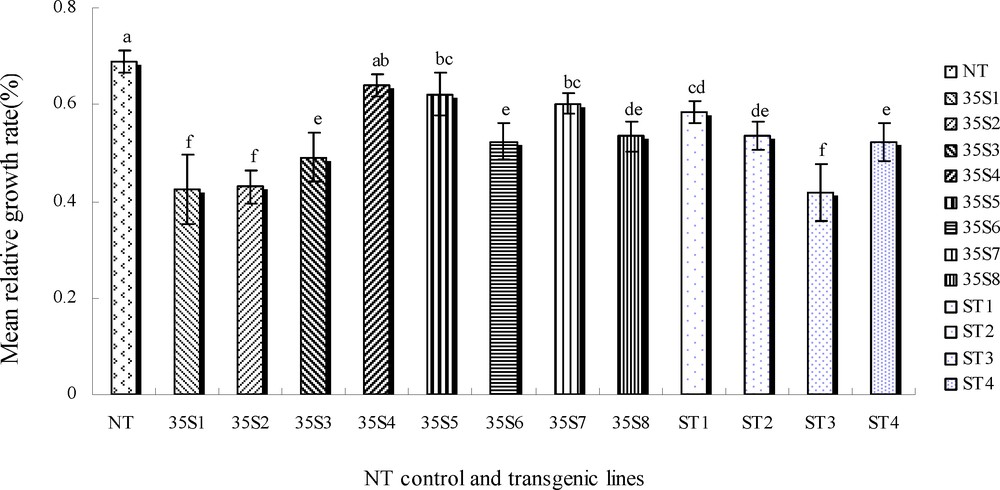
Mean relative growth of first-instar larvae fed on untransgenic control and transgenic plants on the fifth day. NT: untransformed potato plant; 35S1-35S8: the transgenic plant lines are driven by CaMV 35S promoter; ST1-ST4: the transgenic plant lines are driven by ST-LS1 promoter.
The first-instar larvae were especially susceptible to the cry3A protein toxin, so the consumption feeding in all transgenic plants was lower; however, it was higher in the control plants on the fifth days after inoculation (Fig. 7). Feeding was lowest on the transgenic lines (35S8 and ST2) among all plant foliage (7.47 mg/larva/day and 12.46 mg/larva/day). Consumption on transgenic foliage (35S2, 35S3, 35S6) was lower. The consumption on ST1, ST3 was the highest in all transgenic foliage (21.52 mg/larva/day and 26.01 mg/larva/day).
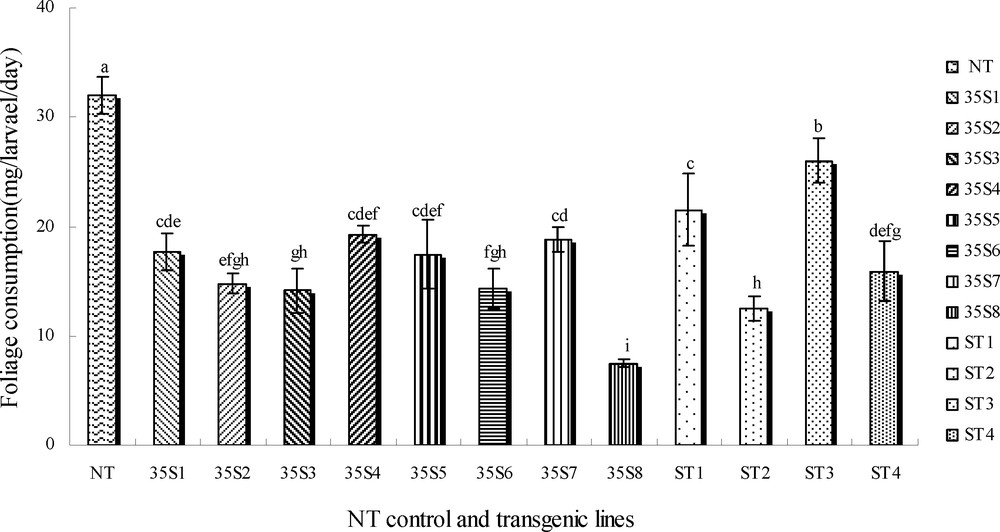
Foliage consumption of first-instar larvae fed on untransgenic control and transgenic plants on the fifth day. NT: untransformed potato plant; 35S1-35S8: the transgenic plant lines are driven by CaMV 35S promoter; ST1-ST4: the transgenic plant lines are driven by ST-LS1 promoter.
4 Discussion
Potato ranks as the fourth most important food crop in the world, behind corn, rice, and wheat [43,44]. Potato is also an important food crop in China. Presently, the goal of many agricultural researches is to increase the production of crops. However, CPB has destroyed almost all fields in the Xinjiang Uygur Autonomous Region, which has brought about a significant threat to potato production, and it has become a serious problem for national food security. Therefore, controlling the damage caused by CPB and its spread has become a vital issue in China. There are some measures, including genetic engineering, applied in order to reduce the damage caused by the CPB to potato crops and stop it's spread eastward toward other potato-producing areas [9].
The wild type Bt-cry gene was found in B. thuringiensis, and its expression was generally low in eukaryotes. Since the insecticidal Bt protein has been found, it has been continually hard efforts to transfer Bt gene into higher plants, and to improve its expression by re-modifying cry genes [45–47]. In this experiment, we obtained the transgenic potato plant lines expressing cry3A gene. The expression of cry3A gene was higher in leaves than in stems and roots, and no expression was detected in roots of the transgenic plants driven by the ST-LS1 promoter. Despite the growth and use of transgenic crops in many areas of the world, some governments, organizations and individuals still speculate the risk that they can pose to the environment and health. In the study, we used the leaf and stem-specific ST-LS1 promoter to make no cry3A gene expression in stems and tubers, which seems to be more favorable to the transgenic biosafety for food.
Up to now, the transgenic crops have widely appeared in many countries, and many insect-resistant strains, such as rice, cotton, creeping bentgrass, tall fescue, Arabidopsis and Brachypodium distachyon have been planted in the laboratory and in greenhouses [48–54]. In this study, the results from inoculation with first-instar larvae on plants showed that larvae in some transgenic lines led to higher mortality, lower insect biomass accumulation and foliage consumption. Different transgenic lines have varying larval growth inhibition abilities. At the same time, we also found that the survivors inoculated with the transgenic lines were weak, moved slowly and had poor digestion. The results suggested that the transgenic potato plants expressed cry3A protein could effectively enhance the resistance to CPB in potato cultivar “Atlantic”. This study not only was as a theoretical investigation but also brought about important economic interests. We can further develop the transgenic potato variety to control CPB damage in the Xinjiang Uygur Autonomous Region and prevent eastward spread of CPB into Gansu province and the Inner Mongolia Autonomous Region of China.
Acknowledgements
This work was supported by the Agriculture Science and Technology Innovation Project of Gansu Province of China (GNCX-2011-49) and the Special Fund of Agro-Scientific Research in Public Interest of China (201103026-2). We would like to thank Professor Wenchao Guo (Institute of Plant Protection, Academy of Agricultural Sciences of Xinjiang Uygur Autonomous Region, Urumchi, China) for help in CPB resistance bioassay tests.