1 Introduction
Surgery and pharmacology have always been the only therapeutic tools for neurodegenerative diseases. In the field of movement disorders, surgery came first [1,2], but had complications, particularly when it was performed bilaterally. The introduction of the treatment by the Dopamine precursor LevoDopa [3] was successful in compensating the loss of production of Dopamine by the degenerated substantia nigra pars compacta (SNc), and eradicated surgery. However, the LevoDopa-induced dyskinesias and motor fluctuations, as well as the complications of surgery called for new methods to complement the therapeutical panel. The discovery [4] that the effects of deep-brain stimulation depend on the frequency provided such an alternative
2 Paradoxical effects of high-frequency stimulation
Electrical stimulation is usually associated to excitation of neural elements. Depolarization induced by cathodal stimulation triggers the opening of sodium voltage-dependent channels and leads to the initiation of a spike, self-reproducing and propagated along the axon in a non-decremental manner. Repetition of the stimuli leads to an increased efficiency, due to temporal and spatial summations. This is not a linear phenomenon and the excitability of the membrane increases with frequency until an optimum above which excitability decreases again. Stimulation is used as a tool to explore the functions of neural structures, in various circumstances and particularly during surgical procedures to help defining the target.
Intraoperative thalamic stimulation abolishes Parkinsonian rest tremor at frequencies above 100 Hz.
It is during a surgery for thalamotomy in a patient with essential tremor [4], who underwent some months ago a thalamotomy on the other side, which we performed for the first time the implantation of an electrode aimed at delivering high-frequency stimulation [HFS]. The reason was that performing a bilateral thalamotomy is usually considered as bearing a higher risk of complication, particularly neuropsychological complications [5–9]. We had observed during previous surgeries that using stimulation for exploration purposes, at low and high frequency, that high frequency (i.e., higher than 100 Hz) was able to stop the tremor, while low frequency (lower than 50 Hz) was not able to stop it or was even able to drive the tremor at frequencies around 5 to 10 Hz. This observation was used for several patients in a row as an additional tool for locating the best site where to make the lesion of thalamotomy. The idea came quickly that this could be used permanently as a treatment, because of the availability of the hardware, previously designed to deliver low-frequency stimulation in the nervous system or along the spinal cord, mainly for treatment of intractable pain.
Using this method in patients who at that time were indications for thalamotomy, and had either rest tremor (patients with Parkinson's disease (PD)) or postural tremor (patients with essential tremor (ET)), it was quickly demonstrated that deep-brain stimulation (DBS) at high frequency (HF) replaces ablative stereotactic methods [4,10]. The electrodes were stereotactically implanted, during a procedure guided by a combination of ventriculography, magnetic resonance imaging (MRI), and electrophysiology (using both microrecording and microstimulation). These electrodes were then connected to programmable pulse generators, implanted in the subclavicular area similarly to cardiac pacemakers. Contrarily to thalamotomy, DBS could be well tolerated when performed bilaterally in the same session. Stimulation was continuous, unipolar (the electrode being the cathode and the case of the stimulator the anode), with frequency at 130 Hz, pulse width at 60 μs, amplitude around 2–5 V.
This empirical observation-based concept could be then applied, in addition to the thalamic target Vim (ventral intermedius thalamic nucleus) for rest tremor and for postural essential tremor, but also to the other classical targets for PD, particularly to the internal pallidum GPi [11–14], known for its specific effect on levodopa-induced dyskinesias and used in pallidotomies [15,16].
The demonstration that the method was reliable, and safe comparatively to lesioning methods, allowed its extension to new targets, theoretically designed from basic neuroscience, such as the subthalamic nucleus (STN) [17–20].
One may consider that, as a conclusion of this first period, one had established the empirical concept ‘HFS is equivalent to Lesion’, stating that HFS-DBS produced a functional inhibition mimicking the effects of lesion. This concept has currently the status of an axiom as it is verified in every circumstance where it is applied (not only in all three targets used for PD, but also in other clinical indications such as the posterior hypothalamus for cluster headaches [21,22], or in experimental animals in the ventromedial hypothalamus [23] where changes in feeding behaviour can be induced), and still cannot be demonstrated. This concept has two consequences:
- − Are there other indications?
- − Is this a new physiological concept? And if so, what is its mechanism?
3 Other indications
Based on these clinically proven experiences, several other indications have been explored and are treated, either on the basis of the extension of the concept to targets having already proven their efficiency, or on the basis of theoretical expectations, drawn from experimental evidence.
3.1 HF-DBS for dystonia
Since the previous reports of [24], thalamotomies were performed either in Vim or in more anterior targets, such as ventro-oral posterior or anterior thalamic nuclei (Vop or Voa), and even in the pallidum. HFS of Vim, although providing some benefits [25], has not been very successful for treating dystonia. Following the reports of improvement of dystonia by pallidotomy [13], HFS has been tried in GPi where once gain it mimics the effects of lesions created by pallidotomy [26–28]. It is striking to observe that in this particular indication the similarities between the effects of HFS and lesion are concern also the time course of improvement of symptoms. While in PD, the effects of HFS are almost immediate (within seconds) and similarly reversible, in dystonia, these improvements are not seen before a rather longer delay, of days if not weeks and keep improving along time even over some years. This delayed time course is similar for HFS and for pallidotomies, proving that this is related to the nature of the cause of the dystonia rather than to the method of altering the function of GPi.
3.2 HF-DBS for epilepsy
It has been known for long [29,30] that cortical excitability may be modified by pharmacological and lesioning manipulations of the nigral system, leading to the concept of ‘Nigral Control of Epilepsy’. Injection of GABA agonists or NMDA inhibitors in the substantia nigra pars reticulata (SNr), or GABA antagonists into the superior colliculus induces an anti-epileptic effect on most of the experimental models of epilepsy in laboratory animals. Based on these observations and on our previous experience of HF-DBS in the STN for PD, and on the direct projections of STN onto SNr, we have implanted STN in several cases of intractable epilepsy with significant results [31,32], opening on a clinical controlled trial of STN HF-DBS in epilepsy cases due to genetic anomalies (ring chromosome). Other targets are being considered, such as the anterior thalamus, projection site of the mamillo-thalamic tract. In these cases, the efficiency is still obtained at 130 Hz. Other data are being reported on the efficiency of direct stimulation of the amygdalo-hippocampic formation [33] or even the epileptic focus itself [34].
3.3 HF-DBS for OCD
The discredit of psychosurgery during the second half of the 20th century has deprived some psychiatric patients from a very useful therapy when all other treatments have failed. Capsulotomies and cingulotomies were still performed in rare institutions, but the risk of complications and their irreversible nature has been a persisting restriction. The reversibility of HF-DBS has reopened an opportunity. Obsessive compulsive disorders (OCD) have been the psychiatric condition that benefited the most from psychosurgery when indications were correctly chosen. For this reason, DBS was used to replace lesions in the internal capsule [35]. The reported results were encouraging, but the parameters used (high voltage, large pulse width) suggested that the neuronal structure involved was at distance from the real target, which could be, according to the current understanding of the physiology of the basal ganglia, the nucleus accumbens. Recent and preliminary data reporting results of HF-DBS of the nucleus accumbens are in agreement with this hypothesis. HF-DBS of STN in two PD cases that had in addition traits of OCD showed that the improvement of the PD symptoms was paralleled by the improvement of the OCD symptoms, as evaluated with the Y-BOC rating scale [36]. A third unpublished case seems to confirm these preliminary observations. A multicentre-randomized double-blinded study has been initiated to verify or delete this hypothesis.
3.4 HF-DBS for cluster headaches
Cluster headaches might be a devastating disease, occurring repeatedly in patients prone to this vascular dysregulation. PET-scan studies have shown that the episodes corresponded to a hyperactivity of the ipsilateral posterior hypothalamus [37]. The implantation of this area with electrodes has demonstrated a striking, acute, reversible, and ipsilateral effect of high-frequency stimulation [21,22].
4 Future applications?
4.1 HFS for obesity or anorexia nervosa?
The ventromedial hypothalamus, and the lateral hypothalamus are structures involved in the control of feeding behaviour. Lesions of the hypothalamus due to lipomas or secondary to surgical lesion (such as after surgery for craniopharyngiomas) may also induce malignant obesity. Experimental data in dogs and cats demonstrated that lesion of the VMH or low frequency stimulation of LH induce hyperphagia and then obesity, while low frequency stimulation of VMH and lesion of LH induce the reverse behaviour, leading to a cachectic state. In rat experiments [23] HF-DBS of VMH in rats induce hyperphagia, while low frequency stimulation decreases the food intake of fasted rats
These are experimental data, suggesting that HF-DBS could be used for treatment of obesity or anorexia mentosa. However, this requires more experimental data and raise important ethical problems to be solved prior to the first clinical attempts
As a conclusion of this second part, HF-DBS is a surgical tool, adaptable, reversible, usable as substitute for lesions in various neural structures and applicable to several targets at the same time. It seems to have wide applications in various targets, and future developments can be foreseen. We still have to understand its mechanism and to investigate its effects
5 Mechanism
The challenge is to solve the following paradox: how can stimulation produce the same clinical effects than lesions in various structures of the brain? Actually, the facts are different depending on the frequency. HFS (more than 50 Hz, actually at about 130–185 Hz) mimics the effects of ablative procedures (considered as inhibitory) in neural somatic structures such as the thalamus (Vim, CM-Pf), the basal ganglia (GPi, STN) and the hypothalamus (VMH). On the contrary, HFS and LFS excite neural-fibre bundles, such as the optic tract (inducing flashes), the pyramidal tract (inducing contractions), the lemniscus medialis (inducing paresthesias), or the third nerve fibres (inducing mono-ocular deviations). When a chronic electrode (1.27 mm in diameter) bearing four contacts (1.5 mm long, separated from the next one by 0.5 mm), with a voltage of about 2 to 3 V, which corresponds to 2 to 3 mA as the average impedance of the system is about , the area involved by the suprathreshold currents is not limited to a point and even to a homogeneous structure such as STN, comprises neuronal somata, of course, but also their dendritic fields, the corresponding synapses and the afferent fibres, as well as the efferent axons of the neurons. The different elements of the neurons respond differently to stimulation. Fibres have a lower threshold than the cell bodies; the orientation of the fibres is important depending on they are parallel or perpendicular to the current lines. Stimulation might induce the silencing of the neuronal firing, the jamming of a network or a feedback loop that would make the wrong message erased or meaningless, the fatigue of synapses by depletion of the neurotransmitter. Several of these mechanisms could be involved altogether in the same structure or at different levels, which allows us to propose a tentative model for the mechanism (Figs. 1 and 2).
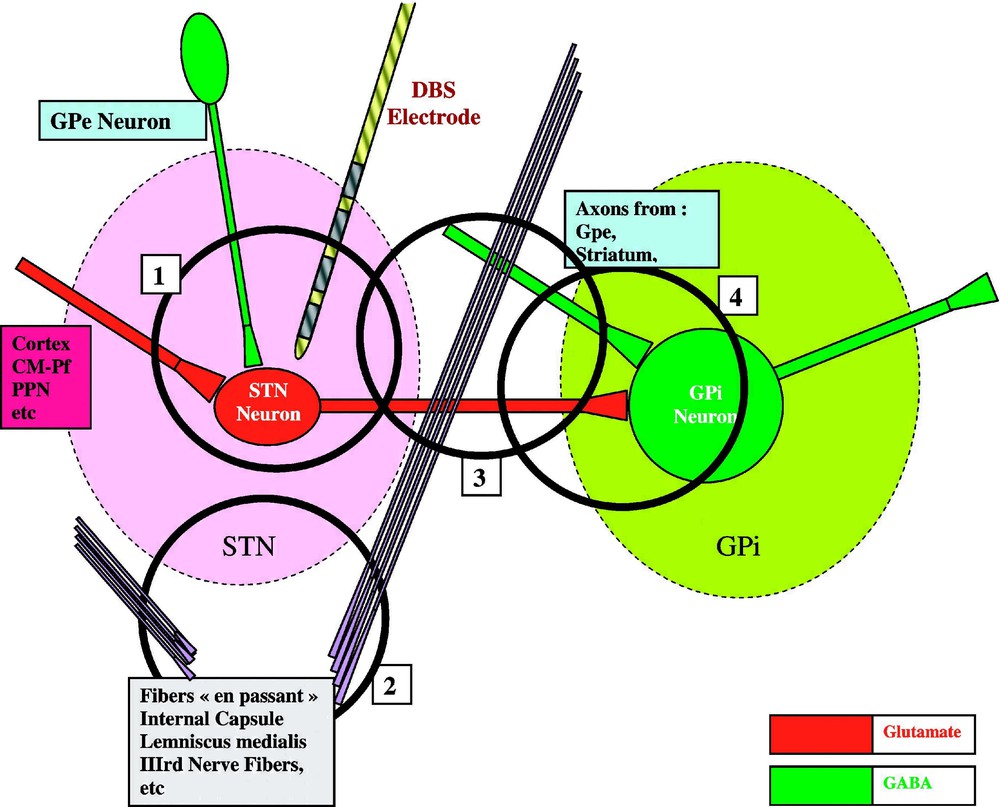
A global model of HFS-induced functional inhibition. Within STN nucleus, where the chronic DBS electrode is inserted, a STN neuron receives Gabaergic afferents from Gpe and Glutamatergic afferents from the cortex, the CM-Pf and PPN nuclei, and emits Glutamatergic efferents to the target nuclei GPi (and SNr not represented here), which receives also terminals from other origins (such as Gpe and the Striatum). Not directly related to this network, axons pass by these structures, constituting the Internal Capsule, the Lemniscus medialis, or lower and more medial, the fibres of the third nerve.
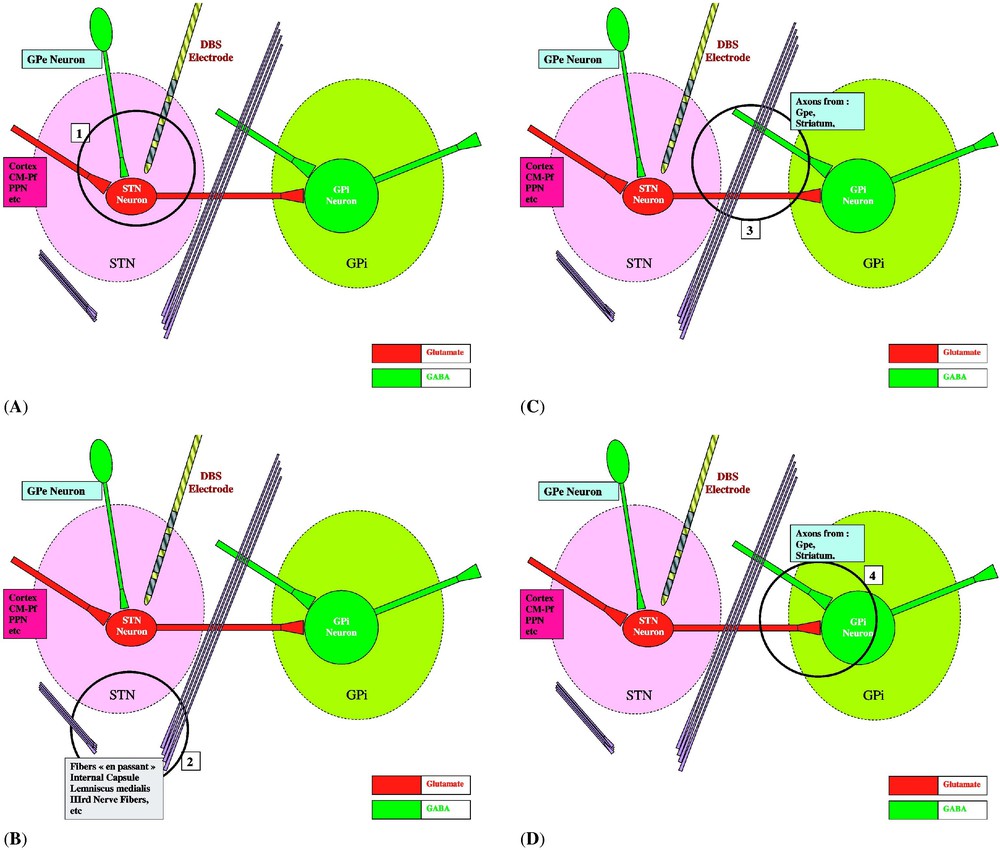
(A) HFS inhibits spike initiation at the level of the STN membrane [40,42,46] and decreases neurotransmitter (glutamate) synthesis and synaptic release [49]. Axons impinging upon the stimulated neurons and their terminals would be excited and would deliver an increased amount of their neurotransmitters. However, their postsynaptic effect (initiation of EPSPs by glutamate terminals coming from the cortex, the CM-Pf and PPN nuclei, or of IPSPs by GABA terminals coming from GPe) might be annihilated by the inhibition of the spike initiation at the level of the postsynaptic STN membrane by HFS (1 in Fig. 1). (B) Axons passing by the stimulated structure but coming from cell bodies which are far from the site of stimulation (such as the fibres of the internal capsule or the fibres of the third nerve) would be excited, therefore inducing remote side effects (gaze deviation, monocular deviation, muscular contractions, sensory paresthesias) (2 in Fig. 1). (C) Axons (subthalamo-pallidal, pallido- and striato-pallidal, and axons en passant) are excited at low and at high frequency. The effect of HFS on these axons depends on their origin, whether or not their cell bodies are within the stimulated area (3 in Fig. 1). (D) Axons originating from the stimulated STN neurons are probably stimulated and generate spikes that are propagated to the subthalamo-pallidal (or nigral) synapse: however, at this level, the neurotransmitter cannot be released anymore, as its synthesis would have been inhibited at the level of the STN cell body [49,50]. If this inhibition is insufficient, the spikes that are not inhibited are propagated under a disrupted pattern of firing; this would render the final neuronal message meaningless for the whole network [47], in a global process which we have called ‘jamming’. Axons originating from Gpe and/or the striatum may be excited when passing close to the stimulated area: they are Gabaergic and should participate to the inhibition of the target structures, GPi and SNr (4 in Fig. 1).
At the level of the cell body, there is now evidence that the neuronal firing is inhibited: direct recording of neuron activity at the site of stimulation has been done in the GPi [38,39], as well as in the STN [40,41], which show that spikes are not induced during HFS, as well as immediately after stimulation [42–44]. However, the stimulation field necessarily encompasses also the neighbouring axons, either originating within the stimulated structure (such as subthalamo-pallidal axons), or passing by it (such as in the F1 and F2 Forel fields, or in the internal capsule). This might explain the results of Garcia et al. [45] on rat-brain slices: HFS of STN induces a dual effect, made of inhibition of firing interrupted by burst of spikes driven at the frequency of stimulation, which might be due to a retrograde propagation to the cell body of axonal spikes initiated along the part of the STN axon leaving the nucleus and involved by the electrical field.
At the level of axons, observation of the effect of stimulation during surgical exploration of the target and of its surroundings demonstrates that high-frequency as well as low-frequency stimulations excite these elements. The excitation of the fibres passing close to the site of stimulation induces the expected symptoms: paresthesias in the lemniscus medialis, muscular contractions in the internal capsule at the level of the pyramidal tract, conjugated eye deviation in the geniculate tract of the internal capsule, mono-ocular deviation in the fibres of the third cranial nerve. Therefore, the axons efferent from the subthalamic neurons should be also excited. The induced spikes should therefore travel along the efferent axons and reach the synapses to the target structures, such as SNr or GPi. Recording in GPi during STN stimulation in monkeys shows increased activity [47], but this could be due to activation of fibres close to the site of stimulation, which in the experimental situation of this paper (monkeys stimulated in STN with a human chronic electrode) might overpass the STN nucleus. Anyway, this activity induced in GPi is linked to the stimulation and disturbs the spontaneous activity, which might create at this level a jamming. Microdialysis in the rat shows an increased release of glutamate, presumably coming from the STN. These data are difficult to interpret because the prolonged time course of the release outpasses by large the duration of the effects of STN stimulation. Moreover, the size of the probe as compared to the size of the investigated structure does not allow us to consider these data as representative of the structure, and therefore does not make the microdialysis data reliable [48]. However, axons originating from the STN neurons should be excited as well. They would be therefore responsible for the excitation of the target cells, in SNr as well as in GPi, provided that the spikes are able to induce a synaptic activity at this level. To check this, we have studied the effect of HFS on a prolactinoma cell line producing prolactine in culture. The release of prolactine is decreased by HFS in a similar extent than by the addition of dopamine, the prolactine inhibitor factor. Similar experiments were performed on the neuronal-like cell line PC12 using a long oligonucleotide microchip bearing 5000 rat gene on a nylon film, with radioactive detection. These experiments show that HFS decreases transcription in 80% of the genes [49] (unpublished data).
One may therefore assume that the synapses activated by the axons originating from neurons situated in the area of stimulation cannot release the expected neurotransmitter, making these neurons like ‘firing blanks’ [50] and creating a functional inhibition.
At the level of the cell body, the axon terminals coming from the afferent neurons are mainly glutamatergic from the cortex, the parafascicularis nucleus and the pedunculopontine nucleus, but also GABAergic coming from the external Pallidum GPe. These two types of afferents might therefore be excited and deliver both glutamate and GABA, the last one being dominant in term of the final results. The hypothesis of the GABA action is the one suggested by Dostrovsky et al. [38,39]. Moreover, the axons originating in GPe are retrogradely activated [42]; they could participate to either a jamming or an incoming additional inhibitory GABA input onto STN, participating to the STN inhibition.
On the basis of these considerations, we propose a global model explaining the major observations and supporting the concept of HFS induced functional inhibition (Figs. 1 and 2). HFS at the level of a neuronal structure inhibits spike initiation at the membrane level and decreases neurotransmitter synthesis and synaptic release. Axons originating from the stimulated neurons could initiate and propagate spikes, which however would be inefficient at the synaptic level, considering the above-described inhibition of the neurotransmitter production and release. If this inhibition were insufficient, the disrupted pattern of firing would render the final neuronal message meaningless for the whole network, in a global process that we would call ‘jamming’. Axons impinging upon the stimulated neurons and their terminals would be excited and would deliver their neurotransmitters (provided their somata would be at sufficient distance to avoid inhibition of the neurotransmitter production), but their action might be inefficient because of the inhibition of the spike initiation at the level of the membrane by HFS. Axons passing by the stimulated structure but coming from cell bodies which are far from the site of stimulation would be excited and would deliver an increased output of their neurotransmitters at the level of their target synapses, therefore inducing remote side effects.
6 Effects of STN inhibition: the putative neuroprotective effect of STN-HFS
The Working Hypothesis is that HF-DBS of STN shuts down or significantly decreases the STN output of glutamate, an excitotoxic amino-acid which in PD might participate to the neurodegenerative process. This is based on the hyperactivity of STN in PD [51], the glutamatergic projections from STN to SNr and SNc [52], the glutamate neurotoxicity through NMDA receptors [53], the NMDA enhancement of neurotoxicity of metamphetamine [54], the attenuation of neurotoxicit by NMDA antagonists (MK801, CPP, …) [55]. To test this hypothesis, experiments were done in a 6OHDA rat model and in a MPTP monkey model.
The 60HDA rat program was achieved using the Sauer and Oertel model [56] where intrastriatal injection of neurotoxin 6OHDA induces a retrograde degeneracy of the dopaminergic neurons of the substantia nigra pars compacta (SNc), which is significantly decreased when the neurotoxin is injected after injection of kainic acid into STN [57–59]. The MPTP monkey program was achieved using MPTP systemic injection. Neuroprotection was obtained either by lesioning the STN with kainic acid injections or by chronic STN stimulation through implanted electrodes connected to a permanent stimulator. In all experimental conditions, the ratio between the protected versus the non protected side was about () for the cell count using the Nissl stain and () using tyrosine immunoreactive staining [60].
Long-term effects in human patients were investigated using the OFF medication-OFF stimulation score at patient evaluation in 89 bilaterally-implanted STN cases of PD. Continuing impairment as usual in PD was observed in 25% of the patients, 36% were stable and 38% showed significant improvement, 19% continuously improving over five years. Although this is usually not observed in PG patients in whom annual impairment is about 10% [61], one might be cautious before interpreting these results as neuroprotection, as it is known that long-lasting effects of levodopa treatment have been observed.
The conclusion of this third part is that HF-DBS of basal ganglia is the current surgical alternative in the treatment of Parkinson's disease. It has already several applications to other diseases (dystonias, epilepsy, OCD, cluster headaches) and future indications are being investigated. Its mechanisms are not fully understood, but produce a functional inhibition, with multiple complex components, from the cell to the gene. The physiology of electrical stimulation in general, or even at a larger scale, the biological effects of electricity, might be further investigated.