1 Galvani, the story, the legend and the images reçues
Among the main achievements of the 18th century science is the demonstration made in 1791 by the scientist of Bologna, Luigi Galvani, of the presence in living tissues of an intrinsic form of electricity involved in nerve conduction and muscle contraction. Galvani's discovery laid the grounds for electrophysiology. Moreover, and unexpectedly, it also opened the path to the invention of the electric battery, by Alessandro Volta, thus paving the way to the development of the physics and technology of electricity, with long-lasting consequences for humankind.
According to Galvani, electricity is mainly accumulated between the interior and the exterior of a single muscle fibre: a nerve fibre penetrates inside it allowing, in either physiological or experimental conditions, “the flow of an extremely tenuous nervous fluid [...] similar to the electric circuit which develops in a Leyden jar” [1 (p. 378)]. With the nerve fibre penetrating into its interior, the muscle fibre represented a “minute animal Leyden jar” for Galvani, and by this image he communicated the discovery of animal electricity in an epoch-making memoir in 1791, De viribus electricitatis in motu musculari [1].
In spite of the importance of his research, Galvani's figure is still largely seen as that of a physician of the Ancien Régime, incurring by chance an unexpected observation (a dead frog preparation jumping when a light suddenly sparked off from a distant electric machine), a man who meandered aimlessly in interpreting his further experiments until the physicists of Pavia, Alessandro Volta, entered the field [2,3]. With his own research, Volta would be able to claim that the electricity responsible of frog muscle contraction in Galvani's experiments was not intrinsic to nerve and muscle tissues, but derived from the metals used by the scientist of Bologna to connect nerve and muscle in accordance with his idea of the neuromuscular preparation as a Leyden jar [4,5].
In order to demolish the ‘legend’ of the doctor of Bologna and of his frogs still dominating historiography as well popular imagery, it is necessary to combine an accurate study of Galvani's original sources with an analysis of the historical context and of the scientific problems he was investigating. It is also been essential to evaluate Galvani's experiments and results in the light of modern knowledge on the physiology of nerve conduction.
In this article I shall present the scientific stature of Galvani and his electrophysiological prior to the formulation (in 1791) of his hypothesis of animal electricity. This work is largely based on the research that I have been carrying out over the last ten years in collaboration with Marco Bresadola [6–10].
2 Electricity in the 18th-century natural philosophy and medicine
Electricity was undoubtedly at the centre stage of the scientific interest of the Grand Siècle, the electrical century par excellence, as a consequence of many discoveries, theories and practical applications [11].
There was, in particular, a great interest in the possibility that the electric fluid might have therapeutic effects. Electricity, provided by electric machines or accumulated in Leyden jars, was administered with the aim of relieving a plethora of diseases. New systems of ‘electric medicine’ were proposed where diseases were considered as due to an excess or to a lack of ‘electric fire’, and thus liable to different electric remedies. Enthusiasm was gradually transmuted into deception, as it became increasingly clear that many of the presumed successful medical applications of electricity were such only in the hands of a few practitioners, and could not be easily and constantly replicated by established scientists and physicians [12–14].
Physiologically, the century was dominated by interest in the possible involvement of electricity in nervous function and muscle excitability [10,15,16]. The prevailing view among the supporters of the ‘neuroelectric’ theory was that an electric fluid propagates along nerves, producing sensations or movements according to the final targets eventually hit, i.e., the central regions of the nervous system or muscular tissue. On this respect, electricity was a possible replacement for ‘animal spirits’, the elusive entities considered in classical science as messengers of soul for sensation and will [17,18].
Even if electricity appeared to be a powerful agent for stimulating nerves and muscles, the idea that the nervous agent could be of an electrical nature encountered fierce opposition among many reputed members of the scientific establishment. This was particularly the case for the followers of the doctrine of ‘irritability’, elaborated by Albrecht von Haller, a dominating figure of the 18th-century science. According to his doctrine, muscles contract in response to physiological (or experimental) stimuli, because they are provided with an intrinsic capability to contract (or ‘irritability’), which depends on their intimate substance and organisation, and is not simply a passive outcome of an external agency. Nerves would act on muscles just as stimulating or exciting factors, capable of putting into action intrinsic muscle irritability [10,16,19].
For ‘Hallerians’ it was difficult to accept the electric theory of nervous conduction, because it implied the electric fluid of nerves as the effective agent of muscle contraction. The neuroelectric theory was challenged through a series of arguments by Haller and his followers, who were particularly active in Bologna, such as Marc' Antonio Caldani and Felice Fontana. Since living tissues are made of liquid matters of a conductive nature – they argued – there could not be any stable disequilibrium inside animal bodies, and thus not the force required to move electrical matter through nerves according to the organism's necessities. Moreover, electrical flow could not be restricted to the specific nerve paths required by physiological needs without spreading and causing unwanted physiological actions. A third objection was based on the effects of ligating a nerve with a thread: this manoeuvre abolished nerve conduction (as evidenced by the loss of movements or sensations) but not the passage of electricity along the nerve [20–23].
The objections of the Hallerians set the background for any plausible theory of the role of electricity in nerve physiology. With time, however, the difficulty that living beings could maintain an electrical disequilibrium inside their tissues was undermined de facto by evidence of the electric nature of the shock of torpedo fish and electric eel as provided by John Walsh in the period 1772–1775 [9,24–26]. It appeared particularly significant, and somewhat paradoxical, that animals living in a water milieu could accumulate electricity inside their tissues and manage it according to their needs.
3 The formation of Galvani and the ‘Istituto delle Scienze’ of Bologna
Galvani's interest in neuromuscular function and in the possible therapeutic effects of electricity had a long history. Galvani was a member of the ‘Istituto delle Scienze’ of Bologna, a scientific institution promoted in 1711 by Count Luigi Ferdinando Marsili (a singular natural philosopher, geographer, diplomatic, soldier), who aimed at renewing scientific research and teaching in his native town at a moment in which the old University was suffering an apparently irreversible decline. In addition to the disciplines traditionally flourishing in the University (such as natural history, anatomy and various aspects of medicine), the ‘Istituto’ had a special interest in the new experimental science burgeoning in Europe after Galileo and Newton: astronomy, electricity, optics, pneumatics and chemistry were particularly cultivated in especially designed and equipped laboratories (camere). The members of the ‘Istituto’ were requested to demonstrate and discuss periodically the results of their experimental researches with their colleagues and this favoured an interdisciplinary circulation of scientific theories and methodologies [27–29].
The Istituto maintained an ideological link with Marcello Malpighi, the founder of microscopic anatomy and one of the main renewers of the life sciences in the 17th century. Apart from insisting on the experimental character of scientific endeavours, Malpighi supported the conception of ‘rational medicine’. Medicine should be based on the scientific study of body functions and of their alterations discoverable by new instruments and with new methodologies. It should not rely exclusively on the records of symptoms and of the effects of treatments, and any new finding should be incorporated into a rational scheme or theory [30–33].
In the years of Galvani's introduction to science, Bologna and the ‘Istituto’ were the site of intense cultural and experimental activity. Some of the members of the ‘Istituto’, namely Marc'Antonio Caldani, Felice Fontana and Tommaso Laghi, were engaged in the debate about irritability and the possibility that an electric fluid could play a role in nerve and muscle function. The ‘Istituto’ was also interested in electrical medicine, and in 1747 one of its members, Giovanni Giuseppe Veratti, was asked to verify experimentally the asserted therapeutic efficacy of electric treatments [34].
In 1772, while he held the prestigious chair of anatomy at the Istituto, Galvani himself read a dissertation on Hallerian irritability. The harsh debate on irritability and the discussions on the efficacy of electrical medicine eventually set the stage for Galvani's interest in the study of the effects of electricity in animals. The triggering event for Galvani's decision to start his experiments at the end of 1780 was probably a discussion on the possible physiological relevance of electricity that emerged during the ‘public function of anatomy’ he performed in 1780 [7,10].
In the spirit of Malpighi's ‘rational medicine’, Galvani was convinced that, in order to put electrical medicine on a firm foundation, he had to carry out an accurate experimental study of the action of electricity on nerves and muscles; it was necessary, in particular, to determine if electricity played a role in the normal function of nerve and muscle, as asserted by the supporters of the neuroelectric theory.
Galvani's experimental attitude blended the long-established Bologna tradition of anatomy (with Malpighi as its main reference), with a new more dynamic approach to the study of organisms: this involved the study of living animal preparations, was based on the new instruments that characterised the physical research of the epoch and reflected the new theoretical interests emerging in post-Newtonian science. From the Galvani's writings and from plates illustrating (in 1791) the first publication of his electrophysiological investigations, one is led to envision Galvani's room of experiments as a combination of the cabinet de physique of a natural philosopher of the 18th century along with a dissection room (see Fig. 1).
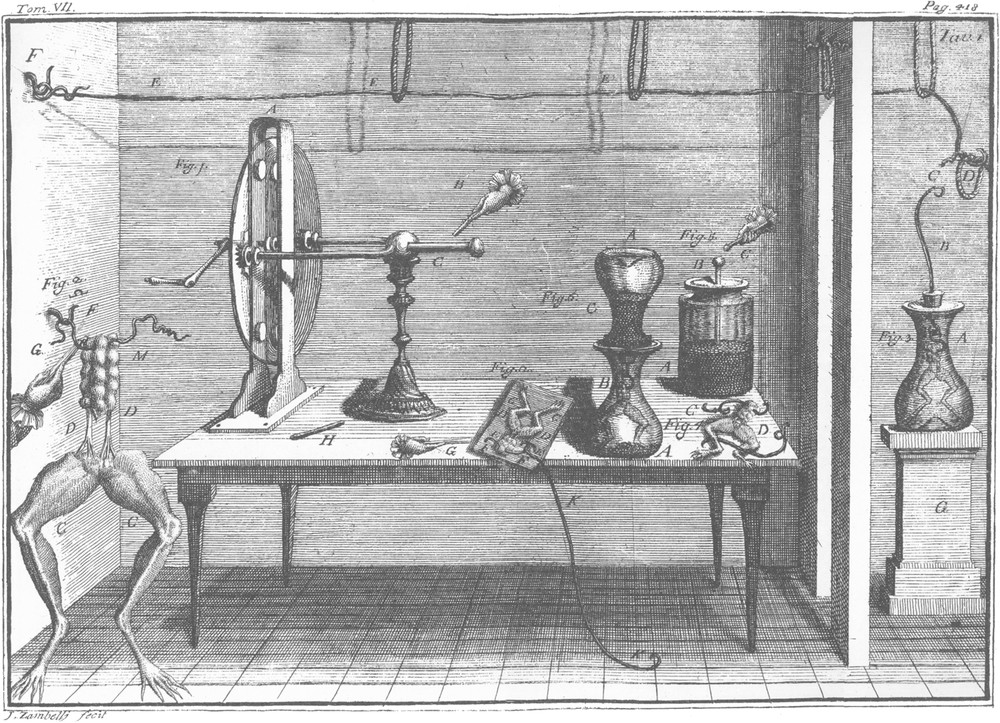
The first plate of the De viribus electricitatis in motu musculari. Beside various frog preparations, notice, respectively on the left and on the right on the table, an electric machine and a Leyden jar (from [1]).
4 Galvani's scientific personality and endeavours
In addition to his published texts, Galvani's electrophysiological researches can be followed through a vast number of manuscripts containing the laboratory protocols in which he recorded the progress of his experiments and, from the three memoirs that he wrote between 1782 and 1787 (and left unpublished, see [35]). From this material, and from the attempt to situate his endeavours within the scientific and cultural contexts of his epoch, Galvani emerges as a figure far away from the stereotype of a pioneer incapable of fully developing his experimental and intellectual elaborations. He stands out, on the contrary, as a researcher of high standards, who aims at solving an important physiological problem (the role of electricity in neuromuscular function) with the power of experimental science; he tests contrasting hypothesis with especially designed experiments, repeated and varied in many ways. He published his results in 1791 only after elaborating a model capable of facing the objections of his contemporaries against the possible involvement of electricity in neuromuscular function [1].
There are several characteristics of Galvani's endeavour worthy to be pointed out here. Among these is his extreme attention for the experimental conditions, which he described in great detail in order – as he said – that other scientists, after him, might be able to obtain his own results when performing the same experiments. Galvani designs his setup and modified it continuously, often by building himself some useful apparatus (his various macchinette), with relation to the specific problems he is addressing. Moreover, he shows a fundamental quality of the genuine scientist, the capability to learn from his previous results (both successes and failures) how to proceed further, what needed to be modified in the laboratory apparatus or in the experimental design, which questions must be particularly pursued. This ‘live learning’ had various aspects. Sometimes it resulted from conscious reflection or logical reasoning, sometimes it was a kind of progressive training that enabled him to improve his efficiency and rapidity in making the appropriate experimental decisions.
Although guided in his investigations by hypothesis and theories, Galvani shows a great intellectual freedom from the dogmatic excess of the various scientific ‘systems’ of his epoch. All along his work he has as constant references the hypotheses (and objections) on the mechanism of nerve conduction and muscle contraction proposed by the two main contemporary doctrines: the neuroelectric and the irritability theory. However, he keeps a liberal attitude towards both theories. His final model (the neuromuscular complex as a Leyden jar) keeps the main assumption of the neuroelectric theory: i.e., the electric nature of nerve conduction; however, it tends to place the responsible agent inside the structure of muscle tissue, somewhat in accordance with the irritability theory.
5 The early phase of Galvani's electrophysiological researches
The first annotations of Galvani's experiments are dated 6 November 1780, but he probably started his researches on the effects of electricity on muscle contractions somewhat earlier [36]. The initial experiments concerned the effects of ‘artificial electricity’, that is of the friction electricity produced by electrical machines and stored in capacitors, like the Franklin's square or the Leyden jar (see Fig. 2). The square capacitor, mentioned in the protocol of his first experiment, was more commonly used in this period, probably because, for its shape, it could serve also as convenient support for the frog preparation (the first reference to a Leyden jar appears in the protocols of 6 December 1780). Another device capable of producing and maintaining electric power in Galvani's laboratory was the electrophore (the atypical electric generator invented by Volta in 1775, see [5]), whose presence is recorded for the first time on 7 February 1781. Besides electric machines, capacitors and electrophores (and in addition to various surgical instruments necessary to prepare the frog), Galvani used, in his first experiments, metallic ‘arcs’ (i.e., the tools normally employed to discharge electrical machines or capacitors, see Fig. 2) and metallic wires in order to connect various parts of animal to the electrical source.

A Leyden jar (A) and a Franklin square capacitor (B) with, in both cases, the ‘arc’ used to discharge the device: this was done by connecting the opposite metallic laminas (or ‘armatures’) in which electricity is accumulated. In the case of the Leyden jar, the electricity of the internal armature flows through the ‘conductor’ protruding out of the jar mouth. (From [10], modified.)
Galvani's interests seemed initially limited at ascertaining the impairments induced by strong electric discharges on the neuromuscular system (i.e., how electricity can extinguish ‘muscle force’ or ‘nervous force’). However, the experimental questions he was addressing were of a more physiological character and reflected the debate on the neuroelectric theory and the objections of the Hallerians as it appears. On 22 November, Galvani compared the effects of the electrical stimulus on a frog preparation in which one crural nerve was ligated and the other set free. The procedure was clearly aimed at ascertaining the validity of Haller's objection of the different effects of ligature on conduction of nerve signal vis-à-vis the passage of electricity along nerve trunk. Three days later, he started verifying another important nerve property implied in the neuroelectric theory, i.e., the ability of the nervous tissue to conduct electricity more or less freely. The results of these experiments convinced Galvani that electricity could flow across the nervous tissue, but its passage may not be so easy and free as it is across metals or other highly conductive bodies. This conclusion fits with some assumptions of the supporters of the neuroelectric theory. In order to face the objections of the Hallerians on the possible spread of the electrical fluid from nerves to the surrounding conductive tissue, they assumed that electricity had a great affinity for the nervous fluid and thus was not free to escape outside nerves.
Another significant result of Galvani's initial experiments was the demonstration that contractions could be evoked by extremely weak electric stimuli, such as those provided by a flat capacitor or a Leyden jar almost completely discharged (so as not to give any clear-cut electric sign, such as sparks, ‘electric noise’, etc.).
Galvani's main aim in starting his research was evidently to verify the neuroelectric theory and its implications (this appears from some annotations in his journal of experiments and is explicitly declared in the introduction to the 1782 memoir Sulla forza nervea, “On the nervous force” [37]). However, in his initial experiments, he seems to consider artificial electricity simply as a way to excite nerves and muscles and to produce contractions, and thus as an external agent of the phenomenon. The possible involvement of an electric fluid internal to animal body emerges more clearly during the researches carried out at the beginning of 1781. It becomes dominant after the fundamental ‘chance observation’ of 26 January. As described at the beginning of the De Viribus, this observation became the starting point of all further investigations, and its fortuitous character is emphasised: a frog preparation contracts when somebody (“my wife or other” he notes in the experimental protocols) extracts the spark from an electrical machine which is “not connected” to the frog by any type of conductor [36 (p. 254)].
In subsequent experiments, Galvani tried to ascertain the circumstances in which the phenomenon occurred and realised that an essential condition was somebody touching an animal nervous tissue with a conductive body (such as “metal, fingers or other”) at the moment when a spark flies from the distant electric machine. No contraction occurred if nervous tissue was touched with an insulating body (“glass or old bone”, he annotates). Moreover, contractions were less easily produced if the conductive body was put in contact with muscles rather than with nerves. This observation appeared to be at odd with the doctrine of the irritability, which stipulated that a force responsible for contraction was intrinsic to muscles.
During the following months, Galvani varied the conditions of the experiment in an astounding number of ways. Most of the experiments made up at the beginning of 1783 appeared to be variations of the ‘spark experiment’ or were carried out to ascertain the underlying mechanism. During this period, Galvani showed a particular ability to develop new and more complex experimental arrangements, sometimes based on particular tools appropriately designed by him (as for instance the various recipients – or caraffe – used to isolate the frog preparation from external mechanical or corpuscular influences). The prepared frog progressively became a part of elaborated spatial dispositions involving electrical sources, metallic wires, sometimes the experimenter himself. This complexity stimulated Galvani to identify the circuit followed by the electric fluid to produce the contractions, in order to get an insight into the mechanisms underlying them. Although preserving his special animal statute, the frog is progressively integrated in a physical complex, both material and logical, and this tends to make Galvani's research more effective and modern.
The phenomenon of contraction produced by distant sparks directed Galvani's attention toward the frog preparation as the place where “a most subtle fluid” is present, which is “excited by the push, by the vibration, by the impulse of the spark” [38 (p. 18)]. However, the electric nature of the fluid responsible for muscle contraction seems to be contradicted by the difficulties encountered in eliciting contractions with electrical stimulation applied to the muscle. One of the predictions of the neuroelectric theory was indeed that muscles should contract in response to direct electric stimulation, because the physiological agent of contraction would be the electricity brought to muscles by nerves.
As frequently happens in experimental science, particularly in new research fields, phases of enthusiasm and deception alternate during Galvani's studies. At the end of 1782, he wrote his memoir on the nervous force in order to summarise the results of two years of experiments and to derive a coherent picture from them. The phrase ‘nervous force’ was a non-committal expression to designate the nervous agent in a period in which there was uncertainty about its nature and role in muscle contraction. Galvani's choice reflected his difficulty in elaborating a theory capable of accounting for the involvement of electricity in neuromuscular function in a comprehensive way. It reflects, moreover, his convincement that electricity acts mainly on nerve, rather than on muscle, and thus in some way it marks Galvani's distance from the doctrine of irritability.
Most of the memoir is devoted to a description of the conditions in which artificial electricity is effective in producing muscle contractions, without any definite attempt to propose a mechanistic explanation of neuromuscular physiology. Two points emerge in a particularly clear way. First the necessity that electrical stimulus be rapid and of sudden onset and offset, since no contraction is usually observed when frog preparation is connected to an electric machine, continuously operated so that “the electric fluid flows constantly” and in great quantity. Sparking electricity is particularly effective, because its time characteristics suit the temporal requirements of nerve excitability. What really matters, however, is not the spark itself, but the impulsive character of the stimulus, its rapidity, its action like a sudden stroke, or quick vibration.
The other important aspect pointed out by Galvani points in his 1782 memoir concerns the relation between stimulus intensity and strength of the contractile response. Although contractions become stronger with more intense electrical stimuli, there is no simple proportionality. Contraction appears only when the stimulus intensity exceeds a certain minimal value. A further increase of its strength results in stronger contractions, but only within a given range. More intense stimuli do not result in stronger effects.
These properties pointed to the animal preparation as the site of the ‘force’ responsible for the contractile response. In other words, the electrical stimulus was not the effective agent of contractions, but only the ‘exciter’ capable of putting in motion an internal force responsible for them. Galvani's ideas fitted the conceptual framework of the irritability, which focussed on the relative lack of direct relation between the intensities of the stimulus and of muscular response. However, he tended to situate the internal force aroused by the external electrical agency in the nerves rather than in the muscles (as implied by the Hallerian paradigm), thus showing his independence from any intellectual dogmatism.
Another aspect of neuromuscular physiology that Galvani points out clearly in his memoir on the nervous force is the recovery of excitability to the electrical stimulus that can be obtained in preparations fatigued by repetitive stimulations, if the preparation is left at rest. Also this observation suggests that the response of the animal is mainly the expression of an internal agency, of a ‘force’ that may become exhausted after prolonged stimulation.
6 The experiments with metals
In the memoir of 1791, in which Galvani first publicly announced his discovery of animal electricity, the description of the results is organized in three parts devoted respectively to experiments on artificial, atmospheric, and animal electricity [1]. The impression one gets is of a logical and temporal sequence of experiments carried out at rather defined and regular paces. The protocols, however, suggest a different view. Galvani carried out the experiments with artificial electricity from November 1780 up to February 1783, and he passed to the investigation of the effects of atmospheric electricity only in April 1786 (that is four years later); in September of the same year he began the last phase of his experiments, largely based on the use of metals and leading to the notion of animal electricity. It appears, moreover, that the passage from the second to the third phase is relatively poorly defined in the experimental protocols compared to the published memoir. This is only one of the occasions in which the picture of the events recorded in the experimental protocols contrasts with that emerging from the published writings (see [10,36]).
In the period 1783–1786, Galvani undertook a series of physicochemical investigations on animal bodies, in the line of the works on the ‘airs’ that were attracting the attention of many eminent scientists of the century (and which would eventually culminate in the chemical revolution of Lavoisier) [39]. As noted by Marco Bresadola, these experiments were probably aimed at investigating if a principle different from electricity might underlie neuromuscular function (see [10]). This research line appeared worthy to pursue to Galvani, particularly in view of the deceptive character of the results obtained in his previous studies on the electrical nature of this principle.
When Galvani eventually came back to electrophysiological studies in 1786, he profited of some results obtained during this physicochemical period: in particular, the observation that nerve tissue produced a great quantity of “animal inflammable air” (i.e., hydrogen), and were thus made of an abundant “oily substance”. This finding would eventually justify the model of the nerve as made by a central conductive core wrapped by an electrically insulating matter, a basic assumption of the Leyden jar hypothesis of neuromuscular physiology.
The experiments on the effect of atmospheric electricity described in the second part of the De viribus were important for Galvani because they proved that effects similar to those of artificial electricity could be produced with electricity from natural source, i.e., that associated with thunder and lightning. The illustration of these experiments, with the frog preparation on a table in Galvani's home terrace and long wires pointing toward the sky, has become famous (also because it has been a sources of inspiration for various cinematographic versions of Frankenstein, see Fig. 3). In these experiments, Galvani proved that electricity from a stormy weather could produce muscle contraction as the artificial electricity, and appeared to do so by following “the same laws”.

Galvani experiments with the atmospheric electricity of a stormy day as illustrated in the second plate of the De viribus electricitatis in motu musculari (from [1]).
The experiments described in the third part of the De viribus begin with a chance observation made on September 1786, in the course of the investigations on atmospheric electricity: a frog preparation with a metallic hook inserted in its spinal cord was suspended on the iron fence of the balcony on a day that is described as clear and calm in the De viribus (but appears much less so from the protocols). The purpose was to ascertain if the weak atmospheric electricity of a non-stormy day could also stimulate contractions. This was in line with the contemporary interest in small degrees of electricity (and fitted with the extreme frog sensitivity to weak electrical stimuli already noticed by Galvani). The episode, as narrated in De viribus, is also particularly famous because was frequently illustrated in physics textbooks of the 19th century. Frog legs stayed quiet for a long while. Eventually Galvani (or possibly his nephew Camillo, according to the protocols) started manipulating the preparations and something unexpected happened: contractions appeared when the metallic hooks were pushed toward the iron bars of the railing, with no relation whatsoever with atmospheric events. To exclude the intervention of atmospheric electricity, the experiments were repeated within in “a closed room”, with the same success. What was needed for getting contractions was simply to put muscle and nervous tissue in contact through a metallic conductor (particularly through a ‘metallic arc’). Nothing happened by using an insulating body or if the metallic contact was interrupted by the interposition of a non-conductive material.
Contractions did not appear if an insulating body was used for the connection, including “glass, rubber, rosin, and well dried stones or wood”. The different efficacies of various metals correlated with their conductive power. Water and electrically-conductive liquids could also be used, although they were less active than metals. An effective circuit could be formed by a chain of persons connected together and touching the nerve and muscle of the animal with a metallic body. As Galvani wrote in De viribus, these experiments led him to suspect the presence, between nerves and the muscles, of “a flow of an extremely tenuous nervous fluid [....] similar to the electric circuit which develops in a Leyden jar” [1 (p. 378)].
As in the first phase of his investigation on artificial electricity, Galvani now performed a great number of experiments and varied their design with great efficacy and imagination. Some of these experiments were described with a richness of visual detail, as a kind of entertainment for the reader. This is the case of the frog preparation that seems to jump because the contractions of the leg produced by the metallic contact results in a break of the circuit, which is re-established at the moment the leg relaxes, thus renovating the contraction.
Compared to the period of the experiments on artificial electricity, Galvani starts now from a safer ground in his attempt to demonstrate that the electric nature of the fluid is responsible for these effects. In the experiments with metals, there is no evident source of electricity external to the preparation: the principle responsible for the contraction is thus very likely internal to the animal; moreover, since this principle is capable of circulating through various material bodies following the same laws of electricity, it is logical to assign to it an electrical nature.
Before conceiving that the electrical source was internal to animal preparation, Galvani considered, however, the possibility that electricity could originate from the metals used to connect nerve and muscle tissues. However, on the basis of a series of experiments and of the known laws of physics, he excluded such possibility.
I shall not describe here Galvani's experiments and considerations on this point, which would be the crucial argument of the famous controversy with Volta (who would elaborate the theory of electromotive power of metallic contacts, which eventually would lead him to the invention of the battery (see [10]). Nor shall I describe in detail other experiments, important for Galvani's elaboration of his final model of the neuromuscular complex as a Leyden jar. I will concentrate instead the following of this paper on the logical and experimental itinerary leading Galvani to his conclusive model, starting from the moment that he considered as safely established the electric nature the neuromuscular fluid. I shall try to do this mainly by analysing the various texts that Galvani wrote during this period. There are several reasons of interest for doing this.
For example, these writings show clearly how, in his electrophysiological investigations, Galvani was pursuing a coherent and ‘rational’ explanation of neuromuscular physiology. He could not content himself simply by obtaining novel experimental findings, even if they might appear novel and interesting. No doubt Galvani had a great confidence in the power of experimental science. Not only did he believe that experiments were absolutely necessary for revealing scientific truth, but he was convinced that what happens in experimental conditions has a necessary counterpart in natural conditions. This was explicitly stated in a work published in 1794, where, in relation to contractions produced experimentally, Galvani wrote:
“If, as we have shown, animal electricity produces muscular contractions once set in action by external artifices, it is a requirement of reason that it should produce them also when induced to action also by internal and natural causes; the contractions are indeed the same in both cases for what concerns their essence, and differ only in degree and force; it is of no likelihood that nature would use the said electricity only for the advantage and pleasure of the experimenters, and not for the benefices of animal economy.” [40 (p. 124)]
Nevertheless, Galvani thought that experimental findings were not per se a guarantee of scientific credibility; moreover, they could not provide secure grounds for useful medical applications if they were not integrated in a logical model. Besides corresponding to the experimental observations, this model should be capable of explaining the mechanisms of the phenomenon on the basis of the laws of physics and of physiology. In this respect, Galvani was under the influence of Marcello Malpighi and his conception of ‘rational medicine’, a scientific legacy that was still alive at the ‘Istituto’.
7 Electrophore and tourmaline stone: on the way to the ‘minute Leyden jar’
From the moment that he became convinced that the electricity responsible for muscle contraction was intrinsic to the animal organism, Galvani entered in an extremely interesting and exciting phase of his research. The contractions obtained through a metallic contact between nerve and muscle led him to suppose the existence of a flow of electricity from nerve to muscle through a metallic conductor “in a way not different from that in which in the Leyden jar can be obtained a passage of electricity from the external surface to the internal one and vice versa” [37 (p. 166)]. The mention of the Leyden jar as a mental tool to represent the hypothetical electric circuit between nerve and muscle, although alluded to in the De viribus, appears first in an unpublished memoir that Galvani wrote at the end of October 1786, i.e., a few months after his first experiments with metals (see [37 (pp. 162–193)]).
In Leyden jars – as Galvani notes – electricity flows because of the presence of two distinct forms of electricity, positive and negative, situated respectively in the inner and outer metallic plates (or armatures) of the jar. The problem was to identify in the animal tissue the site of this “double and opposite electricity, i.e., positive, as it is said, and negative”. After a series of experiments, he arrives to the firm conclusion that: “no doubt can subsist that, out of the said two forms of electricity, one is situated in the muscle and the other in the nerve” [37 (p. 176)]. However, in spite of the important evidence for this conclusion accumulated in this period, and amply discussed in the memoir of 1786, Galvani eventually decided not to publish this memoir. He also decided not to publish another memoir, one dated 16 August 1787. It is only in 1791, more than ten years since the beginning of his studies, that a text will appear publicly, announcing the discovery of animal electricity.
Why did this happen? A possible response to this question can be found by following the itinerary that led Galvani, from the initial convincement of the localization in nerve and muscle of the positive and negative electricity involved in muscle contraction to his final model of the neuromuscular system as a “minute animal Leyden jar”.
There is an important difference between the De viribus and the previous inconclusive attempts made by Galvani to publish his results. In the final memoir, and only in it, he provides a model that appears capable of accounting in a ‘rational way’ for the problem that he was eagerly investigating for so many years: the mechanism whereby electricity is involved in neuromuscular function. It appears evident that, for Galvani, the identification of the localization of the two forms of electricity in nerve and muscle, in spite of the experimental evidence for its support, did not provide a comprehensive explanation for neuromuscular physiology. It was difficult, on this basis, to propose a mechanism whereby, in physiological conditions, electricity would flow to produce muscle motion. It was difficult, moreover, to envision how an electrical disequilibrium could exist between nerve and muscle in spite of the conductive nature of body fluids. Indeed, it appeared physically impossible that an electric difference exists between two different parts of a conductive body.
This argument (central to the objections of the Hallerians to the neuroelectrical theory) was invocated by Galvani himself in his 1786 memoir. It was used to exclude that the positive and negative forms of electricity could be located inside the metal of the arc used to connect nerve and muscle. Galvani was, however, aware of a possible exception to this rule. As he wrote, the presence, inside a conductive body, “of a double polarity, one positive and the other negative, this is a fact that the physicists admit for tourmaline”. However, he noticed, “this appears not to happen for any other metal” and thus concluded that double electricity could not be situated inside the substance of the metallic arc.
The localization of electricity inside animal body being thus, in Galvani's opinion, firmly grounded, he considered afterwards various possibilities (as he also narrates in the De viribus) as to the specific localisation of the positive and negative electricity. In particular, he alluded to the possible localization of both forms of electricity inside muscle tissue. This might appear likely, since, as he wrote, “there is in muscles a big quantity of substance, which for its nature may be apt to develop and hold electricity, in spite of the presence inside it of conductive matter.” And he continued on by saying: “this is not unlike what we saw happening in electrophores which are made of analogous substances. If that were to happen, it would be perhaps justified to call muscles animal electrophores [37 (p. 169)] (italics is mine).
This passage is interesting because it alludes to a first physical model of the neuromuscular physiology. The electrophore was made by the assembly of disks of different substances, some conductive and some insulating; it could thus offer some visual suggestion to Galvani as a model for a biological source of electricity in view of the striated and (thus apparently heterogeneous) structure of muscles. However, in his 1786 memoir, Galvani did not elaborate on this possibility, and concluded that the two forms of electricity (i.e., positive and negative) should be localised, one in muscles, and the other in nerves. As a matter of fact, the electricity production in electrophore depended on a complex and coordinated series of moving manoeuvres whose occurrence was difficult to envision in muscle tissue.
In the other unpublished memoir dated 1787 [37 (pp. 190–212)], Galvani dedicated an ample reflection to the problem of the possible localisation of the intrinsic electricity, which is of particular interest because it offers an important cue to his itinerary toward the final model of 1791. The argument is now centred on the analysis of an electrical tool, already considered en passant in the text of 1786: the tourmaline stone.
Tourmaline was interesting for Galvani for several reasons. It was able to produce unequivocal signs of double electricity upon heating; however, unlike most other electric devices (and similar to the prepared frog), it did not produce any muscle shock when touched by the experimenter. For him there could be other important analogies between the neuromuscular complex and tourmaline, as he wrote in this passage:
“Our electricity has much in common with that of tourmaline stone, for what concerns its localization, distribution, and property of parts. In this stone we observe indeed a double matter, transparent and reddish the first one, opaque and colourless the other; this second one is arranged in stripes. Nobody ignore that nerves are laid down between the layers of muscular fibres, and when these ones are devoid of blood they are transparent, while nerves are opaque. In tourmaline the poles of the double electricity appear to be situated on the same opaque line; so it is in muscles in the same direction. The double electricity of tourmaline does not belong only to the entire stone, but to every fragment. Similarly, in muscles, the admitted double electricity does not belong only to the entire muscle body, but to every part of it.” [37 (p. 194)]
Tourmaline was now invoked by Galvani as a positive reference for a possible physical analogy to the neuromuscular system, as a model, both operative and structural, capable for accounting for the generation of electricity inside the organism. Tourmaline had been studied particularly by Franz Aepinus, who made important analogies between its power and magnetism. As in the case of a magnetic body, the attracting properties, and the capability of generating a double pole, did not reside in the external aspect, nor in the way of cutting it, but in the internal structure and the essential constitution of the stone [41]. Indeed, besides other similarities between the electric behaviour of tourmaline and neuromuscular tissue, Galvani noticed that animal electricity showed its effects both in the entire muscle and in “every part of it recently separated from the animal” [37 (p. 195)].
As in the case of the muscle as “animal electrophore”, Galvani was particularly sensitive to visual suggestions and he now invoked a visual similarity between the muscle, with its striated and heterogeneous aspect, and tourmaline. He suggested that, inside muscle structure, electricity might arise from the contact between a muscle fibre and a nerve fibre. In this way he kept his previous idea of muscle and nerve as the site of the double electricity, but moved his attention form the macroscopic to the microscopic level.
Notwithstanding its attractiveness, the tourmaline analogy was eventually abandoned by Galvani. Although it could provide an insight into the mechanism of production of animal electricity, it did not easily allow him to conceive, in a physically reasonable way, how electricity could be involved in the process of nerve conduction and muscle contraction. Furthermore, Galvani had noticed an important property of animal electricity that pointed toward a different physical instrument as a model of neuromuscular function: the Leyden jar. He had discovered that contractions were more vigorous (and could be excited more easily) if muscle and nerve tissues were wrapped with a thin metallic sheet (silver, brass, golden, orichalc, and particularly tinfoil). Galvani described this power of metallic sheets in both the 1786 and 1787 memoirs; he mentions a series of experiments in which the sheets were wrapped in various ways around muscles, spinal cord, isolated nerves and even around the exposed brain.
There is an important linguistic difference between the two memoirs: in the first one, the metallic sheets are indicated exclusively as laminas or foils (lamine or fogli), whereas in the second memoir a different phrase appears from the outset in relation with these experiments: “metallic armature”. In the electric terminology of the epoch, “armature” was the term commonly used to designate the thin laminas coating the internal and external glass surface of the Leyden jar (see Fig. 2). They were conceived as the sites in which positive and negative charges accumulated due to the capacitive effect of the glass dielectric. Galvani's frequent use of this term (armatura in Italian together the derived verb armare, ‘to arm’) in the 1787 memoir strongly suggests that, in the period 1786–1787, his attention was moving to the Leyden jar as a plausible electrical model of neuromuscular function. The word ‘armature’ was also used to designate the metallic laminas coating the surfaces of Franklin's square type capacitor. However, the square capacitor is mentioned infrequently by Galvani in his unpublished memoirs of 1786 and 1787 (and in the De viribus, in spite of its almost constant use in the course of the experiments (as documented in the laboratory protocols). Very likely, because of its simple shape, it did not exert any special visual suggestion as a model of the involvement of electricity in neuromuscular function.
The Leyden jar represents a fundamental passage of Galvani toward his conclusive model of the neuromuscular system. In addition to its operative characteristics, it had a strong visual attractiveness, as Galvani recognised in an explicit way: the frog leg, with the nerves emerging from the muscle tissue, bore a strong visual resemblance to the Leyden jar with its metallic conductor protruding from the jar mouth (see Figs. 1 and 2).
In the Leyden jar, the discharge was normally obtained by establishing a contact between its outer armature and its ‘conductor’ (i.e., the metallic wire connected to the inner armature); however, the double electricity was not accumulated between the outer armature and the conductor, but between the external and internal armature. If the neuromuscular complex also resembled the Leyden jar from an operative point of view (as the effect of armatures suggested), then electricity should be accumulated in its entirety (i.e., both its positive and negative form) in the muscle rather than between the muscle and the nerve (as Galvani had assumed initially). This elaboration is explicitly expressed in the fourth part of the De viribus, where Galvani writes:
“Even though in order to obtain muscle contractions it is normally necessary to connect one extremity of the arc to the nerves isolated from muscles, it does not follow that nerves have importance for an electricity pertaining to them, i.e., [it does not follow] that one electricity is situated in nerves and the other in muscles; in a similar way in the case of Leyden jar, although usually one extremity of the arc is applied to its external surface and the other to its conductor in order to have the passage of electricity from the one to the other of the two surfaces; nevertheless one cannot deduce that the electricity manifested by the conductor is proper to it and different from that which remain in the bottom of the jar; it is, on the contrary, well known that electricity pertains entirely to the charged inner surface, and that both electricity, in spite of their opposite polarity, are situated in the same jar.” [1 (p. 395)]
But where and how could both forms of electricity be situated inside the mass of the muscle without violating the law of the physics? Where could an insulating matter be found inside muscle? Indeed Galvani had already considered this possibility in the 1786 memoir when he spoke of the presence of “a big quantity of substance, which for its rubbery nature, may be apt to develop and hold electricity, in spite of the presence inside it of conductive matter”; he had then invocated the electrophore as a possible model of the neuromuscular system.
After the 1787 memoir, Galvani had with tourmaline another model capable of suggesting how muscle tissue, with its striated and fibrous aspect, might store electricity inside it. There were three important further logical steps for Galvani in order to pass from the ‘tourmaline model’ to the final ‘minute animal Leyden jar’ (see Fig. 4). One was to conjecture where an insulating substance could exist in muscle, at a microscopic level. As Galvani speculated in the De viribus, a likely possibility is that this substance is situated at the surface of separation of the interior and the interior of every muscle fibre:
“It is even more difficult that the existence of a duplex electricity in every muscular fibre itself could be denied if one thinks not difficult, nor far from truth, to admit that the fibre itself has two surfaces, opposite one to the other; and this from consideration of the cavity that not a few admit in it, or because of the diversity of substances, which we said the fibre is composed of diversity which necessarily implies the presence of various small cavities, and thus of surfaces.” [1 (p. 196)]
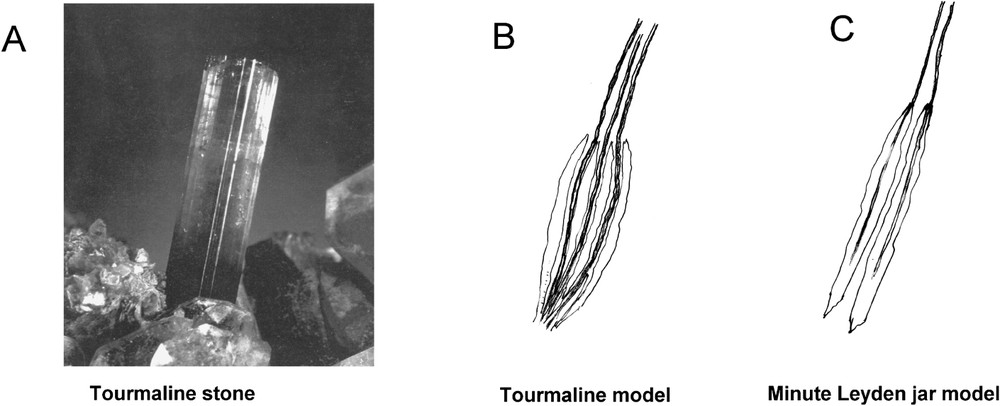
The tourmaline stone (A) and a modern reconstruction of Galvani's model of the neuromuscular system as conceived in the memoir of 1787 (B), and in the final version of the ‘minute animal Leyden jar’ (C). In (B), the nerve fibres are situated between the muscle fibres, while in C a single nerve fibre penetrates inside a single muscle fibre (from [10], modified).
With this bold conjecture, Galvani puts the electric disequilibrium between the interior and the exterior surface of an excitable fibre, according to the laws of physics, and thus can face the fundamental objection of Hallerians against neuroelectric theory. This is fundamental assumption, which would recur with Bernstein's membrane theory of bioelectric potential in 1902 [42]. Galvani put forward his conjecture on an epoch in which cell theory was still to come, and the concept of fibre was the only available microscopic approximation to the elementary constitution of living tissues.
Having situated the two forms of electricity at the two faces of the surface delimitating the muscle fibre (and having attributed an insulating character to this surface), Galvani is in the condition to make his second important step. He assumes that the nerve fibre penetrates inside the muscle fibre like the conductor of the Leyden jar penetrates inside the jar, in order to allow for a possible outflow of the internal electricity. Apparently this is just a small rearrangement of the mutual relation of nerve and muscle fibre with respect to the tourmaline stone model (that Galvani evokes in the De viribus soon after conceiving the insulating nature of the separation between the interior and the exterior of muscle fibre) (see Fig. 4).
Eventually the final step by which Galvani envisions how could electricity, flowing from the interior of muscle to nerve fibre, could be delimitated to this in spite of the conductive character of the humours surrounding nerves. Galvani makes reference to his previous physicochemical studies showing a particular richness of oily matter inside the nervous tissue: he assumes that this oily matter forms an insulating sheet around the central conductive core of nerve fibre. With this conjecture Galvani is able to circumvent another fundamental objection of the adversaries of the neuroelectric theory that he enunciates explicitly in De viribus in the form of an unsolvable dilemma:
“As a matter of fact, either nerves are of an idioelectric [i.e., insulating] nature, as many admit, and they could not then behave as conductors; or they are conductors, and were this the case, how could they contain inside them an electric fluid is contained, which would not spread and diffuse to nearby parts, with a sure detriment of muscle contractions.” [1 (pp. 398–399)]
The dilemma is solved in a clear way in the immediately subsequent passage of the De viribus:
“But this difficulty can be easily faced by supposing that nerves are hollow in their internal part, or at least made up of matter apt to the passage of electric fluid, and exteriorly [made up] of an oily substance or of another matter capable of hindering the passage and the dispersion of the electric fluid which flows inside them.” (Ref. [1 (p. 399)])
The muscle fibre delimited by an insulating substance that separates the two forms of electricity at its two faces and the nerve fibre penetrating inside the muscle fibre with its inner conductive core and its insulating surface: this is the final and conclusive model of the “minute animal Leyden jar” by which, more than two centuries ago, Galvani laid down the foundation of modern electrophysiology.
Within the framework of his model, Galvani conjectured (in the De viribus) that electricity could be discharged in physiological conditions through the insulating substance of the nerve fibres in order to produce physiological effects. Contractions could result directly from the “extremely fast passage” of electric fluid capable of causing “a violent and peculiar attractions of the particles composing them”. Or the electric flow could “exert an irritation and a mechanical stimulation on nerve or muscle fibres, such as to excite their so-called irritability”. It could also act in other unknown ways. Galvani thus kept an uncommitted attitude toward both the irritability and neuroelectric theory. He did so mainly because, in the absence of conclusive evidence, he did not wish to commit to a particular (and possibly inconclusive) interpretation of muscle motion, while he presented his main discovery, that electricity is involved in neuromuscular function.
8 From Galvani to the ‘H–H’ model
Galvani's ‘minute Leyden jar’ model differs in many respects from the modern understanding of neuromuscular physiology. For Galvani, electricity is accumulated exclusively between the interior and the exterior of the muscle fibre, with the nerve fibre playing only the role of conductor of muscle electricity. The core conductor model of Galvani's nerve fibre anticipates the cable model, which in modern electrophysiology accounts for the passive conduction of electric signal in nerves. However, in modern views, the fundamental and distinctive property of electric conduction in nerves is not the passive diffusion of electric signal, but the regenerative mechanism whereby nerve signal spreads along nerve fibres without attenuation, in spite of the extremely high longitudinal resistance of the inner conductive core.
More than a century after Galvani, the regenerative character of nerve signal transmission appeared in electrophysiological researches, mainly due to the work of Keith Lucas and Edgar Douglas Adrian, and its underlying mechanisms was explained in 1952 by Alan Hodgkin and Andrew Huxley, who studied the squid's giant axon [43–46].
In an ideal way, the Hodgkin–Huxley studies concluded the historical cycle initiated by Galvani in the second half of the 18th century. According to the model derived from these studies (and fully developed in contemporary electrophysiology – the famous H–H model), electricity is accumulated in a condition of disequilibrium at the two sides of the plasma membrane of nerve fibre, and it is ready to flow. However, it cannot pass across the membrane because the membrane is largely impermeant to the flow of electrically charged ions, due to the hydrophobic character of its lipid bilayer. Ions can permeate the membrane only through specialized protein pores (ion channels), which, in resting conditions, are largely in a closed state. In order to get ions channels open, it is necessary to move the resting membrane potential (interior negative with respect to the extracellular compartment) in a positive direction (or to ‘depolarise’ the membrane according to the current terminology).
Involvement of electricity in ion channels opening represents the second topological role of electricity in the generation of the nervous signal. Ionic flow caused by channels opening results in a further depolarization and thus in a further opening of ion channels and, consequently, in a further passage of ions. The process eventually leads to the discharge of a full blown nerve impulse according to a ‘regenerative’ mechanism know as the Hodgkin cycle. The process, initiated in a zone of the fibre, acts as a trigger for the nearby zone, thus giving birth to an impulse in that zone (and so on). Consequently nerve signal propagates without attenuation in long and thin fibres in spite of their extremely high longitudinal electric resistance (amounting sometimes to more than millions of millions of ohms). The phenomenon resembles the diffusion of the ignition in a train of gun-powder, according a famous metaphor developed by Lucas and Adrian at the beginning of the 20th century [43,44].
Besides revealing the mechanism of one of the fundamental physiological process of animal organisms, the Hodgkin–Huxley studies also allow for a better comprehension of many aspects of the story of Galvani and his frogs. In particular, they allow us to understand why the opposition between Galvani and Volta in their interpretation of the experiments with metals seemed to lead to a dilemma.
For Galvani, a metallic arc connecting nerve and muscle caused contractions because it allowed for the discharge of electricity accumulated in a condition of disequilibrium inside animal tissues. For Volta, having noticed the particular efficacy of arcs made of two different metals, it was assumed that the electric disequilibrium was produced by the metallic contact: the contraction of the prepared frog would be simply a response to external electricity.
Both scientists were able to obtain experimental evidence in favour of their respective hypothesis. In 1794, Galvani produced contractions by directly connecting nerve and muscle in the absence of any metal; and in 1797 he could induce contraction in two separate frog legs by using the nerve of one preparation to connect two points of the nerve of the other (thus avoiding any heterogeneous contact) [40,47]). In contrast, in 1796, Volta could demonstrate the ‘electromotive’ power of the heterogeneous contact between two metals by using a physical instrument, in the absence of the frog preparation [4 (pp. 391–447)].
Both Galvani and Volta thought they had discovered the effective cause of electric flow in experiments using metals; it appeared thus unreasonable to invoke two different causes for one effect. But this is one of those cases in which, as Galileo asserted four century ago, “nature operates in a way beyond our thinking and contrivance” [48 (p. 96)]. In the experiments with metals, electricity flew because it was in a condition of disequilibrium inside animal tissue (as Galvani assumed); however, an external electric stimulus (provided by the electricity of the bimetallic contact discovered by Volta) was normally necessary to allow for its flow (by causing – as we now know, but Galvani and Volta necessarily ignored – an opening of membrane ion channels).
The modern understanding of membrane electrophysiology also helps to shed a light on the apparently mysterious coincidence, whereby the electromotive power of bimetallic contact (leading eventually to Volta's invention of the battery) emerged in connection with Galvani's discovery of animal electricity. The potential generated at the contact between two different metals is generally less than 1 V. In the second half of the 18th century, the most sensitive electroscopes were unable to detect potential differences smaller than about 100 V There was, however, an important exception: a “very exquisite animal electroscope”, the prepared frog of Galvani. This was because nature (in facing the fundamental problem of electric conduction along thin nerve fibres of high internal resistance) was obliged to contrive the mechanism of voltage-dependence of ion channel opening with an extremely high amplification: the overall gain of the ‘gating mechanism’ of ion channels being of the order of 100 000.
This is why Volta could detect his “metallic electricity” in 1792 by using Galvani's frog preparation. More than ten years before, the same sensitive animal apparatus had been responsible for the frog leg contractions evoked by the sparking of a distant electric machine: an experiment, which, as Galvani wrote at the beginning of the De viribus stimulated in him “an incredible curiosity”, such as “to explain the mystery of the phenomenon”. Galvani did not succeed in fully accounting for the mechanism of nervous conduction. He was, however, the first of a long chain of great scientists who, in the course of two centuries, investigated this extraordinary process, and eventually succeeded in explaining its ‘mystery’.
In the period going from Galvani to Hodgkin and Huxley, ‘animal spirits’ were definitely discarded from nerve physiology and electrophysiology arose: Galvani was at the inception of this new science and we could now fully acknowledge his merit and justify his pride in announcing in 1791 his discovery of “animal electricity” and the “electric nature of animal spirits”:
“If it will be so, then the electric nature of animal spirits, until now unknown and for long time uselessly investigated, perhaps will appear in a clear way. Thus being the things, after our experiments, certainly nobody would, in my opinion, cast doubt on the electric nature of such spirits […] and still we could never suppose that fortune were to be so friend to us, such as to allow us to be perhaps the first in handling, as it were, the electricity concealed in nerves, in extracting it from nerves, and, in some way, in putting it under everyone's eyes.” [1 (p. 402)]
Acknowledgements
I thank Marco Bresadola, Stanley Finger and Nicholas Wade for critically reading the manuscript.