Abridged version
1 Introduction: role of temperature and stress in graphite genesis
The role of temperature in graphite crystallization is well known. Many XRD studies have been conducted on the transformation of organic matter to graphite during metamorphism; all the intermediary terms from amorphous carbon to well-ordered graphite have been seen [1]. Carbonaceous material (graphite-d3, according to Landis classification [1]) in the lowest grade rocks ranges from virtually amorphous material to material possessing a crude graphite structure. Such material appears to consist of variably spaced carbon layers (average width 3.5 to 3.75 Å), which are roughly parallel to one another. Evidence for incipient two-dimensional order in graphite-d3 is provided by a diffuse 2.13 Å line observed in powder photographs. From graphite-d3, on-going graphitization takes place in three ways: (1) improvement of layer lattice structure; (2) reduction of interlayer spacing; (3) ordering of carbon layers with respect to one another. Thus, with decrease in d-value, sharpness of the 0002 peak increases and interlayer spacing decreases toward an ultimate value of 3.35 Å. The transition from graphite-d3 to graphite-d2 is gradual. Pervasive three-dimensional ordering and uniform graphite interlayer spacing are generally developed concomitantly. However, in material such as graphite-d1A, the interlayer orientation of true graphite may be acquired before the interlayer spacing is reduced to 3.35 Å.
At variance with the role of temperature, the role of strain and stress is less known. A recent experimental study [10] showed that non-hydrostatic stress could induce polymorph transformations and accelerate graphitization speed. It is the purpose of the present study to bring some evidence obtained from the field. Graphite-rich samples have been collected in Lotru valley (Schela formation, Romanian Carpathians) and were examined by X-ray diffractometry, Raman microspectrometry and electron microprobe.
2 Geological and mineralogical data
2.1 Geological data
The studied region belongs to Schela formation of the Romanian Carpathians [3,4] (Fig. 1). The Schela formation is an ensemble of anchi-metamorphic rocks constituted of metaconglomerates, sandstones, pyrophyllitic schists, schists with chloritoid and anthracitic lenses [8]. The Schela formation is localized at the contact zone between the Getic nappe and the Danubian autochthon [2,5,12]. The age of the Schela formation is Liassic [2] or Carboniferous [11]. Cartographic work allowed contouring graphite-bearing rocks, in which the intercalations of quartzitic lenses are present. According to some authors [3,6], the graphite-bearing rocks of this region were formed by metamorphism of terrigenous Palaeozoic formations in the condition ranging from green schists facies to quartz, albite and chlorite facies. The graphite-bearing body has more than kilometrical dimensions and present a tabular form (direction of horizontal 220°N, dip 10°–24° southward). This body has a planar fabric, with poorly expressed lineation (it may be observed only under the microscope where it is marked by the aggregates of micas, chlorite and graphite). Quartzites are disposed in concordance with the foliation plane of graphitic schists and appear as lenses of centimetric dimensions. The orientation of minerals is isotropic. An anisotropy corresponding to bands with different proportions between quartz and carbonaceous material may be observed. Enrichment in carbonaceous material toward the exterior of quartzitic lens may be observed. Fragments of carbonaceous material have larger size in the central part of the lens.

Cartes géologiques : (a) carte géotectonique (d'après la carte géologique à , Institut géologique de Roumanie) ; (b) carte lithologique du secteur de la vallée de la Lotru (monts Parâng, Roumanie).
Geological maps: (a) geotectonic map (after geological map , Geological Institute of Romania); (b) lithological map of Lotru valley area (Parâng mountains, Romania).
2.2 Mineralogical composition
2.2.1 Graphitic schist
Modal composition was evaluated under the optical microscope; chemical composition of minerals was determined by use of microprobe. The composition of graphitic schists is: quartz (31–68 %), micas (11–20 %), daphnite (3–9 %), Fe-chloritoid (2–6 %), pyrophyllite (< 2 %), graphite (10–18 %) and rutile (< 1 %). In pyrophyllite mineral, a small part of the aluminium is substituted by iron. Mica is a solid solution between Fe-celadonite, muscovite and paragonite. Mica and graphite associations are disposed in alternation with quartz bands (Fig. 2). Under the optical microscope, redistribution of minerals alongside the foliation plans may be observed.
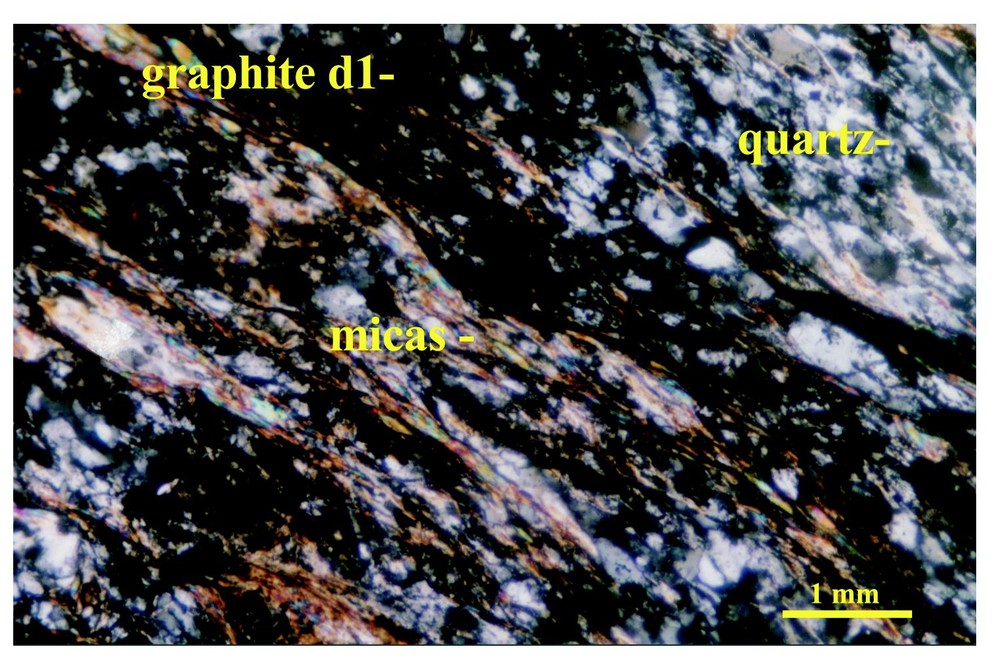
Bandes alternantes de micas, graphite-d1, quartz dans les schistes graphiteux de la vallée de la Lotru (microscope optique, nicols croisés).
Banding of micas, graphite-d1, quartz in the graphitic schists from the Lotru valley (optical microscope, crossed nicols).
2.2.2 Quartzites
Modal composition is: quartz (82–90 %), carbonaceous material (10–20 %), micas (< 1 %). The quartz is disposed in radial columns around the grains of carbonaceous material.
3 Crystallization degree of graphitic material
3.1 Preparation and analytical methods
Sixteen samples from graphitic schist and eight from quartzites containing carbonaceous material have been studied. Separation of carbonaceous material from quartzites was realized under optical microscope and separation of graphite from the graphitic schists was realized by the method described by Tagiri [13]. Samples were hand-picked after crushing at the dimension of 120 μm, a method that does not alter chemistry nor crystal structure. Ten grams of sample were heated on a steambath for 12 h at a temperature of 90 °C in 50 mL-concentrated HCl. Sample was centrifuged and washed with distilled water. To remove the silicate minerals, the sample was mixed with 50 mL 50 % HF, then was heated on a steambath for 12 h at a temperature of 80 °C. Finally, the sample was centrifuged and washed with 37 % HCl and distilled water.
The degree of crystallization of graphite was determined using the XRD (Siemens Kristalloflex D5000) and Raman microspectrometry. Raman spectra were obtained using the 514 nm line of a Coherent Innova 90 Ar+ ion plasma laser as the excitation source set to 0.1–0.2 W. The laser was focused onto the sample through a modified Olympus (BH2-UMA) petrographic microscope to a spot not larger than 3 μm in diameter. The laser line was removed with a notch filter and the Raman signal dispersed onto a liquid nitrogen chilled CCD detector ( pixels) by a Jobin Yvon HR640 monochromator with a 600 groove/mm grating. Samples were placed on glass slides and spectra were collected at room temperature for 200–400 s.
3.2 Results
XRD patterns and Raman shift of first order indicate a clear different degree of crystallization for carbonaceous material in the quartzites lenses and that in the graphitic schist (Table 1, Figs. 3 and 4). According to the classification of Landis [1], the graphite of the graphitic schists of the Lotru valley is a d1-type graphite, and carbonaceous material of quartzitic lenses is a d2-type graphite. Raman shift shows better the degree of crystallization in terms of dimensions of crystalline domains in the a–b plane (0001) [9].
Paramètres du graphite obtenus par diffractométrie des rayons X et type de graphite, d'après la classification de Landis [1] ; d(0002) : distance interfoliaire correspondant aux plans 0002 ; c0=2d(0002) paramètre réticulaire; la correction à partir de l'halite se fait par la comparaison entre et .
X-ray diffraction parameters of graphite and graphite types after Landis classification [1]. d(0002): distance between planes 0002; c0=2d(0002) reticular parameter; correction from halite is made by comparing and .
Échantillon | Correction d'après la halite | d(0002) correcte | Type de graphite | |
1070 (schiste graphiteux) | 26,245 | 0,0001 | 3,370 2 | d1 |
1071 (schiste graphiteux) | 26,390 | 0,0000 | 3,370 2 | d1 |
1073 (schiste graphiteux) | 26,423 | −0,0001 | 3,370 0 | d1 |
1074 (schiste graphiteux) | 26,426 | −0,0002 | 3,369 8 | d1 |
1075 (schiste graphiteux) | 26,422 | −0,0002 | 3,369 6 | d1 |
1077 (quartzite) | 26,428 | 0,0000 | 3,488 7 | d2 |
1078 (quartzite) | 26,476 | 0,0000 | 3,465 2 | d2 |
1082 (quartzite) | 26,418 | 0,0000 | 3,472 8 | d2 |
1083 (quartzite) | 26,444 | 0,0000 | 3,461 5 | d2 |
1084 (quartzite) | 26,439 | 0,0000 | 3,497 2 | d2 |
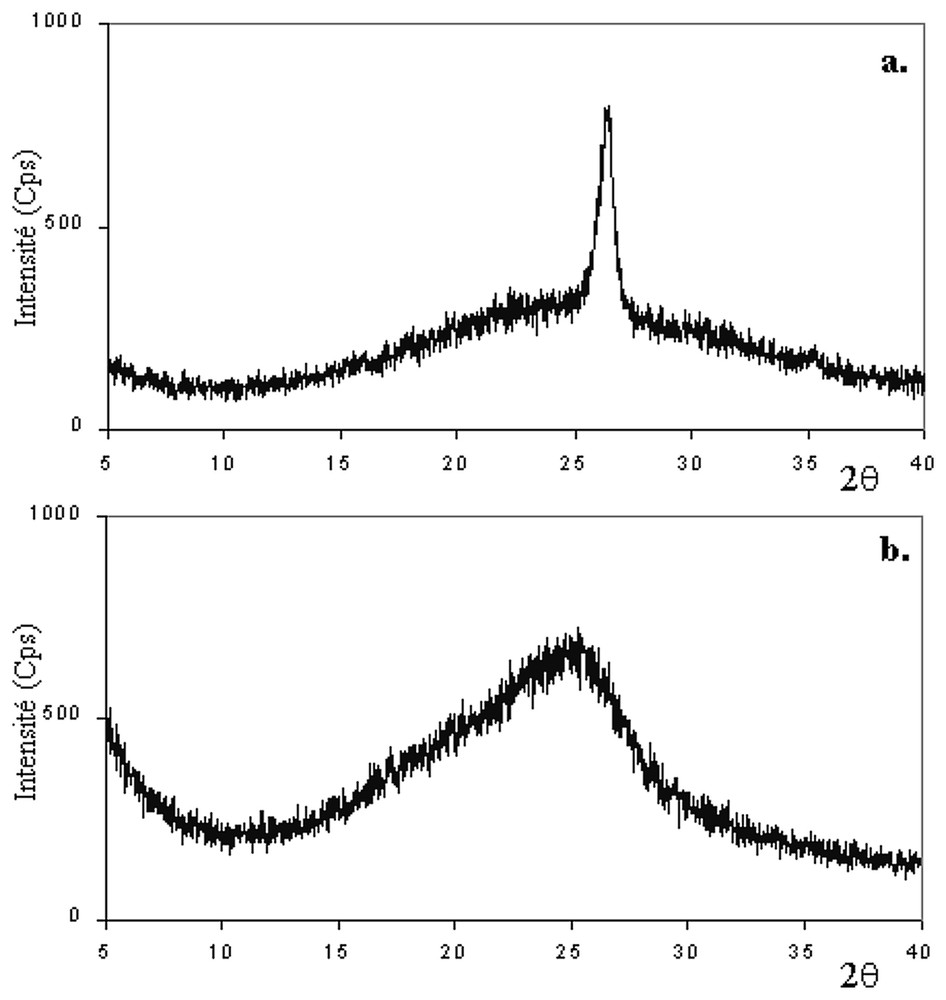
Diffractogrammes de rayons X du matériel carboné des roches de la vallée de la Lotru: (a) graphite de type d1 dans le schiste graphiteux ; (b) graphite de type d2 dans les lentilles de quartzites.
X-Ray diffraction patterns of carbonic material from Lotru valley: (a) d1-type graphite from graphitic schists; (b) d2-type graphite from quartzitic lenses.

Spectre Raman du matériel carboné des roches de la vallée de la Lotru : (a) graphite des schistes graphiteux ; (b) matériel carboné des quartzites.
Raman spectra for carbonaceous material of rocks from Lotru valley: (a) graphite from graphitic schists; (b) graphite from quartzites.
4 Discussion: role of stress in the graphitisation process
X-ray diffractometry (XRD) and Raman microspectrometry show that carbonaceous material is well crystallized in the graphite-bearing rocks, whereas it is less crystallized in the quartzites. In Fig. 4, the crystalline character of graphite within graphitic schist and the amorphous character of carbonaceous material of the quartzites may be observed. The essential difference between the carbonaceous material degree of crystallization of the graphitic schist and of the quartzites is imputable to strain and stress. Thus, in the same thermal conditions, the apparition of discontinuous plane-parallel surfaces allowed to develop the crystalline planar structures of graphite in the graphitic schists. According to Ross and Bustin [10], a carbonaceous substance cannot transform to graphite at temperatures lower than 900 °C if there does not exist any discontinuous plane-parallel surfaces where the crystalline structures of graphite can develop. In the quartzites, the strain is null and, for this reason, carbonaceous material did not evolve to ordered graphite.
During metamorphism, on the planes where prevailed a stronger constraint (together with a possible slightly higher temperature), carbonaceous material could evolve to the level of d1-type graphite. In the quartzitic lenses, the constraint is reduced (the temperature may also be reduced), and carbonaceous material is at the level of d2-type graphite. The decrease of the volume occupied by carbonaceous material is a proof of the evolution of carbonaceous material of biogenic origin toward graphite. An interesting observation refers to the arrangement of quartz around the grains of carbonaceous material (Fig. 5). New radial (columnar) quartz crystals develop while the volume occupied by carbonaceous material is reduced.

Disposition radiale de cristaux colonnaires de quartz autour des grains de matériel graphitique, quartzites de la vallée de la Lotru (microscope optique, nicols croisés).
Radial disposition of columnar crystals of quartz around the graphitic fragments, Lotru valley quartzites (optical microscope, crossed nicols).
1 Introduction : rôle de la température et de la déformation dans la formation du graphite
Le rôle de la température dans la cristallinité du graphite des roches métamorphiques est bien connu. Les études aux rayons X faites sur la transformation en carbone de la matière organique au cours du métamorphisme ont montré divers termes intermédiaires, depuis le carbone amorphe jusqu'au graphite bien ordonné [1]. Ce dernier auteur a proposé une classification du graphite en trois types. Le graphite de type d3 correspond à la matière organique carbonée rencontrée dans les roches de bas degré de métamorphisme, depuis la matière pratiquement amorphe jusqu'à une matière possédant une structure graphitique rudimentaire. Les matériaux de ce type apparaissent constitués de couches carbonées à distances interfoliaires variables (3,5 à 3,75 Å), qui sont grosso modo parallèles les unes aux autres. Des indications de l'amorce d'un ordre bi-dimensionnel dans le graphite de type d3 sont fournies par l'apparition d'une raie diffuse à 2,13 Å sur les diagrammes de poudre. À partir du graphite de type d3, la graphitisation se développe de trois façons : (1) amélioration de la structure en feuillets ; (2) réduction de la taille des espacements interfoliaires ; (3) amélioration de l'ajustement des feuillets les uns par rapport aux autres. Ainsi, lorsque l'on va du type d3 au type d1, la raie du pic 0002 se resserre, l'espacement interfoliaire diminue jusqu'à une valeur finale de 3,35 Å. Le passage du graphite de type d3 au graphite de type d2 est progressif.
En contraste avec le rôle de la température, on connaı̂t moins bien le rôle de la déformation et des contraintes. On sait que des contraintes non hydrostatiques peuvent induire des transformations polymorphiques et accélérer certains changements de phase [8]. Une étude récente [10] a montré que des déformations anisotropes pouvaient augmenter la vitesse de graphitisation. Nous avons voulu ici apporter des évidences de terrain sur cette question, qui n'a pour l'instant été abordée que de façon expérimentale par les auteurs cités. Pour cela, nous avons prélevé des échantillons dans la vallée de la Lotru (formation de Schela, Carpathes roumaines) et les avons examinés par diffractométrie des rayons X, microspectrométrie Raman et microsonde électronique. Nous nous poserons, dans le même cadre, la question de l'origine biologique ou non biologique du graphite, qui peut avoir son importance en ce qui concerne sa possible valorisation économique.
2 Données géologiques et minéralogiques sur les roches étudiées
2.1 Données géologiques
La région étudiée appartient à l'unité structurale de Schela de la nappe dite gétique des Carpathes roumaines [3,4] (Fig. 1). La formation de Schela montre un ensemble de roches anchi-métamorphiques constitué de métaconglomérats, de métagrès carbonés, de schistes pyrophyllitiques, de schistes à chloritoı̈de et de lentilles anthracitiques [8]. La formation de Schela représente un ensemble de roches métamorphiques localisées au contact entre la nappe gétique et l'autochtone danubien [2,5,12]. L'âge de la formation de Schela est liasique [2] ou carbonifère [11].
Dans cette région, le travail cartographique a permis d'individualiser un corps de schistes graphiteux, dans lequel sont présentes des intercalations de lentilles de quartzites. D'après quelques auteurs [4,7], les schistes graphiteux de cette région ont été formés par le métamorphisme de formations terrigènes, d'âge Paléozoı̈que, transformées dans les conditions allant du faciès des schistes verts au faciès à quartz, albite et chlorite. Le corps des schistes graphiteux possède des dimensions plurikilométriques et présente une forme tabulaire ; l'horizontale de la foliation a une direction N 220° et l'inclinaison des plans est de 10° à 24° vers le sud. Ce corps a une fabrique essentiellement planaire, avec une linéation peu exprimée ; elle est observée seulement au microscope, où elle se marque par la direction des agrégats de micas, chlorite et graphite. Les quartzites sont disposés en concordance avec le plan de foliation des schistes graphiteux et se présentent sous la forme de lentilles de dimensions centimétriques. Les minéraux n'y montrent pas d'orientation préférentielle. On note, toutefois, une alternance de bandes plus ou moins riches en matériel carboné. On observe un appauvrissement en matériel carboné vers l'extérieur de la lentille de quartzite. Les fragments de matériel carboné ont des dimensions plus grandes dans la partie centrale de la lentille.
2.2 Composition minéralogique
2.2.1 Schistes graphiteux
La composition minéralogique modale a été évaluée au microscope polarisant sur lames minces ; la composition chimique des minéraux a été déterminée à la microsonde électronique. Le corps des schistes graphiteux est formé de quartz (31–68 %), de micas (11–20 %), de daphnite (3–9 %), de Fe-chloritoı̈de (2–6 %), de pyrophyllite (< 2 %), de graphite (10–18 %) et de rutile (< 1 %). Dans la pyrophyllite, une petite partie de l'aluminium est substituée au fer. Les micas représentent une solution solide entre les pôles Fe-céladonite, muscovite et paragonite. Les associations de micas et graphite se disposent en alternance avec des bandes de quartz (Fig. 2). Au microscope optique, on peut observer la redistribution des minéraux le long des plans de foliation. Sur la base des données chimiques et minéralogiques (Barzoi, travail en cours), on peut estimer que la pression a pu atteindre des valeurs de 1 à 2 kbar et la température des valeurs de 240 à 260 °C.
2.2.2 Quartzites
La composition minéralogique modale est : quartz (82–90 %), matériel carboné (10–20 %), micas (< 1 %). Dans les quartzites, on rencontre des fragments de matériel carboné, avec des contours arrondis qui indiquent que le matériel carboné n'est pas totalement cristallisé. Le quartz est disposé en colonnes radiales autour des grains de matériel carboné.
3 Cristallinité du matériel graphiteux
3.1 Préparation et méthodes analytiques
L'étude a porté sur 16 échantillons de schistes graphiteux et huit échantillons de quartzites, contenant du matériel carboné. La séparation du matériel carboné des quartzites a été réalisée par broyage, puis tri sous microscope. La séparation du graphite des schistes graphiteux a été réalisée par la méthode décrite par Tagiri [13] : broyage des échantillons à 120 μm ; mélange de 10 g de roche broyée avec 50 mL de HCl concentré ; chauffage dans un bain de vapeur pendant 12 h, avec montée progressive de la température pendant 3 h jusqu'à atteindre une valeur de 90 °C. Les échantillons ont été centrifugés et lavés avec de l'eau distillée. Pour séparer le graphite des autres minéraux silicatés, les échantillons ont été mélangés avec 50 mL de HF 50 %, puis chauffés dans un bain de vapeur pendant 12 h à une température de 80 °C. Les échantillons ont été ensuite centrifugés et lavés avec de l'HCl 37 % et de l'eau distillée.
Le degré de cristallisation du graphite a été déterminé à l'aide d'un diffractomètre Siemens Kristalloflex D5000 (constante de temps : 2,0 s ; pas de variation : 0,020° ; longueur d'onde : 1,540 6 Å ; température : 20 °C) et à l'aide d'un spectromètre Raman. Les spectres Raman ont été obtenus en utilisant un laser à 514 nm d'ion Ar+ à 0,1–0,2 W. Le laser a été focalisé sur l'échantillon à l'aide d'un microscope pétrographique Olympus (BH2-UMA), modifié de façon à obtenir un spot de taille inférieure à 3 μm de diamètre. La raie laser a été éliminée grâce à un filtre et le signal Raman dispersé par un monochromateur Jobin Yvon HR640 avec 600 traits/mm sur un détecteur CCD ( pixels) refroidi à l'azote liquide. Les échantillons ont été disposés sur lames minces et le spectre a été établi à température ambiante pendant 200 à 400 s.
3.2 Résultats
Les diffractogrammes et le spectre Raman indiquent un degré de cristallisation différent entre le matériel carboné rencontré dans les lentilles de quartzites et celui rencontré dans les schistes graphiteux (Tableau 1, Figs. 3 et 4). D'après la classification de Landis [1], le graphite des schistes graphiteux de la vallée de la Lotru est un graphite-d1, et le matériel carboné des lentilles de quartz est un graphite-d2. Le spectre de diffraction commençant à 5° 2-théta, on est sûr de l'absence de mica résiduel (absence de raie autour de 8,8° ; la forte raie 006 du mica aurait pu interférer avec la raie 0002 du graphite). Par ailleurs, les différences de cristallinité ne paraissent pas imputables à la taille des domaines cohérents : leur taille, estimée par l'observation des sections de roches en lumière réfléchie, est en général supérieure à quelques dizaines de microns et ne joue donc pas sensiblement sur l'élargissement des raies et l'espacement basal apparent des cristallites. Les spectres Raman du premier ordre montrent bien le degré de cristallinité en termes de dimensions des domaines cristallins dans le plan (0001) [6]. Les deux bandes concernées sont celles à 1 400 et .
4 Discussion : rôle de la déformation dans le processus de graphitisation
Les deux méthodes utilisées (diffraction des rayons X et spectrométrie Raman) montrent que le matériel carboné est bien cristallisé dans les schistes graphiteux, alors qu'il est amorphe dans les quartzites. Sur la Fig. 4, on peut observer le caractère cristallin du graphite (a), des schistes graphiteux et le caractère amorphe (b) du matériel carboné des quartzites. La cristallinité augmente avec l'étroitesse des largeurs à mi-hauteur des bandes d'absorption, les bandes Raman qualifiant une cristallinité très locale (ordre à courte distance des vibrateurs intracouches). La différence essentielle de degré de cristallisation entre le matériel carboné des schistes graphiteux et celui des quartzites est imputable à la déformation. Ainsi, dans des conditions thermiques identiques, l'apparition de surfaces de discontinuité plan-parallèles a permis de développer les structures cristallines planes du graphite dans les schistes graphiteux. D'après Ross et Bustin [10], une substance carbonée ne peut pas se transformer en graphite à des températures inférieures à 900 °C, s'il n'existe pas de surfaces de discontinuité plan-parallèles où puissent se développer les structures cristallines du graphite. Dans les quartzites, la déformation est pratiquement nulle et, pour cette raison, le matériel carboné n'a pas évolué jusqu'à un graphite ordonné.
Pendant le métamorphisme, sur les plans où existe une contrainte plus forte, le matériel carboné évolue jusqu'au stade du graphite-d1. Dans les lentilles de quartz, la contrainte au sein des agrégats de matière organique est réduite. La température dans les schistes pourrait être légèrement supérieure à celle dans les quartzites, du fait des frottements accompagnant les ruptures et déplacements des plans de foliation, et contribuer ainsi à l'évolution plus importante du matériel carboné dans ces roches. Dans les quartzites, le matériel a pu évoluer plus lentement et est resté au stade du graphite-d2. La diminution du volume occupé par le matériel carboné est une preuve de son origine biologique et de son évolution en un matériel plus ordonné. La perte de volume correspond probablement à celle engendrée par la conversion de la matière organique en graphite d2, soit 10 % (en supposant raisonnablement que la matière carbonée est insoluble dans les fluides intergranulaires). Un problème intéressant que l'on peut observer (Fig. 5) est celui de la disposition du quartz autour des grains du matériel carboné ; le quartz est néoformé et le volume occupé par le matériel carboné s'est réduit. On peut supposer la simultanéité du développement du quartz et de la réduction du volume du matériel carboné.
Remerciements
Les auteurs remercient MM. A. Baronnet, P. Bussek, J. Moutte, M. Seclaman, G. Thomas et O. Valfort pour leur concours.