1 Introduction
The absence of marine magnetic anomalies older than 250 Ma forces to study type sections to build the pre-Jurassic GPTS (Geomagnetic Polarity Time Scale) [15]. However, in general, the type sections have problems, like unconformity, poor age control, lack of diagnostic fossils, and inadequate descriptions, which impede establishing a reliable magnetostratigraphy.
During the last decade, new data has become available on the length and duration of the Permo-Carboniferous reversed superchron (PCRS or Kiaman after [14]). The review of this dataset [11,22,23] concluded that the boundaries for this period (PCRS) are from 260 to 315 Ma [6]. Opdyke [18] also pointed out that the frequency of reversal before and after the onset of the PCRS is similar to that observed before and after the Cretaceous quiet zone (KQZ). Thus, the Earth's magnetic field seems to have two states, one with reversal frequencies exceeding one per million years and the other with no or very few reversals for tens of millions of years. Finally, regarding to the possibility of correlation between tectonic and volcanic effects with the behavior of the Earth's magnetic field, it was speculated that the KQZ occurred at a time of continental separation and the PCRS at a time of continental amalgamation. Despite an obvious interest to study with great detail the Permo-Carboniferous time, there is only few reliable paleomagnetic data available and the magnetostratigraphy is still uncertain.
Here, we report new data from a sedimentary sequence of a well-defined stratigraphy and high-resolution biostratigraphy [29]. This sequence is approximately 700 m thick, and represents one of the best Permo-Carboniferous outcrops in North America. We carried out a paleomagnetic survey to determine their magnetic polarities and to try to obtain some decisive constraints for Permo-Carboniferous magnetostratigraphy. A previous, very detailed paleontological study [29] was particularly encouraging.
2 Geology and sampling
The Patlanoaya Formation consists of sedimentary rocks with abundant macrofossils on the Tepazulco hills, to the northern part of Patlanoaya, Puebla [30]. It overlies unconformably the Acatlan Complex (Fig. 1) of Lower Paleozoic age [24]. The Patlanoaya Formation seems to be the oldest sedimentary sequence that covers the Mixteco Terrain [2] and it is considered as a valuable geological piece for understanding the Paleozoic tectonic history of Mexico [29].
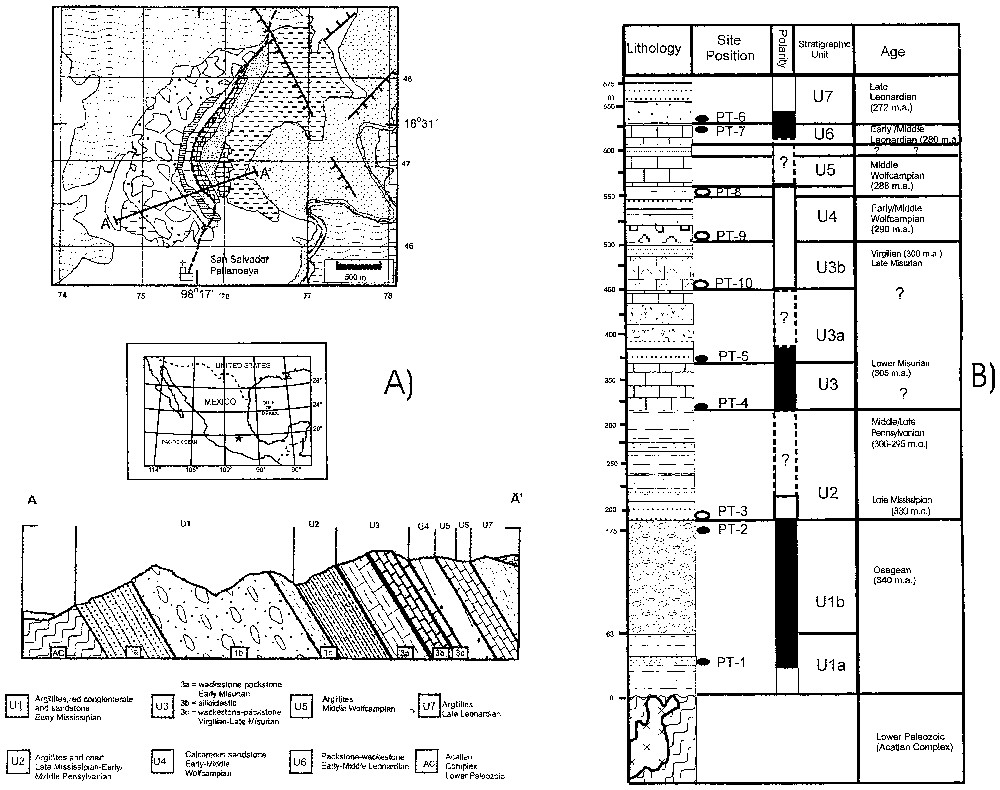
Location and geologic setting of the San Salvador–Patlanoaya region (upper left). The geologic structure and lithological description of sampled profile is shown at the lower part (A). At the right part is showed the lithology, (magneto)stratigraphy and ages of limestones (B). Stratigraphic positions of the studied sites are also shown.
Localisation et cadre géologique de la région de San Salvador–Patlanoaya (en haut à gauche). La structure géologique et la description lithologique du profil échantillonné sont donnés dans le bas de la figure (A). Sur la partie de droite sont indiqués la lithologie, la (magnéto)stratigraphie et les âges des calcaires (B). Les positions stratigraphiques des sites étudiés sont également fournies.
The Patlanoaya Formation was first dated as Mississippian–Permian in age (brachiopods and cephalopods, [30]), later confirmed by additional biostratigraphic work [1,9,31]. Recent geological and biostratigraphic study indicates an Early Osagean to Late Leonardian age for the Patlanoaya Formation (∼340–275 Ma) [29], equivalent to a Mississippian-Early Permian age. The time scale proposed by Ross et al. [25] was used to assign numerical ages to the units.
Based on lithology and age, the Patlanoaya Formation is subdivided into seven units (Fig. 1), whose lithological and paleontological description is taken mainly from [29] and [31].
In total, 63 oriented cores belonging to 10 sites were sampled. Commonly, the outcrops extend laterally over a few tens of meters. In these cases we drilled typically six to eight cores per site. The samples were distributed throughout each unit, both horizontally and vertically, in order to minimize the effects of block tilting and lightning. At a few small outcrops, only four samples were collected. Cores were obtained with a gasoline-powered portable drill, and then oriented with magnetic compass.
3 Magnetic measurements
The remanent aimantations of four to eight samples from each unit were measured with a JR-5A spinner magnetometer (sensitivity ∼10−9 A m2). Both alternating field (AF) desaimantation using a laboratory made AF-demagnetizer and stepwise thermal desaimantation up to 500–520 °C using a non-inductive Schonstedt furnace were carried out on pilot samples. All 63 samples were subjected to thermal desaimantation (see upper part of Fig. 2). In general, 12–14 temperature steps were distributed between 20 °C and 520 °C. During thermal desaimantation, the low-field susceptibility at room temperature was measured after each step with a Bartington susceptibility meter.

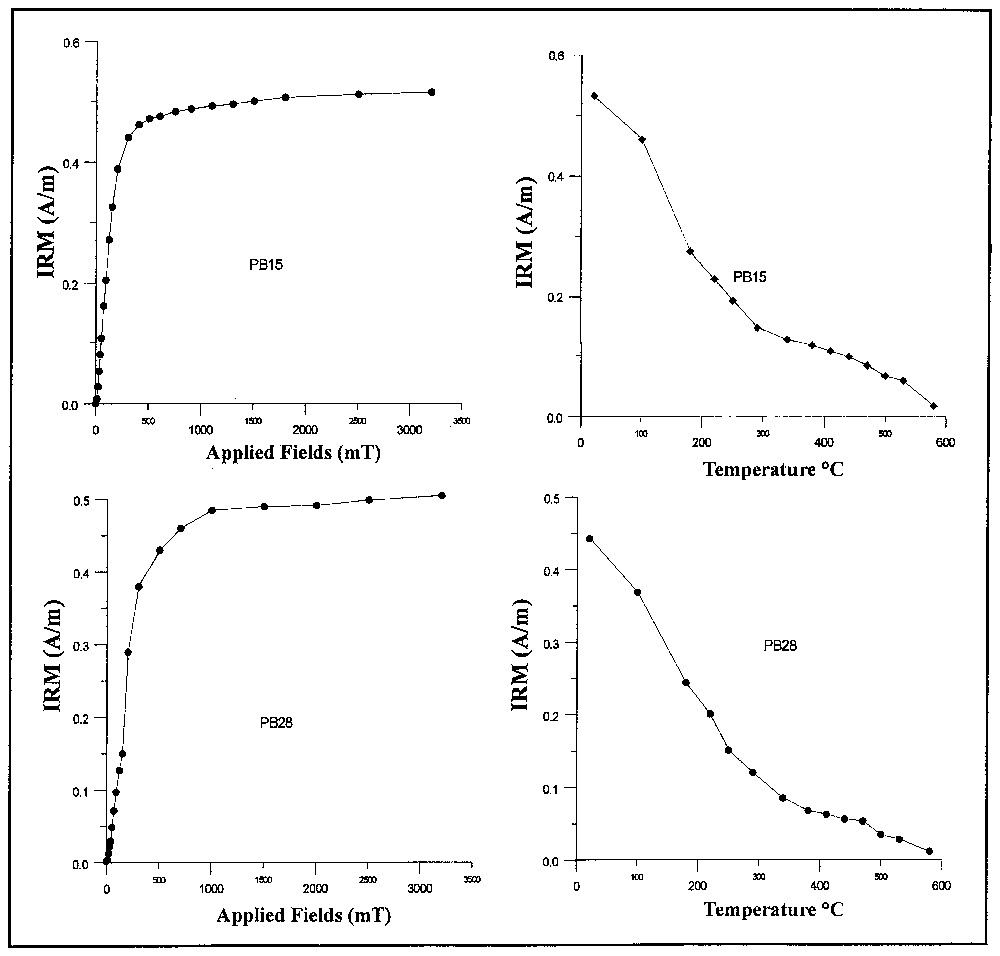
The upper part shows representative orthogonal vector plots of stepwise thermal desaimantation (stratigraphic coordinates). The numbers refer to the temperatures (in °C). ∘: Projections into the horizontal plane; ×: projections into the vertical plane. At the bottom are displayed acquisition and thermal desaimantation curves of the isothermal remanent aimantation for representative samples.
Dans la partie supérieure sont figurés les courbes représentatives des vecteurs orthogonaux de la désaimantation thermique par étapes. Les nombres correspondent aux températures (en °C). ∘ : Projections dans le plan horizontal ; × : projections dans le plan vertical. Dans la partie inférieure sont présentées l'acquisition et la désaimantation thermique de l'aimantation rémanente isothermale, pour des échantillons représentatifs.
In most units only one paleomagnetic component could be recognized (Fig. 2). Small secondary components were easily removed applying 100–180 °C. Results from units PT1, PT9 and PT10 yielded unstable thermal behavior and evidence for multicomponent aimantation. These samples are characterized by relatively big capacity for VRM (viscous remanent aimantation) acquisition. Primary aimantation components have been defined after 250 °C for these samples. The greater part of remanent magnetization was removed at temperatures between 450 and 510 °C (Fig. 2), which may indicate that relatively low-Ti titanomagnetites are responsible for magnetization. Nevertheless, a smaller fraction of higher unblocking temperatures could sometimes be observed (Fig. 2).
Directions of characteristic aimantation components were calculated by principal component analyses [17], using at least four points. A structural correction was applied to the sedimentary units, measured as 50–60° (according to outcrop) in the direction NW ∼35 °SE. The average unit directions are rather precisely determined (Table 1). Almost all α95 are less than 13, except units PT1 and PT5. These values are higher compared to studies from volcanic units, but still reliable for the magnetic polarity determination, which is the main objective of this study. Six units are normally magnetized and four units yielded reverse polarity aimantation. We note however that it cannot be ascertained that unit PT9, which is presented only with one sample, is reversely magnetized.
Average, tilt corrected paleodirection of cleaned remanence and corresponding VGP positions for all studied units. , number of treated samples; , number of specimens used for calculation; Dec, declination; Inc, inclination; and , precision parameter and radius of the 95% confidence cone of the Fisher statistics; Pol, magnetic polarity; Plat/Plong: latitude/longitude of the VGP position.
Paléodirection moyenne, corrigée du pendage de la rémanence nettoyée et positions VGP correspondantes pour toutes les unités étudiées. , nombre d'échantillons traités ; , nombre de spécimens utilisés pour le calcul ; Dec, déclinaison ; Inc, inclinaison ; et , paramètre de précision et rayon du cône de confiance à 95% des statistiques de Fisher ; Pol, polarité magnétique, Plat/Plong : latitude/longitude de la position VGP.
Site | n/N | Dec | Inc | A95 | Plat | Plong | Pol | |
PT6 | 5/6 | 336 | 36 | 31.8 | 13.8 | 67 | 179.4 | |
PT7 | 5/6 | 34.9 | 33 | 39 | 12.4 | 56.9 | 347.1 | |
PT8 | 5/6 | 215 | −18 | 47.7 | 12.6 | −55.4 | 182 | |
PT9 | 1/6 | 170 | −13 | −75 | 302.8 | R? | ||
PT10 | 6/7 | 153 | −36 | 62.3 | 7.8 | −64.1 | 359.2 | |
PT5 | 5/6 | 41.3 | 46 | 22.7 | 16.4 | 51.1 | 330.2 | |
PT4 | 8/8 | 24.5 | 35 | 30.2 | 6.7 | 66.8 | 346.1 | |
PT3 | 4/4 | 226 | −35 | 70.5 | 11 | −46.3 | 162.5 | |
PT2 | 6/6 | 355 | 29 | 51.9 | 9.4 | 84.1 | 142.3 | |
PT1 | 3/7 | 321 | 37 | 44.6 | 18.7 | 53.1 | 181.8 |
To determine the isothermal remanent aimantation (IRM) curves, previously AF demagnetized samples from all units were subjected to magnetic fields along vertical axes. The magnetic fields were steadily increased up to 3.2 T, their remanence being measured after each step. IRM acquisition curves were found very similar for all samples (see bottom part of Fig. 2). Saturation is reached in moderate fields of the order of 150–200 mT, which points to some spinels as remanence carriers. Thermal desaimantation of IRM indicates that important part of aimantation is already unblocked at 300 °C. Isothermal remanence is completely removed around 580 °C. This behavior may be owned by presence of both high- and low-Ti titanomagnetites with similar coercivities [16]. Alternatively, one can speculate that the rapid decay of IRM around 300 °C may correspond to greigite, which is also a ferrimagnetic mineral [8] and can provide a stable remanence [3].
4 Discussion and conclusions
We consider the characteristic paleomagnetic directions determined in this study to be of primary origin. This is confirmed by the fact that both normal and reversed polarities directions were determined. The reversal test (i.e., check the antipodality of reverse and normal polarity directions) cannot be applied correctly in our case because six units were found normally magnetized, against only three reverse polarity units. The reversal test is commonly used with more numerous datasets with equal numbers of reverse and normal polarity units. However, characteristic aimantation components were successfully isolated in most cases. The mean tilt corrected paleomagnetic direction obtained in this study is Inc=35.5°, Dec=7.5°, k=7.5, α95=17.8°, N=10. Mean directions without the correction for tilting is Inc=29.2°, Dec=203.7°, k=1.6, α95=59.3°, which additionally attests the primary character of remanent aimantation. Our dataset is not sufficiently robust to enable constraints about the tectonic evolution of the studied area. However, it may be used for high-resolution magnetostratigraphic studies, which are the main objective of present study.
Although numerous paleomagnetic studies were carried out from Permo-Carboniferous time, their magnetostratigraphy is still controversial. Upper Permian provides at least eight normal magnetozones between 262 and 250 Myr, after studies from several areas of the world [18]. A detailed study from Upper Permian was done by [20] and [11]. These authors found several reversals up to 262–265 Myr, followed by a relatively big interval of reverse aimantation, known as PCRS (initially known as Kiaman superchron).
The best determination of the age for the duration of the PCRS comes from Canadian Maritimes [6,7], which gives approximately a 50-Myr interval between 262 and 312 Myr. This period of time is dominated by reverse polarity aimantation. However, some authors report occurrence of several short normal subchrons within the PCRS [4,5,10,12,13,16,21,27,32]. Some of them coincide with the base of Permian (298–300 Myr), which shows normal polarity. Our paleomagnetic record reveals two consecutive normally magnetized units at about 305 Myr, which may be speculatively correlated with normal magnetozone found by [20] (Fig. 3). Another normal subchron (defined also by two consecutive units) was detected at about 280 Myr. This seems correspond to the normally magnetized sediments, found 100 m below of a lava dated at 288±5 Myr [26].

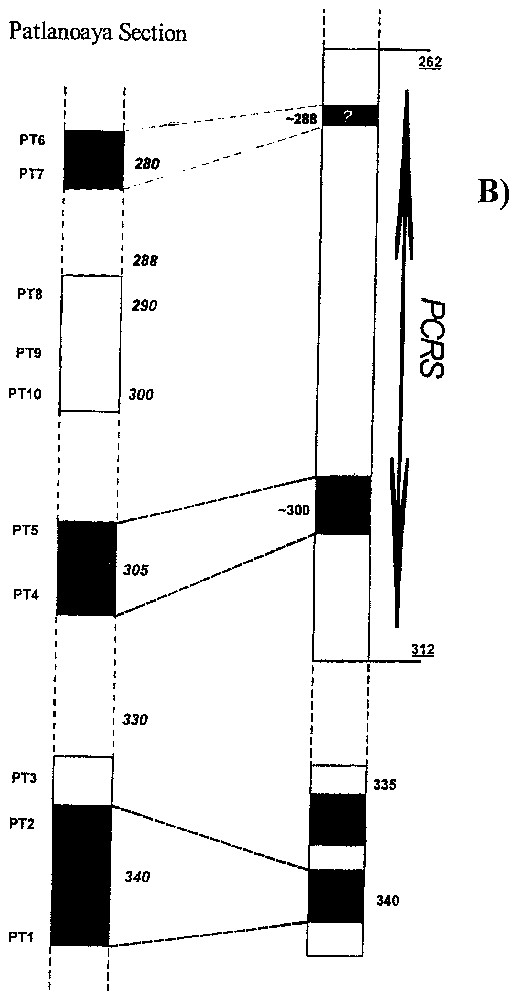
A. Equal area projections of the site-mean characteristic paleodirections for the studied sequence. B. A tentative magnetostratigraphic correlation between Patlanoaya section and geomagnetic polarity time scale (retrieved from [22]).
A. Projections en surface des paléodirections caractéristiques de moyenne de site pour la séquence étudiée. B. Tentative de corrélation magnétostratigraphique entre la coupe de Patlanoaya et l'échelle de temps de la polarité géomagnétique (d'après [22]).
There is still great uncertainty for the reversal pattern for the Carboniferous time. McCabe and Channell [19] found that previous studies for this period are remagnetized, and therefore seriously doubtful. The rocks at the base of Patlanoya section seem to be free of strong remagnetization and yield both normal (two sites) and reverse polarity chrons at about 340 Myr, in agreement with [28] study.
In conclusion, rock-magnetic experiments yielded that mean magnetic carriers are some spinels, most probably, titanomagnetites, although greigite may also exist judging from thermal demagnetization of isothermal remanence. Remanence analyses yield that in most of studied units, only one paleomagnetic component could be recognized with minor secondary components, which were easily removed applying 100–180 °C. The data quality appears to be high and the results internally consistent. Six normal and four reverse magnetozones were recognized from bottom to top in Patlanoya section between 340 and 280 Myr. These results may help to a better knowledge of Permo-Carboniferous magnetostratigraphy. Both normal and reverse polarity rocks were found in Carboniferous time around 340 Myr, which supports previous paleomagnetic works. Our record revealed that two normal subchrons within PCRS at 305 and 280 Myr, respectively, which may be speculatively correlated to normal aimantations, occasionally occurred during the Kiaman interval. More detailed studies are needed in order to establish a fine magnetostratigraphy for Permo-Carboniferous time.
Acknowledgements
This work was supported by a sabbatical grant from Instituto Mexicano Petroleo, financial support from CONACyT Bilateral Project Mexico–Cuba, and CONACyT research project J32727-T. Magnetic measurements were carried out by A. Gonzalez.