1 Introduction
Geomagnetic paleointensity data are critical for understanding the workings of the Earth's dynamo. Coe et al. [7] recently suggested that absolute intensity should be a fundamental constraint in numerical models that promise to provide unprecedented insight into the operation of the geodynamo. However, absolute paleointensity data are still scarce and cannot be yet used to document the general characteristics of the Earth's magnetic field [36]. Reliable paleointensity values are generally much more difficult to obtain than reliable directional data, because only volcanic rocks which satisfy some very specific magnetic criteria [4,20] can be used for absolute paleointensity determination.
Judging from the existing paleointensity database (ftp://ftp.dstu.univ-montp2.fr/pub/paleointb, see also [26]) only large-scale features can be resolved, such as the pronounced lowering of the Earth's Virtual Dipole Moment (VDM) in the whole Mesozoic and part of Paleozoic [29], during which the dipole structure of the field was preserved [25]. However, high geomagnetic intensities have been recently reported [14,38] for the Middle Cretaceous and thus the reliability of Mesozoic Dipole Low (MDL) may be questioned. To try to obtain more constraints for the Mesozoic geomagnetic field strength, we carried out Thellier paleointensity experiments on ∼187 Ma old La Negra volcanic succession in northern Chile. Obtaining of new paleointensity and paleodirection determination from the southern America volcanics is critical, because the geographic distribution of paleointensity data is still very uneven (e.g., [37]). In particular, very few data are available from the southern hemisphere, which impedes an accurate analysis of the fine-scale changes in the statistical characteristics of paleosecular variations [16].
2 Sampling details
The La Negra Formation (Figs. 1 A and B) comprises a thick pile of lava flows, largely of high-K basaltic andesites, with thin intercalated sediments. It seems that they erupted in an extensional environment [34] and that, together with their chemical similarity to the Puente Piedra volcanics in Peru [3] and the lavas at Bustamante Hill in Central Chile [22], suggests they were erupted in an ensialic back-arc basin rather than in an actual volcanic arc.


(A) Sketch map of northern Chile illustrating the locality of paleomagnetic sampling (modified from [34]). (1) La Negra Formation, (2) and (3) are Gatico (∼158 Ma) and Tocopilla (∼155 Ma) intrusions, respectively. (B) Simplified stratigraphy of Antofagasta region, northern Chile, with the principal names of geological formations (redrawn from [2]).
(A) Carte schématique du Nord-Chili, montrant l'emplacement des lieux d'échantillonnage paléomagnétique (modifié d'après [34]). (1) Formation de La Negra ; (2) et (3), intrusions de Gatico (∼158 Ma) et Tocopilla (∼155 Ma). (B) Stratigraphie simplifiée de la région d'Antofagasta, Nord-Chili, avec les principales dénominations des formations géologiques (redessiné à partir de [2]).
Paleomagnetic sampling was done near of town of Tocopilla (Fig. 1). Rogers et al. [34] proposed a mean age of 187 Ma as the best estimate of the time of emplacement of the lower La Negra volcanic sequence. It is only regrettable that these authors do not report the error on their K–Ar age. We note, however, that the sample dated by Rogers and Hawksworth [34] most probably belongs to site NE06 of our collection (lower part of La Negra sequence [1]). In total, about 280 oriented samples belonging to 32 consecutive lava flows were collected. Commonly, the outcrops extend laterally over a few tens of meters and, in these cases, we drilled typically 6–11 standard paleomagnetic cores per flow. The samples were distributed throughout each flow both horizontally and vertically in order to minimize effects of block tilting and lightning. Cores were obtained with a gasoline-powered portable drill, and then oriented with both magnetic and sun compasses.
3 Rock-magnetic characteristics of selected samples
Magnetic characteristics of typical samples selected for Thellier paleointensity measurements are summarized in Fig. 2 (upper part) and could be described as follows.
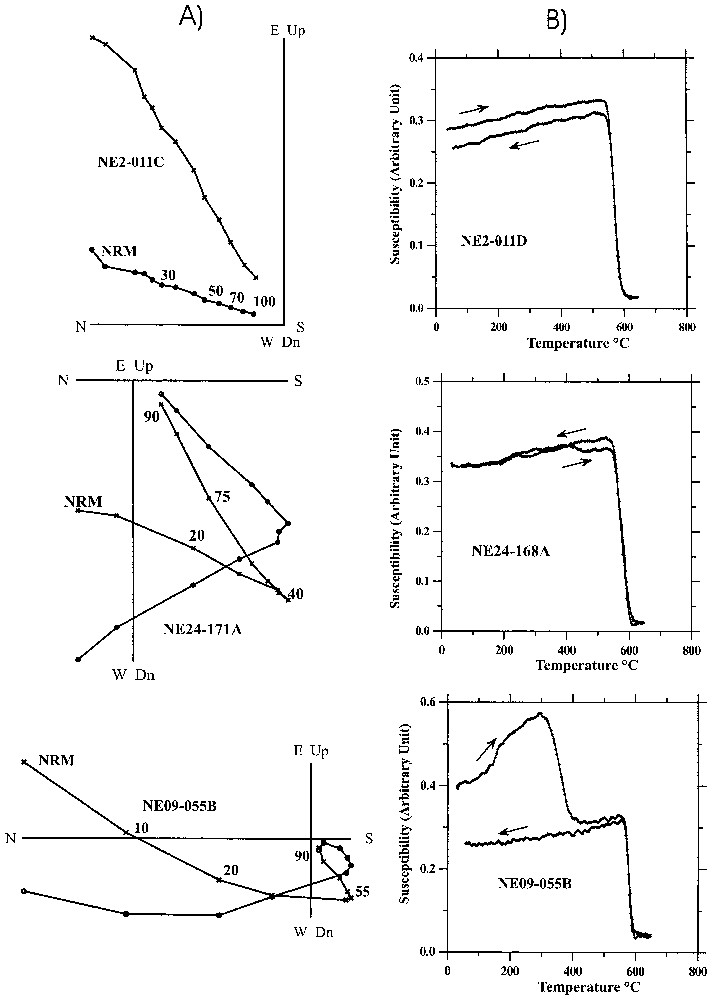
Summary of the magnetic characteristics of the typical samples, selected (class 1) and unselected (classes 2 and 3; see also text) for Thellier paleointensity experiments. (A) Orthogonal vector plots of stepwise alternating field demagnetization (stratigraphic coordinates). The numbers refer to peak alternating fields in mT. –•–: projections into the horizontal plane, –
Résumé des caractéristiques magnétiques des échantillons typiques, sélectionnés (classe 1) et non sélectionnés (classes 2 et 3; voir aussi le texte) pour les expériences de paléointensité de Thellier. (A) Représentation par rapport à des vecteurs orthogonaux de la désaimantation par palier du champ alternatif (coordonnées stratigraphiques). Les nombres se rapportent au pic des champs alternés, en mT. –•–: Projections dans le plan horizontal, –
Résumé des caractéristiques magnétiques des échantillons typiques, sélectionnés (classe 1) et non sélectionnés (classes 2 et 3; voir aussi le texte) pour les expériences de paléointensité de Thellier. (A) Représentation par rapport à des vecteurs orthogonaux de la désaimantation par ... Lire la suite
1. Selected samples carry essentially a single and stable component of magnetization, observed upon alternating field (Fig. 2A, sample NE2–011C) treatment. A generally minor secondary component, probably of viscous origin, was present but was easily removed. The median destructive fields (MDF) range mostly in the 40–60-mT interval, suggesting the existence of small pseudo-single to single magnetic domain grains as remanence carriers, which are suitable material for the Thellier paleointensity study [8].
2. Low-field continuous susceptibility measurements with temperature show the presence of a single ferrimagnetic phase with Curie point compatible with Ti-poor titanomagnetite. However, the cooling and heating curves are sometimes not perfectly reversible (Fig. 2B, sample NE2–011D) probably due to the heating in air. In addition, hysteresis measurements were carried out on all samples (not shown). However, we believe that the magnetic domain state estimation using room temperature hysteresis parameters in terms of the plot of magnetization ratio vs. coercivity ratio has no resolution for most of natural rocks [12]. Thus, these data were not used to select the most promising samples for Thellier paleointensity experiments.
In all, we selected 26 samples for the paleointensity experiments having the above-described magnetic characteristics. Remaining samples may be divided in two groups (Fig. 2, middle and lower parts) after their magnetic properties: (i) the samples yield reasonably reversible continuous susceptibility curves (Fig. 2B, sample NE24–168A) associated with the remanence dominated by strong secondary magnetization (Fig. 2A, sample NE24–171A); (ii) the k−T curves of these samples yield the evidence of two ferrimagnetic phases during heating (Fig. 2B, sample NE9–055). The lower Curie point ranges between 350–400 °C, and the highest one is about 580 °C. The cooling curve shows only a single phase, with a Curie temperature close to that of magnetite. Such irreversible k−T curves can be explained by titanomaghemite, which probably transformed into magnetite [24,30] during heating. It is possible that these samples carry chemical remanent magnetization. In addition, this behavior is related by very unstable multivectorial magnetization (new version of Fig. 2A, sample NE09–055B). It is obvious that these samples cannot be used to determine absolute geomagnetic paleointensity.
4 Paleodirections
As part of their efforts to study the details of the volcanic stratigraphy and paleotectonics on Antofagasta region (northern Chile), Alva-Valdivia et al. [1] recently carried out a detailed paleomagnetic study of La Negra Formation. The reliable paleodirections were obtained for 25 sites among 32 sampled (Fig. 3). These directions are considered to be of primary origin because of the occurrence of almost antipodal normal and reversed polarities (Fig. 3). In addition, unblocking temperature spectra and relatively high coercivities point to ‘small’ pseudo-single domain magnetic structure grains as responsible for remanent magnetization. The secondary magnetizations were successfully removed in most cases using the alternating-field demagnetization technique.
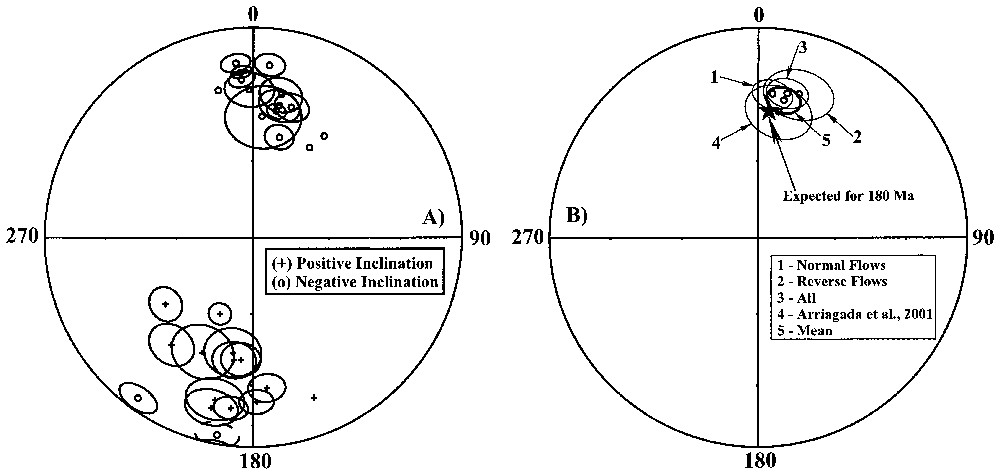
(A) Equal area projections of the flow mean characteristic paleodirections for La Negra Formation. Circles/Crosses denote to the negative/positive inclination. (B) Equal area projection of the mean directions for Antofagasta–Tocopilla volcanic units [1].
(A) Projections en surface des paléodirections caractéristiques moyennes de coulée dans le cas de la Formation de La Negra. Les cercles/croix indiquent l'inclinaison négative/positive. (B) Projections en surface des directions moyennes pour les unités volcaniques d'Antofagasta –Tocopilla [1].
The mean paleodirection obtained in this study is
5 Paleointensity determination
Paleointensity experiments were performed using the Thellier method in its classic form [41]. Heating was made in a vacuum better than 10−2 mbar. All remanences were measured using both JR5A and JR6 spinner magnetometers. Paleointensity data are reported on the Arai-Nagata [23] plot in Fig. 4 and results are given in Table 1. We accepted only determinations: (1) obtained from at least 5 NRM-TRM points corresponding to a NRM fraction larger than about 1/3 (Table 1), (2) yielding quality factor [6] generally above 5, (3) with positive ‘pTRM’ checks i.e. the deviation of ‘pTRM’ checks were less than 15% and (4) with reasonably linear Zijderveld diagrams obtained from the paleointensity experiments. No significant deviation of NRM remaining directions towards the direction of applied laboratory field was observed. To better illustrate this point, we calculated the ratio of potential CRM(T) to the magnitude of NRM(T) for each double heating step in the direction of the laboratory field during heating at T [9]. The values of angle γ – the angle between the direction on characteristic remanent magnetization (ChRM) obtained during the demagnetization in zero field and that of composite magnetization (equal to NRM(T) if CRM(T) is zero) obtained from the orthogonal plots derived from the Thellier paleointensity experiments – are reported in Table 1. For accepted determinations γ values are all <10.6°, which attests that no significant CRM was acquitted during laboratory heating [9]. This approach is probably more restrictive than simple calculation of angle between the characteristic directions determined by Thellier experiments and by thermal demagnetization in zero field.
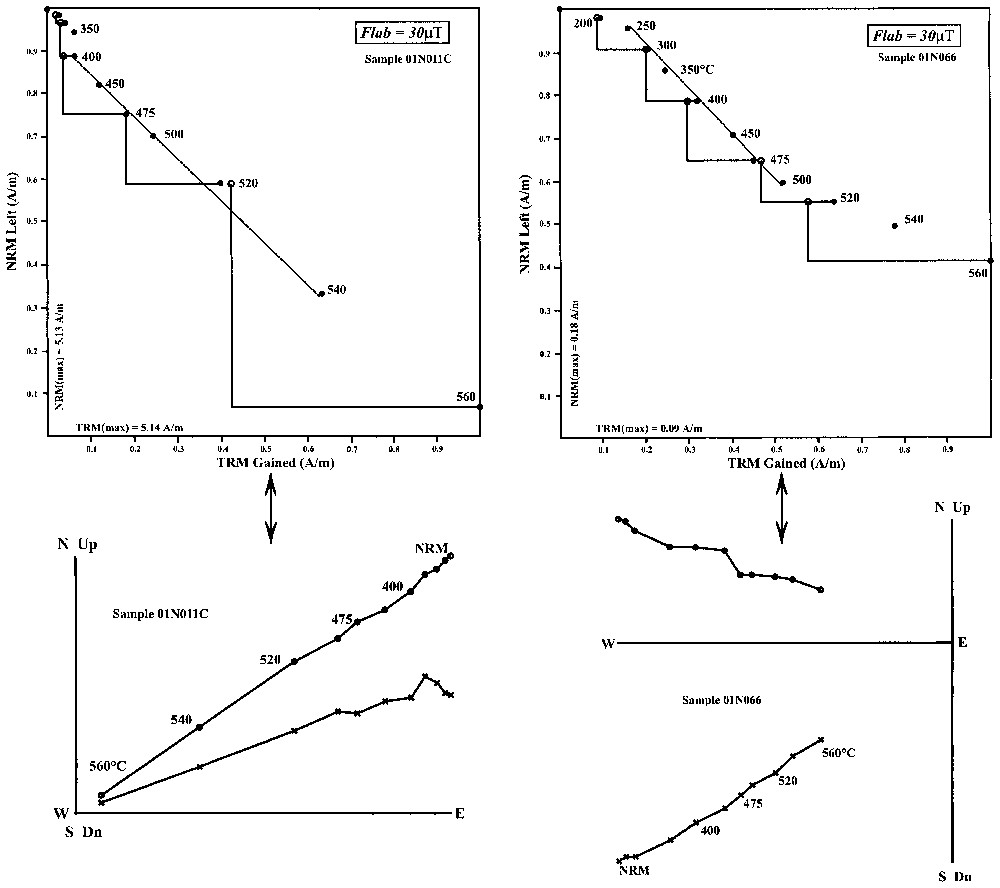
The representative NRM–TRM plots and associated orthogonal diagrams for La Negra samples. In the orthogonal diagrams we used same notations as in Fig. 2A.
Courbes représentatives NRM–TRM et diagrammes orthogonaux associés pour les échantillons de La Negra. Dans les diagrammes orthogonaux sont utilisées les mêmes notations que sur la Fig. 2A.
Paleodirectional and paleointensity results from La Negra volcanic lava flows: N, number of treated samples; n, number of specimens used for calculation, Dec: declination, Inc: Inclination, k and α95: precision parameter and radius of confidence cone, Inc′ is the individual inclination derived from Thellier paleointensity experiments, N′ is number of NRM–TRM points used for paleointensity determination, Tmin−Tmax is the temperature interval used,
Valeurs de paléodirections et paléointensité obtenues sur les coulées volcaniques de La Negra. N, nombre d'échantillons traités; n, nombre d'échantillons utilisés pour le calcul ; Dec: déclinaison ; Inc: inclinaison ; k et α95: paramètre de précision et rayon du cône de confiance ;
Paleodirections | Paleointensity | |||||||||||||||
Site | n/N | Dec (°) | Inc (°) |
|
k | Specimen |
|
Tmin−Tmax | N′ | f | g | q | γ | FE±σ(FE) | VDM | VDMe |
NE02 | 6/6 | 14.8 | −49.2 | 5.5 | 148 | 01N007C | −48.4 | 350−520 | 6 | 0.54 | 0.78 | 5.9 | 3.3 | 18.2±1.7 | 3.59 |
|
01N008B | −49.6 | 400−520 | 5 | 0.55 | 0.78 | 8.4 | 4.4 | 21.5±1.3 | 4.18 | |||||||
01N011C | −56.2 | 400−540 | 6 | 0.58 | 0.81 | 9.8 | 1.5 | 26.1±2.6 | 4.69 | |||||||
01N012C | −54.3 | 450−560 | 6 | 0.75 | 0.79 | 6.5 | 2.9 | 24.0±2.1 | 4.41 | |||||||
NE11 | 6/6 | 203.4 | 57.3 | 4.3 | 159 | 01N062C | 51.4 | 250−500 | 7 | 0.42 | 0.82 | 4.5 | 10.6 | 35.1±3.1 | 6.68 |
|
01N063B | 54.3 | 250−500 | 7 | 0.45 | 0.85 | 6.6 | 6.3 | 41.7±3.0 | 7.67 | |||||||
01N066C | 60.5 | 250−520 | 8 | 0.51 | 0.87 | 10.7 | 2.1 | 40.4±1.5 | 6.87 | |||||||
NE19 | 7/7 | 11.3 | −36.2 | 6.9 | 76 | 01N142B | −38.6 | 300−520 | 7 | 0.45 | 0.86 | 9.4 | 0.8 | 13.8±0.7 | 3.0 |
|
01N143C | −39.3 | 300−500 | 6 | 0.30 | 0.83 | 4.4 | 8.8 | 21.6±1.7 | 4.67 | |||||||
01N145B | −44.2 | 300−500 | 6 | 0.35 | 0.87 | 6.3 | 5.3 | 16.3±1.1 | 3.36 | |||||||
NE20 | 6/6 | 5.4 | −18.8 | 5.4 | 128 | 01N146B | −19.6 | 250−500 | 7 | 0.31 | 0.82 | 5.5 | 7.7 | 14.5±0.7 | 3.59 |
6 Main results and discussion
Only 11 samples, coming from four individual lava flows, yielded acceptable paleointensity estimates. The almost 95% failure rate that we find in our study is not exceptional for a Thellier paleointensity study of old volcanic rocks, if correct pre-selection of suitable samples and strict analysis of the obtained data are made. The Thellier and Thellier [41] method of geomagnetic absolute intensity determination, which is considered the most reliable one, imposes many restrictions on the choice of samples that can be used for a successful determination [4,5,19,21,27,28].
Although our results are not numerous, some credit should be given because of good technical quality determination, attested by the reasonably high Coe et al.'s quality factors [6]. For the accepted determinations, the NRM fraction f used for determination ranges between 0.30 to 0.75 and the quality factor q varies from 4.4 to 10.7 (generally more than 5). The mean virtual dipole moments (VDMs) are ranging from 3.7±0.9 to
La Negra mean VDM is shown in Fig. 5 together with 16 other selected mean VDMs and VADMs (virtual axial dipole moment) for the period 5–200 Ma. We selected data using quite modest criteria [33] demanding (a) the mean based on more than nine successful determinations from at least three cooling units, (b) no transitional data and (c) paleointensity estimates obtained with Thellier method with pTRM checks. The perhaps most striking observation from inspecting Fig. 5 is that, in spite of the many published paleointensity studies, there exists only 17 paleomagnetic dipole moment estimates in the 5–200-Ma period that fulfill the above mentioned modest criteria. Among them, six are from submarine basaltic glass, one from single plagioclase crystal and remaining VDMs come from whole-rock samples. The excellent technical quality of paleointensity data obtained from basaltic glasses, first underlined by Tauxe and Pick [39,40] and later by Juarez et al. [17,18] is not by itself a proof of the geomagnetic validity of the paleostrength found. Goguitchaichvili et al. [10] showed that the magnetite found in this kind of material may be not of magmatic origin, but crystallizes at rather low temperatures as a result of either glass demixtion or alteration. In such a case, the Thellier paleointensity results would underestimate the actual paleofield strength. More data are needed before the intriguing differences between different materials can be put into a geomagnetic context.

Evolution of mean virtual dipole moments (VDMs) and virtual axial dipole moments (VADMs) for 5 to 200 Ma. Open squares are mean VDM obtained from continental lava flows [11,13,14,28,31–33,42] (this study – big symbols). Diamonds are mean VADMs obtained from submarine basaltic glass [36]. The closed circles are mean VDM-obtained single plagioclase crystals [38].
Évolution des moments dipolaires virtuels moyens (VDMs) et des moments dipolaires axiaux virtuels (VADMs) entre 5 et 200 Ma. Les carrés représentent les VDM moyens obtenus à partir de coulées de laves continentales [11,13,14,28,31–33,42] (cette étude – grands symboles). Les losanges représentent les VADMs moyens obtenus à partir de verre basaltique sous-marin [36]. Le cercle noir représente le VDM moyen obtenu à partir de monocristaux de plagioclase [38].
Given the large dispersion and the very poor distribution of reliable absolute intensity data, it is hard to draw any firm conclusions regarding the time evolution of the geomagnetic field. As showed by Guyodo and Valet [15], a very large number of records of absolute intensity, reinforced by numerous and precise ages, are necessary to provide a reasonable picture of the field variations trough time.
Acknowledgements
The comments of Mireille Perrin and one anonymous referee on an early version of this manuscript lead to significant improvements of the scientific content and English style of this paper. Economic support for this project has been provided by CONACYT project J32727-T. LA and AG are grateful to financial support given by DGAPA-UNAM grant No. IN100100.
The authors are grateful to Dr Fernando Henríquez for the invaluable help during the field trip.