Abridged version
1 Introduction
Because its high elevation suggests recent fast exhumation, the Mont-Blanc range (4807 m), tallest peak of the Alps, appears to be a good target to study vertical movements of the Alpine belt. The Mont-Blanc range is one of the external crystalline ranges that fringe the Alpine belt. It is composed of a 304±3 Ma granite intruding Hercynian gneiss (Fig. 1). Surprisingly, its structure and its role in the Alpine collision remain discussed. Is the Mont-Blanc massif a crustal culmination above a major thrust ramp [16]? Is it a basement fold at the core of the Helvetic nappes [7,8]? Is it bounded to the northwest by a large thrust [4,6], or by a dextral strike-slip fault [12,15]? Is it an active horst limited to the southeast by a recent or active normal fault [17,22]? To decipher between these hypotheses, we conducted conjoint structural, geochronologic, and gravimetric studies. This article describes the gravimetric results and the first interpretations of the Bouguer anomaly map. The structural study has evidenced that the Hercynian gneiss fringing the Mont-Blanc range to the northwest are affected by a steep southeast-dipping shear zone [18]. This Mont-Blanc shear zone shows a reverse shearing with a small right-lateral component.
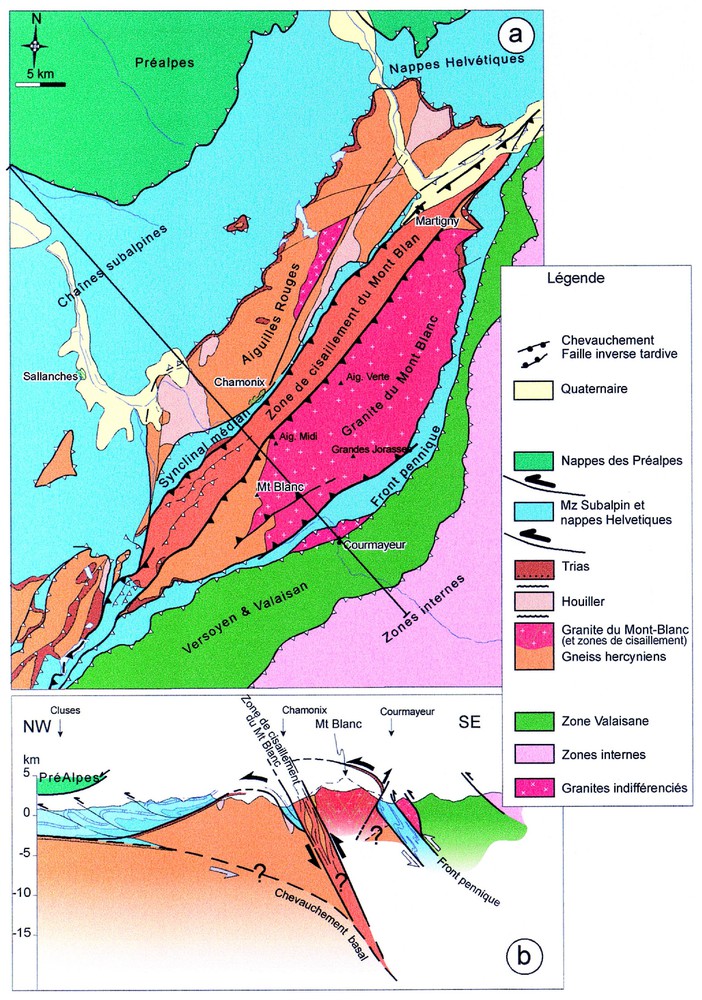
(a) Schéma structural des massifs cristallins externes du Mont-Blanc et des Aiguilles-Rouges ainsi que des formations adjacentes. (b) Coupe géologique simplifiée.
(a) Structural sketch of the Mont-Blanc and Aiguilles-Rouges crystalline external ranges and the adjacent structures. (b) Simplified geological cross-section.
2 Data compilation and data processing
Gravity data were compiled from three sources: (1) the data banks of the ‘Bureau gravimétrique international’ (BGI), (2) unpublished data graciously provided by R. Lanza, of the Milan University, and (3) two GéoFrance3D surveys, performed in 1999 and 2000. These last data have been acquired along roads in the Chamonix and Saint-Gervais valleys (157 data), along hiking pathways in the Aiguilles-Rouges (113 data), and using a helicopter to reach remote areas of the Mont-Blanc range (36 data). For the first time, we dispose of a homogeneously distributed gravity data set of an extremely rugged area. Measurements have been performed using two Lacoste & Romberg and one Scyntrex portable relative gravimeters. Topographic corrections have been calculated assuming a density of 2.67 and using a flat roof prism approximation based on Digital Elevation Model (DEM). The resolution of the DEM decreases with increasing distance from the measurement point: 15, 75, 300, and 1250 m for 0.01 to 1, 1 to 10, 10 to 30 and 30 to 167 km, respectively. The 15 m resolution DEM has been obtained by bicubic interpolation of the 75 m resolution DEM. Uncertainties on gravity measurements and GPS levelling lead to an error of −0.08 mgal on the free-air anomaly [1]. Error on the Bouguer anomaly results from the lack of correction of the 0–10 m topography, errors of the MNTs and the use of a flat roof prism approximation. The total error is probably lower than 2.5 mgal in the more rugged areas and decreases in flatter areas [19].
The gravity effect of a structure is a function of the distance and thus strongly depends on the altitude of the measurement point. Therefore, Bouguer anomaly data in Fig. 2 are not interpolated because of large variation in altitudes. The Bouguer anomaly varies from −167 to −63 mgal (Fig. 2). Along a NW–SE profile, the Bouguer anomaly decreases progressively towards the Peninic front. Most of this large wavelength anomaly can be explained by Moho depth variations. To better image the gravity effect of the crustal structures, we have calculated the Bouguer anomaly corrected of these variations (Fig. 3). For this purpose, we used a three-dimensional interface modelling, based on the Moho geometry defined from seismic data [26].
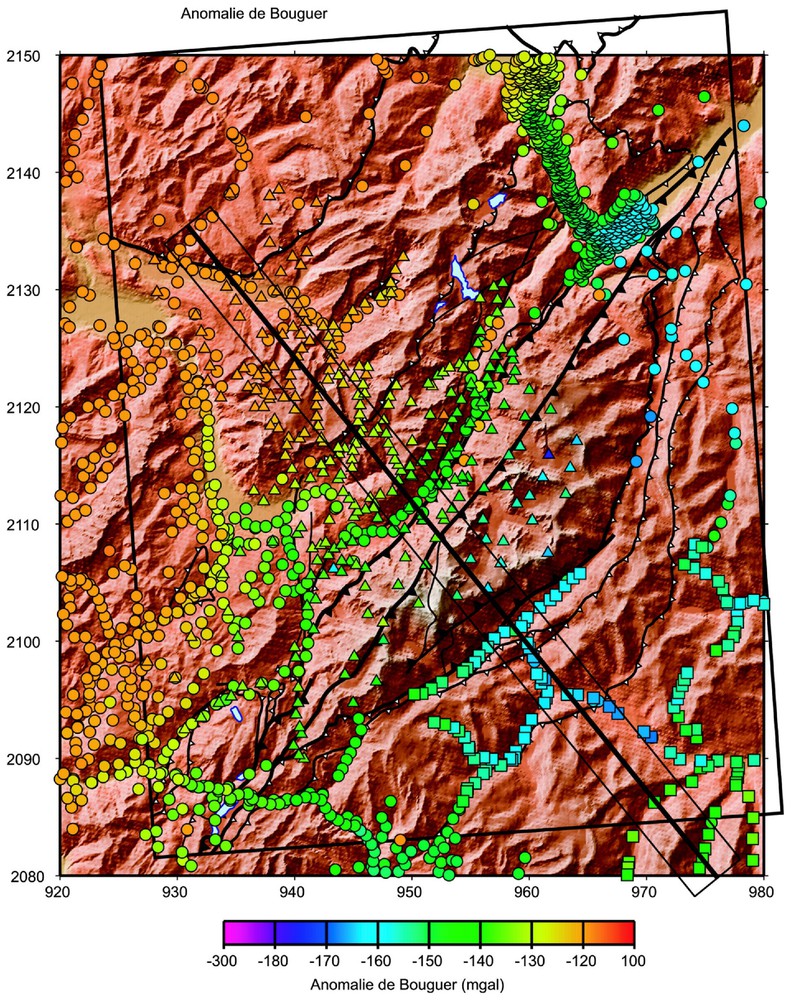
Carte de l'anomalie de Bouguer. Les ronds correspondent aux anciennes données du BGI, les carrés au données gracieusement fournies par R. Lanza, de l'université de Milan, et les triangles aux données acquises durant les nouvelles campagnes. On peut noter la bonne cohérence des trois jeux de données dans les zones où ils sont adjacents. Le grand cadre noir correspond à la carte géologique de la Fig. 1a. Les principales limites géologiques sont représentées. La ligne NW–SE correspond au profil modélisé sur la Fig. 4b. Le petit cadre autour de cette ligne montre la limite des données utilisées pour la modélisation.
Bouguer anomaly map. Circles indicate the former BGI data, squares indicate the data kindly provided by R. Lanza (University of Milan) and triangles indicate the new data. We can note the good consistency of the data in the regions where they are superimposed. The large black frame indicates the location of the map in Fig. 1a. Main geological structures are redrawn. The NW–SE line indicates the profile modelled in Fig. 4b.
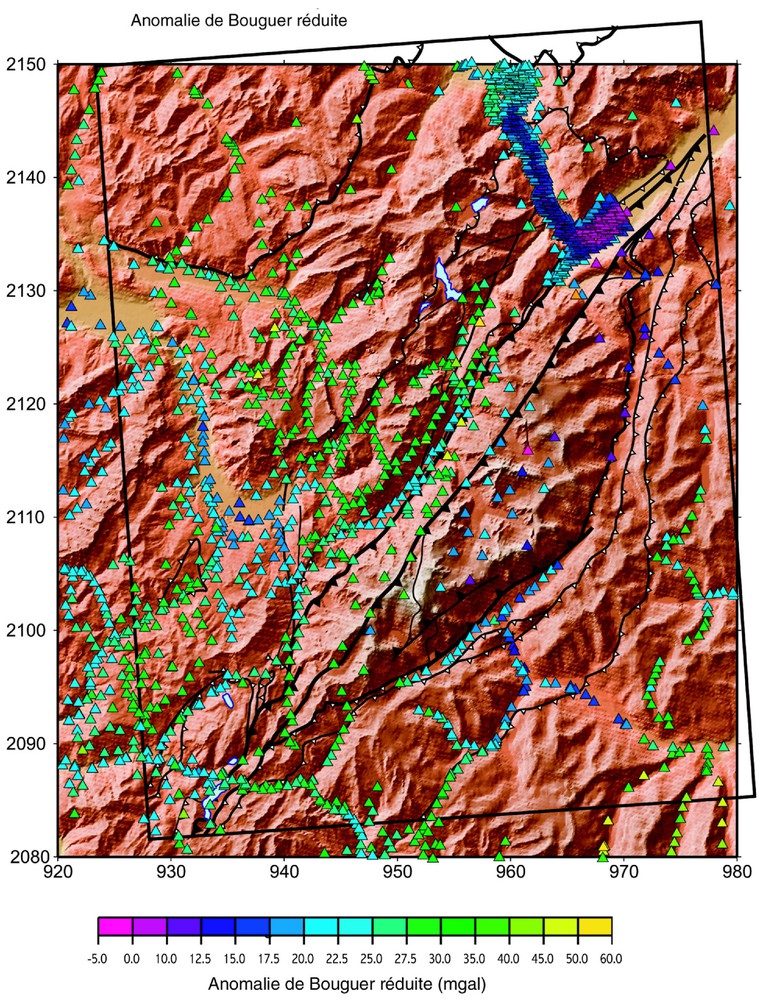
Anomalie de Bouguer résiduelle, c'est-à-dire corrigée de l'effet gravimétrique du Moho, superposée aux principales limites géologiques. L'effet du Moho est calculé à partir du modèle proposé basé sur une compilation de données sismiques [26].
Residual Bouguer anomaly corrected of the Moho depth variations [26], superposed with the main geological boundaries.
3 Gravity effect of the glaciers
The Mont-Blanc Glaciers are among the largest of the Alps, and it is necessary to estimate their gravity effect. The main difficulty for such an estimate is the determination of the ice volume, because data about thickness and geometry of the glaciers are sparse. We have extrapolated some data available for the largest glaciers (‘mer de Glace’, ‘glacier d'Argentière’, etc.) to create synthetic models and calculate the corresponding maximum effect on our gravity measurements. The largest computed effect is −3.68 mgal in the centre of the Mont-Blanc massif, close to the ‘mer de Glace’. Therefore, the anomaly due to geological structures in the centre of the massif is slightly higher than shown in Fig. 2. However, at all measurements points, the difference is smaller than 4 mgal and less than 1 mgal in most cases.
4 Analysis of the Bouguer anomaly and gravity modelling
The Bouguer anomaly map corrected from the effect of the Moho shows a close correlation between the gravity anomaly and the main geological units of the Aiguilles-Rouges and Mont-Blanc ranges (Fig. 3, with low anomalies between −5 and 25 mgal in blue and high anomalies between 25 and 60 mgal in green–yellow). From this map, it appears that:
- – the valleys (Chamonix, Courmayeur, Rhone) exhibit very low anomalies, resulting from Quaternary glacial and alluvial sediments deposits; this shows the importance of measurements outside the valleys in order to constrain the deep crustal structures;
- – the granitic part of the Mont-Blanc massif (from the Mont-Blanc to Martigny) is clearly underlined by a low gravity anomaly (0 to 20 mgal);
- – the Mont-Blanc shear zone and the southwestern part of the massif, mainly gneissic, are correlated to a high anomaly; the limit between the granite and the surrounding rocks is clearly imaged by the gravity data; the granite has certainly a deep root, because only a large volume of low-density material can explain such contrast; in the same way, the Mont-Blanc shear zone, well imaged on the Bouguer anomaly, is probably not confined to the upper part of the crust;
- – the Aiguilles-Rouges range and the ‘chaı̂nes subalpines’ immediately to the north show an anomaly similar to the embedding rocks of the Mont-Blanc range.
Along a 5-km wide NW–SE profile passing through Chamonix and Courmayeur, we have calculated a 2D-gravity model assuming cylindrical symmetry of the structures (Fig. 4). Calculations use the polygon method [23]. The model based on the geometry of the structures at outcrop (geological limits, apparent dip, etc.) and the seismic Moho, was built to minimise the differences with the measurements. Densities assigned to geological formations are consistent with measures performed on numerous sampled rocks. This model shows that:
- – the density of the Mont-Blanc granite (2.62) is lower than the density of the Hercynian gneiss (2.7) and the density of the Mont-Blanc shear-zone (2.72);
- – in the Chamonix valley, a 200 m-thick deposit of Quaternary glacial and alluvial sediments (d=2.2) covers a steep Mesozoic sediments slice (d=2.62);
- – the Mont-Blanc shear zone has a deep root and could offset the Moho by several kilometres.
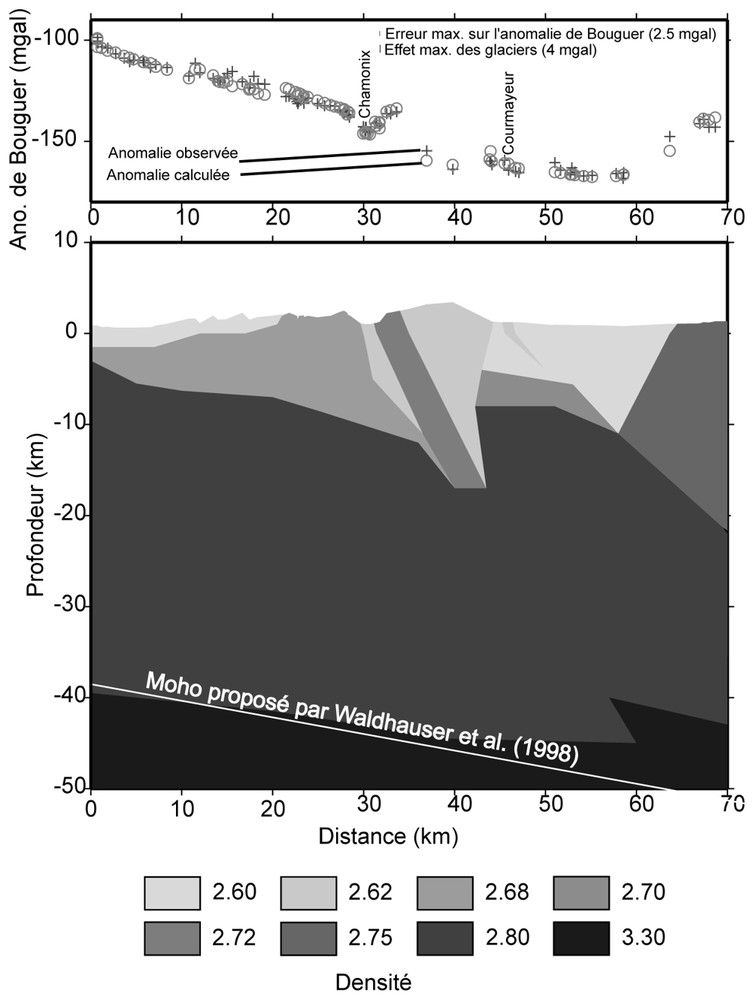
Modélisation de l'anomalie gravimétrique observée le long d'un transect Chamonix–Courmayeur (voir Fig. 2). En haut : anomalie de Bouguer observée (+) et calculée (∘). En bas, le modèle proposé.
Modelling of the gravity anomaly along a transect passing through Chamonix and Courmayeur (see Fig. 2). Top: observed (+) and computed (∘) Bouguer anomaly. Bottom: the density model.
Our model suggests that the Mont-Blanc shear zone is a large reverse fault, responsible for the thrusting of the Mont-Blanc over the Chamonix syncline and the Aiguilles-Rouges range after the emplacement of the Helvetic nappes. The precise kinematics of the shear zone has still to be investigated, but probably partly explains the high elevation of the Mont-Blanc range.
1 Introduction
L'altitude élevée du massif du Mont-Blanc, point culminant de la chaı̂ne alpine (4807 m), suggère une exhumation rapide récente. Ce massif est donc a priori une zone idéale pour l'étude des mouvements verticaux récents de l'orogenèse alpine. L'ensemble des massifs du Mont-Blanc et des Aiguilles-Rouges correspond à une fenêtre de socle granito-gneissique hercynien au cœur de l'empilement des nappes sédimentaires alpines (Fig. 1). Paradoxalement, la structuration de cette fenêtre de socle lors de l'orogenèse alpine reste discutée. S'agit-il d'une culmination crustale au-dessus d'un chevauchement majeur [16] ? Le mont Blanc lui-même est-il un anticlinal de socle, formant le cœur des plis des nappes helvétiques [7,8], ou est-il bordé par un grand chevauchement [4,6] ? Est-il longé par un décrochement dextre [12,15], ou constitue-t-il un horst actif, limité au sud-est par une faille normale récente, voire active [17,22] ?
Pour répondre à ces questions, une triple étude (cartographie des structures géologiques des massifs du Mont-Blanc et des Aiguilles-Rouges, études géochronologiques et études gravimétriques) a été réalisée dans le cadre du programme GéoFrance 3D Alpes. Cet article présente les premiers résultats de l'étude gravimétrique.
La connaissance de l'anomalie de Bouguer dans les Alpes occidentales a été définie comme l'une des priorités du programme GéoFrance 3D Alpes, car elle permet une première caractérisation 3D des structures géologiques. Si les vallées et les reliefs de faibles amplitudes ont fait l'objet de nombreux travaux dans les années 1980–1990, notamment lors de la réalisation du profil ECORS [2,20,21], les zones les plus accidentées sont restées très peu couvertes. À la fin des années 1990, de nouvelles mesures ont été effectuées pour combler ces lacunes, principalement dans les massifs situés du Pelvoux à la Méditerranée [19]. L'étude ici présentée, centrée sur le massif du Mont-Blanc, est la première réalisée à petite échelle, au cœur d'un massif de haute montagne. Nous insisterons sur deux problèmes qui se posent lorsqu'on réalise des études gravimétriques en haute montagne, le problème des corrections topographiques et celui de la prise en compte des glaciers.
2 Présentation de la zone d'étude : les massifs du Mont-Blanc et des Aiguilles-Rouges
Les massifs du Mont-Blanc et des Aiguilles-Rouges sont localisés à l'ouest du front Pennique, qui marque la limite entre les Alpes internes et externes (Fig. 1a). Ils font partie des massifs cristallins externes (MCE), que l'on retrouve tout au long de l'arc Alpin. Le massif du Mont-Blanc est essentiellement constitué d'un granite daté à 304±3 Ma [3]. Ce granite est intrusif dans des gneiss hercyniens qui affleurent largement à la bordure nord-est et à l'extrémité sud du massif du Mont-Blanc et dans le massif des Aiguilles-Rouges (Fig. 1). Localement, ces gneiss sont recouverts par des dépôts carbonifères, regroupés sous l'appellation de « Houiller » (conglomérats et schistes noirs), qui forment des synclinaux (Fig. 1). Des grès triasiques reposent en discordance sur les gneiss et le Houiller. L'ensemble de ces terrains constitue le socle au-dessus duquel la couverture sédimentaire mésozoı̈que est plissée et décollée vers le nord-ouest, pour constituer les nappes helvétiques et les chaı̂nes subalpines (Fig. 1b). La discordance et le niveau de décollement forment un anticlinorium au cœur duquel le socle apparaı̂t en fenêtre au cœur des nappes helvétiques (Fig. 1b). Les massifs du Mont-Blanc et des Aiguilles-Rouges sont séparés par une étroite bande de terrains sédimentaires mésozoı̈ques, le synclinal médian. Les données structurales et géochronologiques que nous avons acquises par ailleurs montrent que les gneiss et les granites hercyniens de la bordure nord-ouest du mont Blanc ont été déformés lors de l'orogenèse alpine dans une zone de cisaillement, la zone de cisaillement du Mont-Blanc [18] (Fig. 1). Cette zone de cisaillement montre un jeu inverse, avec une légère composante dextre. Elle a accommodé le chevauchement du mont Blanc sur le synclinal médian et le massif des Aiguilles-Rouges. À l'affleurement, la zone de cisaillement est exprimée par une schistosité alpine plongeant de 55 à 75° vers le sud-est [18] (Fig. 1b). Au sein de la zone de cisaillement, les déformations hercyniennes ont localement été préservées au cœur de grands boudins peu déformés à l'Alpin (Fig. 1b). Le versant sud-est du massif correspond à la racine des nappes helvétiques, localement recoupées par un rétro-chevauchement, qui souligne les plus hauts sommets du massif entre le mont Blanc et les Grandes Jorasses (Fig. 1).
3 Les données gravimétriques
Les données gravimétriques disponibles dans la zone d'étude avant la campagne GéoFrance 3D étaient peu denses dans les massifs du Mont-Blanc et des Aiguilles-Rouges, plus denses dans le massif du Beaufortin et en Suisse, mais avec une répartition inhomogène (principalement le long des axes routiers, dans les vallées). Mille cent quatre-vingt-deux mesures préexistantes, rassemblées au sein de la banque de données du Bureau gravimétrique international, ont été utilisées. Pour compléter et densifier cette base de données, les approches suivantes ont été utilisées :
- – densification des mesures le long des axes routiers, notamment au nord de Chamonix, dans la région de Saint-Gervais–Megève et de Samoëns–Sixt, en 1999 et 2000 (157 mesures) ;
- – mesures le long de sentiers de grande randonnée, surtout dans le massif des Aiguilles-Rouges ; une quinzaine d'itinéraires ont ainsi été suivis (113 mesures) ;
- – mesures héliportées pour les points difficilement accessibles à pied, surtout dans le massif du Mont-Blanc (36 mesures) ; ces mesures ont permis l'obtention d'une couverture relativement homogène de l'ensemble du massif du Mont-Blanc, y compris dans ses zones les plus élevées.
De plus, 121 mesures effectuées en Italie et non publiées nous ont été gracieusement fournies par R. Lanza, de l'université de Milan. Un ensemble de 1609 données a donc été utilisé pour cartographier le champ de pesanteur sur le massif du Mont-Blanc et les régions avoisinantes.
Les nouvelles mesures ont été réalisées avec deux gravimètres Lacoste et Romberg et un gravimètre Scintrex CG3. La précision intrinsèque de ces appareils est de l'ordre du centième de mgal, ce qui a pu être vérifié pour les stations occupées à plusieurs reprises, une fois les corrections de marée et de dérive instrumentale effectuées. Toutes les mesures ont été rattachées à la base BRGM de premier ordre I4 du tunnel du Mont-Blanc, aucune mesure de la pesanteur absolue n'ayant été effectuée lors de cette campagne. Chaque nouveau point de mesure a fait l'objet d'une localisation à l'aide de récepteurs GPS. Chaque jour, les coordonnées d'une base située à Chamonix ont été calculées en utilisant cinq stations permanentes du réseau REGAL (Joux, La Feclaz, Modane, Saint-Jean-des-Vignes et Le Châtel). Les points de mesures ont ensuite été localisés par rapport à la base de Chamonix. Les hauteurs ellipsoı̈dales ainsi calculées ont été converties en altitudes normales, en utilisant le modèle de quasi-géoı̈de RAF 96 [5]. La précision verticale sur le positionnement est d'environ 25 cm [1]. L'ensemble de données brutes et corrigées (voir ci-dessous) sont disponibles sur le site http://www.dstu.univ-montp2.fr/PERSO/fmasson/index.html.
4 Calcul de l'anomalie de Bouguer
Les mesures de pesanteur sont interprétées dans le système IGSN71. Le calcul des corrections topographiques est le problème majeur lors du calcul de l'anomalie de Bouguer. La méthode employée consiste à approcher la forme des reliefs en les modélisant par des prismes à toit plat, en employant une densité de réduction uniforme de 2,67. L'utilisation de cette valeur, légèrement supérieure à la densité moyenne des échantillons prélevés sur le terrain, rend aisée la comparaison de nos résultats avec ceux obtenus dans d'autres régions de France. La méthode est fondée sur l'utilisation des abaques de Hammer [13] systématisée grâce à l'emploi de Modèles Numériques de Terrain. Pour rendre compte le plus fidèlement possible de la topographie autour de chaque point de mesure, trois MNT ont été nécessaires. Dans la couronne la plus éloignée du point de référence (30 à 167 km), la maille de 1250 m du MNT ETOPO30 a été utilisée. Dans les secteurs suivants (10 à 30 km puis 1 à 10 km), les MNT ont des pas plus faibles, respectivement 300 m et 75 m. La couronne suivante, s'étendant entre 1 km et 10 m, est celle où la correction est la plus importante, donc celle où le rendu de la topographie doit être le meilleur possible. Un MNT de 15 m, obtenu par interpolation bicubique du MNT de 75 m, a donc été employé. Un test synthétique a été effectué pour tester cette procédure. Un MNT synthétique d'un pas de 15 m a été créé et les corrections topographiques calculées en 400 points. Ensuite, ce MNT de 15 m a été sous-échantillonné à un pas de 75 m. Enfin, le MNT de 75 m a été réinterpolé au pas de 15 m, de façon bilinéaire et bicubique. Pour ces trois derniers MNT (75 m, 15 m bilinéaire et 15 m bicubique), les corrections topographiques ont été recalculées. Il apparaı̂t alors que l'écart avec la correction topographique initiale est minimal (moyenne : −0,5 mgal) en utilisant le MNT de 15 m, réinterpolé de façon bicubique. L'interpolation bicubique permet de minimiser les erreurs réalisées lors du calcul de l'effet topographique proche. Entre 0 et 10 m, on considère la topographie comme plane, cette condition étant respectée pour la plupart des points de mesure.
L'erreur sur l'anomalie à l'air libre dépend essentiellement de l'erreur sur l'altitude de la station. Elle est estimée à 0,3 mgal pour les points cotés et à 0,08 mgal pour les points GPS [1]. L'absence de correction proche de 0 à 10 m, l'incertitude sur les MNT et l'utilisation pour les calculs de prismes à toit plat constituent les trois causes d'erreur sur les corrections pour le calcul de l'anomalie de Bouguer. Tout cela induit une erreur de 2,5 mgal au maximum pour les points situés dans les zones les plus accidentées et une erreur bien moindre dans les zones plus planes [19].
L'anomalie de Bouguer calculée en chaque point de mesure est représentée sur la Fig. 2. Les données sont présentées de manière ponctuelle, le tracé d'isocontours n'étant pas justifiable dans le cas de données acquises à des altitudes fortement variables. L'effet gravimétrique d'une structure profonde est, en effet, dépendant de la distance entre cette structure et le point de mesure, et donc fonction de l'altitude. Seul un prolongement permettrait la réalisation d'une carte. Celui-ci est malheureusement difficile à effectuer dans notre cas, du fait de la non-homogénéité des données.
L'anomalie de Bouguer varie entre −167 mgal et −63 mgal (Fig. 2). Elle est maximale au nord-ouest de la zone étudiée, diminue progressivement vers le sud-est avant de ré-augmenter légèrement à l'extrême sud-est. Les valeurs les plus faibles se trouvent dans la zone interne valaisanne. Cette variation de grande longueur d'onde de l'anomalie est principalement liée à l'approfondissement progressif du Moho vers le sud-est. Cet effet de grande longueur d'onde du Moho est suffisamment important pour masquer certains effets crustaux. L'anomalie de Bouguer peut être corrigée des effets du Moho, dont la profondeur est relativement bien contrainte à grande échelle par les données sismiques [26]. La méthode utilisée est identique à celle utilisée dans un précédent article, décrivant la réalisation d'une carte gravimétrique des Alpes [19]. L'anomalie de Bouguer réduite correspondante (Fig. 3) présente l'avantage de refléter principalement les hétérogénéités crustales.
5 Effet gravimétrique des glaciers
Avant d'interpréter plus précisément ces observations, il est apparu important d'évaluer l'effet gravimétrique des nombreux glaciers du massif du Mont-Blanc. La difficulté majeure pour étudier ce problème est la détermination des volumes de glace en jeu. Effectivement, les données relatives à l'épaisseur des glaciers sont peu nombreuses et très ponctuelles. Elles concernent surtout les glaciers majeurs : mer de Glace [11], glacier d'Argentière [14], glacier du Tacul [24] et dôme du Goûter [25]. Pour rendre compte de la géométrie réelle des glaciers, les contours des glaciers ont été numérisés. Afin d'évaluer un effet maximal, nous avons retenu les épaisseurs les plus fortes proposées par les auteurs pour les glaciers majeurs. Les autres épaisseurs ont été fixées à partir d'une analyse cartographique des sites concernés, en émettant les hypothèses suivantes : les glaciers de vallée ont les profondeurs les plus conséquentes (mer de Glace, glacier d'Argentière, du Tacul), les cirques glaciaires et les glaciers de calotte ont des profondeurs fonction de leur diamètre, et les petits glaciers de couloir font quelques dizaines de mètres, tout au plus. L'anomalie gravimétrique due aux glaciers a été calculée pour chacune des 1609 mesures gravimétriques. L'anomalie est maximale (−3,68 mgal) au cœur du massif, au voisinage immédiat du triptyque glacier de Leschaux–glacier du Tacul–mer de Glace et diminue graduellement, lorsque l'on s'éloigne du massif. Elle devient rapidement nulle en dehors des massifs du Mont-Blanc. L'anomalie au cœur du massif due aux structures géologiques est donc certainement légèrement supérieure à l'anomalie de Bouguer, calculée et présentée sur la Fig. 2. Cependant, l'effet maximum sur la valeur de l'anomalie de Bouguer est de l'ordre de 3 mgal et les grandes tendances observées sur les cartes de l'anomalie de Bouguer et de l'anomalie de Bouguer corrigée de l'effet du Moho ne peuvent être significativement modifiées.
6 Analyse des cartes d'anomalies de Bouguer et modélisation gravimétrique
À grande échelle, nous avons vu que l'anomalie de Bouguer était directement corrélée à la variation de la profondeur du Moho. L'anomalie de Bouguer réduite de l'effet du Moho est comprise entre −5 et 60 mgal (Fig. 3). Les anomalies « faibles », en bleu sur la Fig. 3, sont comprises entre −5 et 25 mgal. Les anomalies « élevées », en vert sur la Fig. 3, sont comprises entre 25 et 60 mgal. Les principales structures observables sur la carte d'anomalie de Bouguer réduite sont très fortement corrélées aux principales structures géologiques. On peut faire les observations suivantes.
- – Les vallées (vallée de Chamonix en France, vallée de Courmayeur en Italie ou vallée du Rhône en Suisse) sont caractérisées par des anomalies très faibles, proches de zéro (entre −5 et +25), résultant principalement des remplissages glaciaires et alluviaux, dont la densité contraste avec celle des massifs bordiers. Ceci montre l'importance, lorsque l'on effectue des campagnes gravimétriques en montagne, de réaliser des mesures en dehors des vallées, car les mesures en vallée informent surtout sur la quantité de dépôts quaternaires et peu sur les structures profondes. Par exemple, dans la vallée du Rhône, près de Martigny, les mesures gravimétriques permettent de bien visualiser la géométrie du remplissage glaciaire (Fig. 3). Des études détaillées combinant gravimétrie et sismique réflexion ont mis en évidence un remplissage glaciaire compris entre 400 m dans la haute vallée du Rhône et 1000 m près du Léman [9,10].
- – La partie granitique du massif du Mont-Blanc (du sommet du mont Blanc au sud de Martigny) est caractérisée par une anomalie faible (entre 0 et 20 mgal). Cette anomalie n'est que très faiblement due à l'influence des glaciers (voir ci-dessus).
- – La zone de cisaillement du Mont-Blanc ainsi que la partie sud-ouest du massif, principalement gneissique, présentent des anomalies plus élevées que le massif granitique. La limite entre le granite et les encaissants est soulignée par l'anomalie gravimétrique. Le granite semble s'étendre en profondeur, car seul un volume important de granite peut expliquer le contraste observé avec l'encaissant. De même, la zone de cisaillement du Mont-Blanc est très nettement imagée par l'anomalie de Bouguer réduite, ce qui laisse à penser qu'elle ne se limite pas à la seule partie supérieure de la croûte.
- – Au nord de la vallée de Chamonix, le massif des Aiguilles-Rouges ainsi que la zone correspondant aux couches calcaires mésozoı̈ques du désert de Platé et de l'ensemble de la nappe de Morcles sont marqués par une anomalie positive, proche de celle observée pour l'encaissant gneissique du massif du Mont-Blanc. Cette anomalie est très uniforme, soulignant une grande homogénéité géologique.
Pour discuter la géométrie à courte longueur d'onde des unités crustales et du Moho, nous avons choisi de modéliser l'anomalie de Bouguer non corrigée de l'effet du Moho ni de l'effet des glaciers le long d'un profil orienté NW–SE, perpendiculaire aux directions structurales (Fig. 2). L'ensemble des données se trouvant à moins de 2,5 km de part et d'autre de ce profil passant par Chamonix et la vallée de Courmayeur ont été retenues (Figs. 2 et 4a). La modélisation est à deux dimensions, en faisant l'hypothèse d'une symétrie cylindrique des structures, hypothèse raisonnable à cet endroit du massif. Elle est effectuée à partir de la méthode des polygones [23]. La solution n'est pas unique, mais la géométrie présentée sur la Fig. 4b est déduite de l'observation des structures en surface (limites géologiques de la Fig. 1) et de la connaissance a priori du Moho, modifiée pour ajuster le mieux possible l'ensemble des données. Les densités proposées sont cohérentes avec les mesures faites sur des échantillons prélevés en surface et à l'intérieur du tunnel du Mont-Blanc. Les points essentiels du modèle sont :
- – le granite du Mont-Blanc a une densité (d=2,62) plus faible que celle des gneiss Hercyniens encaissants (d=2,7 à proximité de la surface et d=2,8 plus en profondeur) et que ceux de la zone de cisaillement du Mont-Blanc (d=2,72) ;
- – la vallée de Chamonix est marquée par un remplissage glaciaire de 200 m de profondeur (d=2,2) sous lequel se trouve une lame de terrains sédimentaires mésozoı̈ques (d=2,62) ;
- – la zone de cisaillement de Chamonix, qui sépare cette lame du granite, se prolonge en profondeur et semble décaler le Moho de plusieurs kilomètres ;
- – la remontée progressive du Moho et du socle vers le nord-ouest explique la remontée de l'anomalie de Bouguer dans cette direction.
7 Conclusion
L'analyse des cartes de l'anomalie de Bouguer des massifs du Mont-Blanc et des Aiguilles-Rouges et la modélisation d'un profil NW–SE nous conduit à proposer que la zone de cisaillement du Mont-Blanc se prolonge en profondeur jusqu'au niveau du Moho. Cette grande faille inverse responsable du chevauchement du Mont-Blanc sur le synclinal de Chamonix et le massif des Aiguilles-Rouges serait donc une structure majeure postérieure à la mise en place des nappes helvétiques, qui aurait été négligée jusqu'à présent. La cinématique de cette structure doit être précisée, mais expliquerait, au moins en partie, l'altitude élevée du Mont-Blanc et aurait des conséquences importantes sur les mécanismes d'épaississement dans les Alpes externes.
Remerciements
Les auteurs remercient l'ensemble des participants aux campagnes d'acquisition des nouvelles données gravimétriques (Roger Bayer, Philippe Collard, Marc Daignières, Erik Doerflinger, Robin Lacassin, Francis Lucazeau, Jean-Patrick Respaut, Michel Sarrailh, Jérôme Verdun) ainsi que Emile Klingelé (ETH Zurich) pour le prêt d'un gravimètre Lacoste et Romberg. Ils remercient aussi le peloton de gendarmerie de haute-montagne (PGHM) de Chamonix, qui a assuré la sécurité des opérateurs gravimétriques lors des mesures héliportés. Ils remercient enfin Pascal Brun, pilote d'hélicoptère de la compagnie Mont-Blanc Hélicoptère (CMBH), qui a permis à la campagne héliportée de se dérouler dans d'excellentes conditions. Cette étude a pu être réalisé grâce au soutien du programme national GéoFrance3D. Anne-Claire Morillon a réalisé la Fig. 1.