Version française abrégée
1 Introduction
La meilleure compréhension de l'organisation spatiale des sols affectés par un excès d'eau est essentielle pour la gestion des paysages, la modélisation de l'hydrologie ou de l'érosion. Dans les zones de fonds de vallée, où ces sols hydromorphes (Fluvisol) sont rencontrés, l'excès d'eau est un des facteurs qui contrôle la genèse du ruissellement de surface, l'érosion des sols, le lessivage des nutriments et des pesticides ainsi que la dénitrification des eaux. Ces sols sont donc des acteurs essentiels de la dynamique des fonds de vallée. La représentation fine de leur organisation spatiale est relativement difficile à obtenir en raison de la nécessité d'études pédologiques très détaillées, laborieuses et coûteuses du fait de la nécessité d'un nombre important d'observations. Les méthodes géophysiques ont déjà démontré leur intérêt pour la caractérisation continue, rapide, non destructive et peu onéreuse des sols à travers les paysages (par exemple, [3,6,8,11,15,17,20]). Cependant, dans les fonds de vallée, des difficultés sont rencontrées en raison des grandes variations d'humidité, de texture et de profondeur des sols [6,18]. En effet, les variations respectives de ces différents paramètres peuvent susciter des signaux géophysiques ambigus, qui interdisent la discrimination des sols. Par exemple, une diminution du contenu en eau, qui se traduit par une augmentation de la résistivité électrique, peut être contrebalancée par une augmentation de la teneur en argile, qui se traduit, au contraire, par une diminution de la résistivité électrique. Nous montrons ici que la combinaison de techniques géophysiques nécessitant une mise en œuvre aisée et un post-traitement limité permet d'optimiser la prédiction spatiale des sols affectés par un excès d'eau. Trois techniques géophysiques, la radio-magnétotellurique (RMT), le radar géologique (GPR) et le quadripôle électrostatique (ESQP) sont mises en oeuvre dans le Massif armoricain (Ouest de la France) le long d'un transect représentatif où les sols sont finement décrits.
2 Matériels et méthodes
L'aire d'étude est présentée sur la Fig. 1. Il s'agit d'une zone de fond de vallée de 2 ha environ, située au sein d'un bassin versant (« La Roche ») de 7,8 km2. Les versants sont courts (150 à 250 m) et peu pentus. Les pentes varient de 0 à 2 % en bas et au sommet des versants à 6 % au milieu. L'étude a pris place le long de huit transects perpendiculaires aux courbes de niveau allant du cours d'eau principal au sommet de pente. Le long de chaque transect, un échantillonnage pédologique régulier (10 m) par sondage à la tarière est initialement implanté, puis complété par des observations secondaires aux lieux des variations latérales de sol. La nature des horizons, leur épaisseur et leur texture sont repérées. Le long de ce transect, la RMT, le GPR et le ESQP sont mis en œuvre à la surface du sol. La RMT est utilisée à la fréquence de 162 kHz (comme proposé par Lagabrielle [17], pour les études de sol) avec une distance entre les capteurs telluriques de 0,7 m [10]. Les données sont ensuite analysées afin d'estimer la résistivité apparente des matériaux en suivant la procédure définie par Lagabrielle [17]. Le GPR (Subsurface Interface Radar SIR System-8, Geophysical Survey Systems, Hudson NH) est mis en œuvre à la fréquence de 500 MHz. Le signal obtenu est présenté en section temps. Le ESQP (type ELCORE développé par SELVA et LCPC) est utilisé à une fréquence de 10 kHz et une intensité de 1 mA.

Location of the study site in the Armorican Massif (‘La Roche’ Catchment, western France).
Localisation du site d'étude dans le Massif armoricain (bassin de « la Roche », Ouest de la France).
Lors de l'étude géophysique, une évaluation des teneurs en eau du sol à différentes profondeurs est obtenue par TDR (couche 0–10) et par pesée d'échantillons au laboratoire (10–20 à 110–120 cm).
3 Résultats et discussions
L'étude des transects, montre que la couverture de sol est composée d'une association de fibric Fluvisols, gleyic Fluvisols et Albeluvisols [26] se succédant depuis les berges du cours d'eau jusqu'au pied de versant. La distribution spatiale des sols le long d'un transect représentatif est présentée en Fig. 2A. Les fibric Fluvisols présentent un horizon composé de matériel organique partiellement ou non décomposé. Les gleyic Fluvisols localisés immédiatement en amont, présentent des horizons minéraux limoneux à sableux « oximorphiques » (fluvic CGF1) et « reductomorphiques » (fluvic CGF3). En amont d'un fossé, apparaissent les Albeluvisols et leurs horizons diagnostiques blanchis, éluvial (albic horizon, Ea) et brun foncé illuvial (argic horizon, BG).
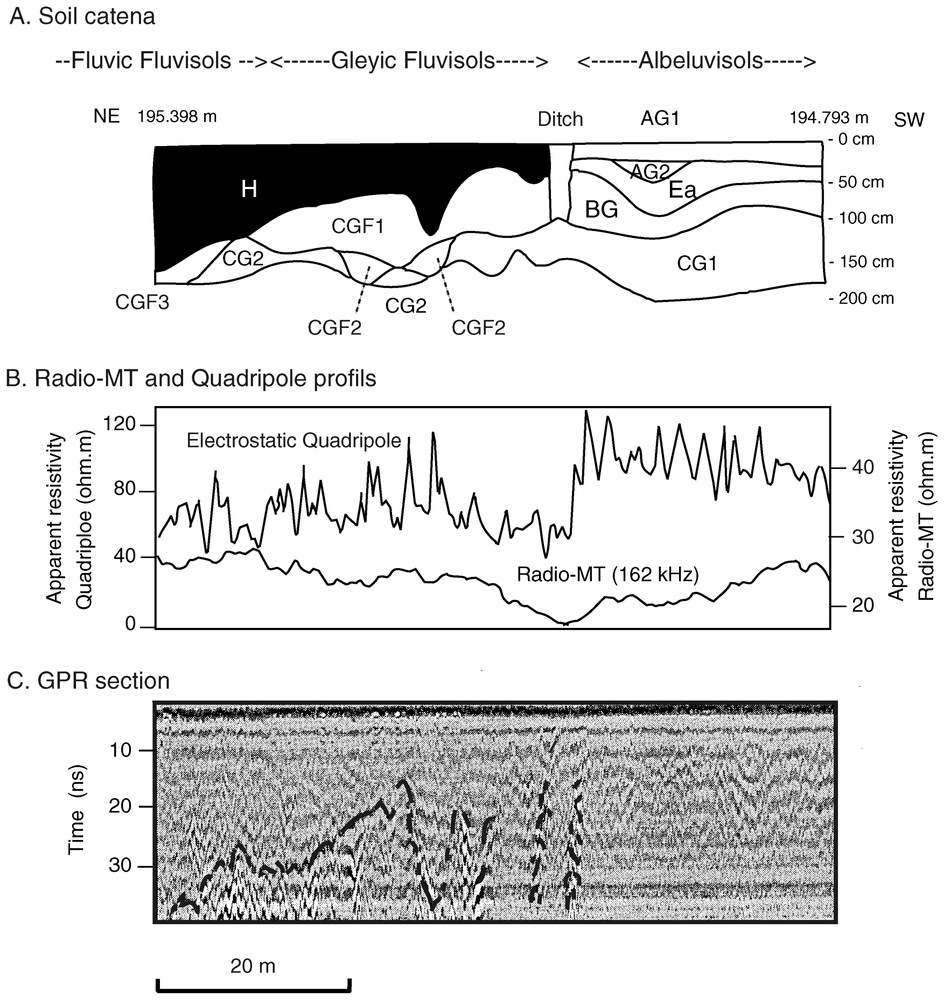
Soil and geophysical investigations along one representative transect from the stream bank (left) to the footslope position (right). A: Soil organisation. B: Apparent resistivity profiles measured with radio-MT and electrostatic quadrupole. C: Raw radargram (time section).
Recherches sur les sols et la géophysique le long d'un transect représentatif de la rive du fleuve (à gauche) jusqu'à la position de bas de pente (à droite). A : Organisation du sol. B : Profils de résistivité apparente, mesurés par radio-MT et quadripole électrostatique. C : Diagramme radar brut (coupe en fonction du temps).
Les Fig. 2B et C présentent les profils RMT et ESQP ainsi que les coupes temps GPR pour ce même transect. La résistivité apparente obtenue avec la RMT varie de 16 à 28 Ω m. Les valeurs maximales observées aux deux extrémités du transect décroissent jusqu'au fossé central. Les variations de résistivité apparente semblent surtout correspondre aux variations d'épaisseur de la couverture de sol et non à celles des types de sol. En effet la profondeur de pénétration de cette méthode dépasse la base de la couverture et englobe largement l'altérite et la roche sous-jacentes. Ceci confirme néanmoins l'apport de cette technique dans les fonds de vallée démontrée par Mérot et al. [18] et Chaplot et al. [6].
En complément, le ESQP permet de distinguer très nettement les deux grands domaines : les fibric et gleyic Fluvisols, d'une part, et les Albefluvisols, d'autre part. Finalement, les réflecteurs majeurs identifiés sur la coupe temps du GPR semblent correspondre à la base des horizons organiques ainsi qu'aux faces du fossé. Les horizons organiques sont ici d'excellents réflecteurs en raison probablement de la faculté des matériaux riche en matière organique à retenir une quantité d'eau bien plus grande que les matériaux limoneux (voir, par exemple, [25]).
Au total, la combinaison de ces trois techniques géophysiques permet d'obtenir une représentation continue satisfaisante des principaux traits de l'organisation des sols de fonds de vallée affectés par un excès d'eau.
1 Introduction
In bottomland areas, soil types or soil horizons, defined from the occurrence and intensity of redoximorphic features, allow the spatial prediction of the duration of soil saturation by water or reducing conditions. A better understanding of soil spatial variations in landscapes is fundamental for land use planning, ecological, hydrological and erosion modelling. In bottomland areas, soils are indeed important regulators of water, nutrients and sediment fluxes within landscapes [2]. Due to an excess of water, these soils determine the production of surface runoff, which is the driving mechanism for soil erosion, nutrient or pesticides losses in hillslopes, and for flooding in downstream areas. In addition, such soils show a nitrogen buffering capacity, which is controlled by two main processes: microbial denitrification, and microbial and plant uptake that protect river channels from agricultural diffuse pollution [13]. Because the direct assessment of all these processes over large areas is difficult, soils are thus used to predict indirectly bottomlands functioning.
A detailed description of soil spatial distribution in landscapes is generally difficult to achieve, since soil investigations performed using Auger hole and laboratory analysis are faced with both methodological and financial constraints. Such procedures are indeed very costly, since they require a large number of individual analyses, especially in areas with a high degree of short-range variability.
Numerous studies have aptly pointed out that geophysical methods allow us to characterise soils continuously at lower cost. For example, soils are successfully delineated using the radio-magnetotelluric resistivity (RMT) (e.g., [3,6,17,20,24]), ground penetrating radars (GPR) (e.g., [1,8,9,11]), electromagnetic induction methods (e.g., [14,15]) or multielectrode resistivity imaging (e.g., [7,12,21]). In some extent, a combination of geophysical techniques was proven to increase the soil prediction accuracy. This was successfully tested in loessial soils at upslope positions by using ground-penetrating radar (GPR) and electromagnetic induction (EMI) techniques in combination to gather soil morphological information [15]. These authors concluded that “using precursory EMI data to pinpoint GPR surveys is a precise, accurate, and rapid means of acquiring field-scale soil morphological information”.
But in bottomland areas, much more difficulties are expected in relating geophysical properties to soil distribution due to large variations of soil depth [18], soil texture [5,23] or soil water content [3]. Chaplot et al. [5] showed for instance the necessity to take into account soil properties such as the soil water content and the upper boundary of saprolite to evaluate the spatial prediction of redoximorphic soils through RMT. The best correlations were between the electrical conductivity and the depth to the upper boundary of saprolite, and the topsoil water content (r=0.54 and r=0.65, respectively). But high prediction errors remained in discriminating soil with higher durations of saturation conditions. In addition, the shape of limits between soil horizons and especially between the soil cover and the indurated saprolites, directly affecting water pathways, was not accurately estimated [4].
Our objectives were (i) to conduct a complete soil morphological characterisation by soil science investigation, (ii) to conduct a non-intrusive soil investigation by geophysical methods, and (iii) to compare the results from the traditional investigation with the non-intrusive investigations. Three geophysical techniques, the RMT, the GPR and the ESQP were implemented in a bottomland of Armorican Massif (western France) to precisely delineate soils and horizons. We show here that increased accuracy in delineating soils affected by an excess of water may be obtained by using a combination of geophysical techniques requiring a limited amount of fieldwork and data processing.
2 Materials and methods
2.1 Study site and soil investigation
The study area is located in the eastern part of the Armorican Massif (western France, 20 km west of Avranches, Fig. 1), a complex basement of Proterozoic- and Palaeozoic-age bedrock. It is an agricultural field of about 2 ha within a granitic catchment, the ‘La Roche’ catchment (7.8 km2). This catchment is characterised by 150 to 250 m long hillslopes with a mean elevation range of 10 m from the stream bank to the slope summit. Hillslope topography is relatively smooth, with slope angles varying from 0 to 2% close to the stream bank and on summit to 6% backslope.
The bottomland planted with corn is situated along a characteristic hillslope of the ‘La Roche’ catchment.
Soil sampling at the study area was performed on a regular grid with a mesh of 10 m. This regular grid defined 8 transects from the top of the slope to the channel network with a length of 150 m. Transects are 10 m distant from each other. Intermediary observations were performed to precise soil variations. On each of the 182 points defined, the following parameters were recorded: (i) type and thickness of horizons, (ii) texture, (iii) soil water content at 20, 40 and 60 cm depths (W20, W40, W60) measured at the lab on small samples; and (iv) surface (0–10 cm) volumetric soil water content (θ10) determined by Time-Domain Reflectometry (TDR). In order to describe and analyse the encountered soils, the study of the area was completed by five pits.
2.2 Geophysical methods and investigation
Three geophysical methods were considered here. The first method, the RMT, is an electromagnetic technique derived from the VLF method. According to Keller and Frischnecht [16], Parasnis [19] and Telford et al. [22], the measurement consists in obtaining both the relations between the amplitudes of the Ex and Hy components and their relative phase. The resistivity is calculated following Eq. (1):
(1) |
The depth of investigation of this method, noted D (m), is given by Eq. (2):
(2) |
The GPR and the ESQP techniques are the two other methods used. The GPR used is a bi-static ‘Subsurface Interface Radar’ (SIR) System-8 (Geophysical Survey Systems, Hudson, NH), operated at a 500 MHz-centred frequency. The antennas offset used is 30 cm. The GPR results are presented as raw-time sections.
The ESQP is an ELCORE type, developed by SELVA and LCPC. It is run at a frequency of 10 kHz and an intensity of 1 mA, with an AMNB electrode configuration. The AB and MN distances were 1 and 5.3 m, respectively.
RMT and ESQP results are presented as raw apparent resistivity profiles.
Measurements using these three techniques are presented for one representative transect where soils are precisely delineated.
3 Results
3.1 Soil distribution
Fig. 2 describes the lateral variations of redoximorphic soils over the characteristic transect. The soil cover is formed by an association of fibric, gleyic Fluvisols, and Albeluvisols [26], distributed in the bottomland from the stream bank to the foot of the hillslope (Fig. 2A). The fibric and gleyic Fluvisols occur at the vicinity of the stream bank. They are characterised by the presence of a dark (10YR2/1 to 10YR3/1) H horizon resulting from undecomposed or partially decomposed organic material. In fibric Fluvisols, H horizons predominate. The entire soil profile is marked by an organic carbon (OC) stock of 366 kg m−2 [5]. Mineral light grey sandy horizons CGF3 composed of thin strata of fine sand with less organic carbon are topped by H horizons. Mineral horizons with oximorphic properties and loamy texture (fluvic CGF1) or reductomorphic properties with sandy texture (fluvic CGF3) are observed upslope in gleyic Luvisols. An oximorphic saprolite (CG2) is observed under these horizons. Albeluvisols occur upslope a ditch cutting the soil cover. These soils are characterised by a bleached (10YR5/1) eluvial horizon (‘albic horizon’, Ea), a strong brown (7.5YR4/6) to grey (10Y8/1) clay-enriched horizon (argic horizon, BG) and the occurrence of albeluvic tonguing. Under these horizons, a reductomorphic sandy saprolite is found.
3.2 Relation between geophysical properties and soil spatial distribution
Along the transect of Fig. 2, the apparent resistivity measured with RMT ranged from 16 to 28 Ω m−1. Highest apparent resistivities were observed at the stream bank as well as upslope the transect. Lowest values were observed at the ditch. Nevertheless, the ditch alone was not sufficient to explain the variations of the apparent resistivity observed here. Within fibric Fluviosols, apparent resistivity was almost constant. A continuous decrease in the apparent resistivity occurred within gleyic Fluvisols. Upslope the ditch, the apparent resistivity slightly increased within Albeluvisols (i.e., for thick BG and Ea horizons and afterwards, it greatly increased to the maximum of 26 Ω m). RMT apparent resistivity seemed to be better correlated with the total thickness of soil horizons than with the soil type itself. In addition, the results revealed that the shallower the bottom of CG1 and CG2 horizons, the lower the apparent resistivity estimated with the RMT. This may be linked to the depth of penetration of this method, which is about 7 m for a homogeneous half space with a resistivity equal to the mean apparent resistivity measured here. Consequently, most of the signal results from saprolites with low resistivity and not from topsoil materials with higher resistivity.
The apparent resistivity measured along the same profile with the ESQP was significantly higher than this evaluated with the RMT. This may be linked to a lower depth of penetration using ESQP than using RMT. This may explain the higher sensitivity of ESQP to the lateral variations of soils. ESQP values exhibited two distinct zones separated by the ditch. The first one showed low resistivities generally ranging between 40 and 90 Ω m. The lowest values correspond to the vicinity of the ditch. The second zone, located upslope the ditch, exhibited high resistivities that ranged between 80 and 120 Ω m. The apparent resistivities measured with ESQP seemed to discriminate well Fluvisols (either fibric and gleyic) from Albeluvisols. The time section for GPR is presented in Fig. 2C. A main reflection occurs at the interface between two adjoining materials (Fig. 2C) that probably present a pronounced contrast in dielectrical permittivity. The shape of this reflection is consistent with the bottom of the Fluviosols organic H horizon. This allows us in addition to estimate the speed for electromagnetic-wave propagation within the organic H horizon at about 6–7 cm ns−1. In the middle of the transect two vertical discontinuities arise, and seemed to correspond to the vertical faces of the ditch. Upslope, no significant reflectors were observed within loamy horizons. These results confirmed the bottom of organic horizons to be an excellent reflector of electromagnetic waves. This may be explained by the large amount of water hold by the organic matter [25]. Indeed this was confirmed by water contents, estimated by TDR, of about 80% in organic soils and 40% only in mineral horizons.
4 Conclusion
In this study of a bottomland in the Armorican Massif (western France), our main objective was to improve the continuous characterisation of soils affected by an excess of water by using a combination of geophysical techniques. Three geophysical techniques, the RMT, the GPR, and the ESQP, were implemented along eight transects where soils are extensively described. This study reveals that a complete characterisation of bottomland soil cover cannot be achieved using only one of these methods. The GPR and the ESQP allow the discrimination between thick organic horizons characterising the Fluvisols and loamy volumes characterising the Albeluvisols, as observed previously by Van Dam et al. [25] and Inman et al. [14]. Particularly, GPR allows us to delineate precisely the bottom of H organic horizons as well as anthropic features such as ditches. Such discrimination is not achieved by RMT, confirming previous work made in the same region by Mérot et al. [18] and Chaplot et al. [6]. Recent developments of this method, which may allow taking into account displacement currents in the bedrock and then to better characterise superficial materials [20] are promising. As a matter of fact, in this study, the RMT above all mainly characterises the soil material thickness, which is not a direct discriminant parameter between the soils.
This study shows that the implementation of geophysical multi-methods survey in bottomland areas may allow the continuous characterisation of soils' most important features. Such an implementation remains costly, but as it is non-invasive, it appears to be particularly suitable for the monitoring of an experimental site. From another point of view, the combination of geophysical methods allows the accurate interpolation and extrapolation of the still necessary direct observations of soils made at sparse locations, and consequently to optimise the outcome of such information.