Version française abrégée
1 Contexte géologique de l'étude
L'alignement volcanique de la Société résulte du mouvement de la plaque pacifique vers le nord-ouest au-dessus d'un hot-spot (Fig. 1). L'île de Tahiti, située à environ 50 km au nord-ouest de la zone active du point chaud, se compose de deux systèmes éruptifs coalescents (Tahiti-Nui et Tahiti-Iti), édifiés au cours des deux derniers millions d'années. L'évolution volcanologique de l'île principale (Tahiti-Nui) se caractérise par la concentration des magmas le long d'une zone de rift principale de direction est–ouest à N110 (Fig. 2). Cette discontinuité majeure est responsable de la déstabilisation des flancs nord et sud de l'île par de grands glissements de terrain, il y a environ 870 ka [18]. Dans la dépression créée par le glissement nord s'est rapidement reconstruit un second volcan bouclier. Les coulées ont rapidement débordé de la dépression nord, recouvrant les pentes préservées du volcan bouclier principal, et remplissant des vallées déjà creusées (Fig. 2). Finalement, la dépression sud [6] a été massivement comblée il y a environ 500 ka.

Location of Tahiti Island in the Society Archipelago. The bathymetric contours are shown at 1000-m intervals. The currently active hot-spot zone is indicated schematically by a circle. The arrow shows the movement of the Pacific plate.
Localisation de Tahiti dans l'archipel de la Société. Les contours bathymétriques sont représentés au pas de 1000 m. La zone active du point chaud est indiquée par un cercle. La flèche montre le mouvement de défilement de la plaque Pacifique.
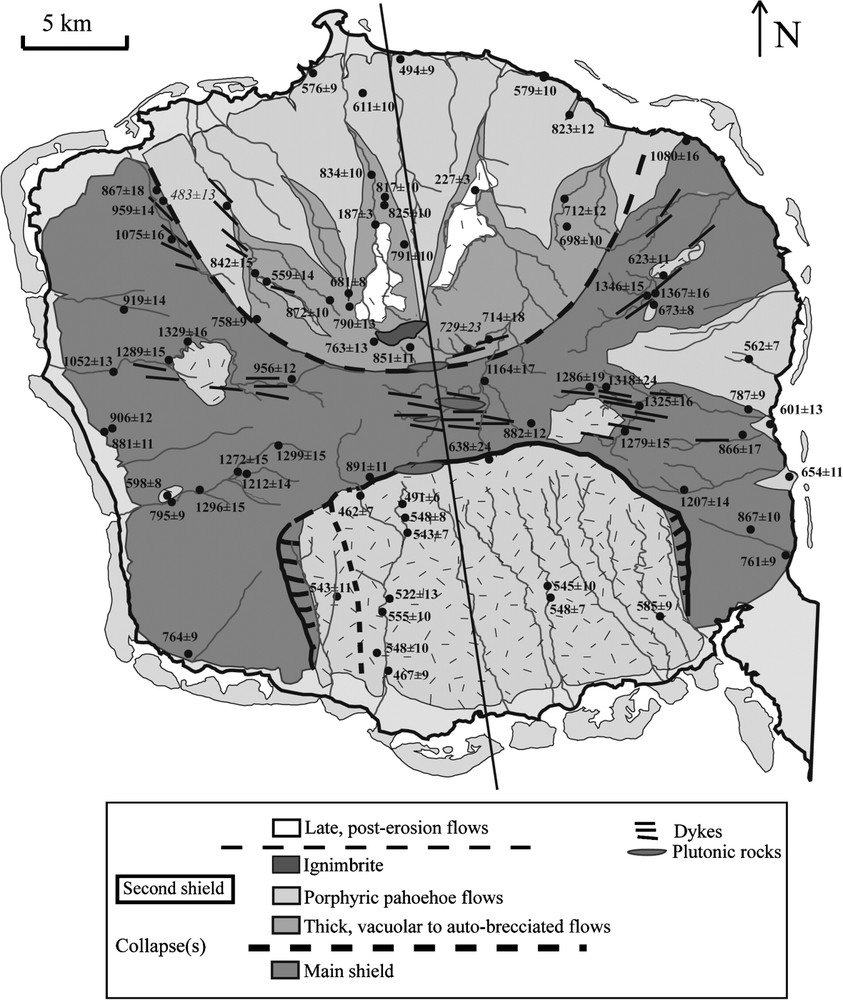
Simplified geological map of Tahiti-Nui. Previous ages and uncertainties [18] are reported in ka. Italic symbols for peculiar samples discussed in the present study, i.e. 81U and 81I. The solid line refers to the cross-section exposed in Fig. 4; after [18], modified.
Carte géologique simplifiée de Tahiti-Nui (d'après [18]). Âges et incertitudes sont indiqués en ka (symboles italiques pour les échantillons discutés dans le texte, i.e. 81U et 81I). La ligne noire indique le tracé de la coupe géologique exposée dans la Fig. 4.
2 Découverte d'une activité ignimbritique tardive
La fin de l'activité du second volcan bouclier est marquée par l'existence d'une épaisse formation volcanique de type ignimbrite constituant la partie supérieure du mont Orohena (Fig. 3), sommet actuel de l'île de Tahiti (2241 m). Les produits pyroclastiques associés, inatteignables dans la paroi, ont été échantillonnés au pied du massif, dans le fond de la haute vallée de la Vaitamanu (Fig. 4). Ces roches sont vésiculaires et différenciées. Elles contiennent des feldspaths alcalins, des amphiboles déstabilisées et des cristaux de néphéline précoce. Par ailleurs, de nombreuses billes de magma plus basique sont dispersées dans la mésostase évoluée de l'ignimbrite (Fig. 5), trahissant un mélange incomplet entre deux magmas. La minéralogie des billes est dominée par des phénocristaux d'augite dans une pâte vésiculaire riche en microlites de néphéline et d'amphiboles.

View toward south of the 200-m-thick pyroclastic formation constituting the upper part of the Orohena Massif.
Vue vers le sud sur l'épaisse formation pyroclastique constituant la partie supérieure du massif de l'Orohena.
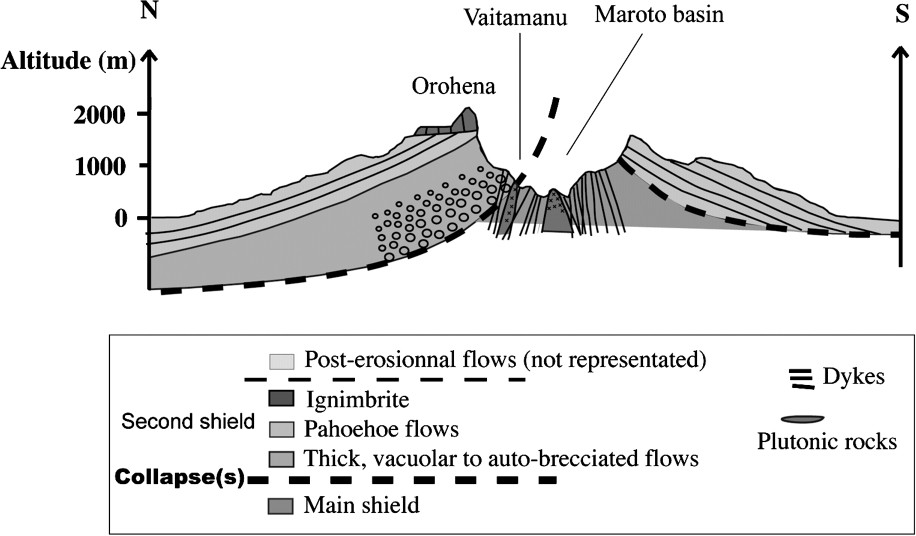
Schematic north–south cross-section showing the relations between the main volcano-structural units. Vertical exaggeration is 2×; after [18], modified.
Coupe nord–sud schématique, montrant les relations entre les principales unités volcano-structurales. L'exagération verticale est de 2. D'après [18], modifié.

(a) Photograph of ignimbritic products sampled in the east–west Vaitamanu valley. (b) Close-up of a centimetre-scale basic volcanic bleb. Note the surrounding zones of numerous smaller blebs within the more differentiated groundmass.
(a) Photographie des produits ignimbritiques échantillonnés dans la vallée est–ouest de la Vaitamanu. (b) Agrandissement d'une bille basique centimétrique. À noter les zones voisines riches en nombreuses billes plus petites, dispersées dans la mésostase différenciée de l'ignimbrite.
3 Datations K–Ar et géochimie sur roches totales
La datation des produits ignimbritiques différenciés a été réalisée sur mésostase volcanique et sur feldspaths alcalins selon la technique Cassignol–Gillot de la méthode K/Ar (Tableau 1). Ces déterminations, respectivement de et (Tableau 1), sont indissociables dans les marges d'incertitude . Un âge moyen de est ainsi obtenu pour l'ignimbrite, en bon accord avec sa position stratigraphique.
K/Ar dating results
Résultats de datation K/Ar
Sample | Phase | K (%) | 40Ar⁎ (%) | 40Ar⁎ (1011 at/g) | Age (Ma) | Uncertainty (Ma) |
81K | groundmass | 4.722 | 33.49 | 24.595 | 0.499 | 0.007 |
34.4 | 24.333 | 0.493 | 0.007 | |||
mean | 0.496 | 0.007 | ||||
81Kb | Alkali feldspars | 2.349 | 17.25 | 12.498 | 0.509 | 0.008 |
81U⁎ | groundmass | 1.547 | 6.86 | 7.644 | 0.473 | 0.012 |
6.59 | 7.962 | 0.493 | 0.013 | |||
mean | 0.483 | 0.013 |
⁎ Sample from [18].
Des analyses chimiques sur roche totale ont été effectuées au CRPG de Nancy (France) sur l'ignimbrite (81K), d'une part, et sur les billes de magma basique (81K2) préalablement extraites, d'autre part (Tableau 2). Les résultats montrent une importante différence de teneur en silice (Fig. 6). L'ignimbrite est une téphro-phonolite (SiO2 de 53,5%), tandis que les billes sont des basanites (SiO2 de 44%). Ce contraste de compositions explique très probablement le caractère incomplet du mélange, dû à l'importante différence de viscosité et de température entre les deux magmas impliqués.
Major elements geochemistry of the samples exposed in Fig. 7
Géochime des éléments majeurs pour les échantillons présentés sur la Fig. 7
Sample | SiO2 | Al2O3 | TiO2 | Fe2O3⁎ | MgO | CaO | Na2O | K2O | P2O5 | MnO | L.O.I | Total |
81K | 53.41 | 21.29 | 0.83 | 4.1 | 0.97 | 2.74 | 6.41 | 5.04 | 0.17 | 0.12 | 4.94 | 100.02 |
81K2 | 44.13 | 15.61 | 3.21 | 11.17 | 4.9 | 9.73 | 3.3 | 2.89 | 0.58 | 0.2 | 4.32 | 100.04 |
81I⁎ | 49.28 | 17.7 | 2.64 | 8.87 | 2.84 | 5.65 | 6.02 | 2.54 | 0.84 | 0.13 | 3.47 | 99.98 |
81L | 48.38 | 16.23 | 3.29 | 11.58 | 4.05 | 6.89 | 5.32 | 1.41 | 0.93 | 0.2 | 1.71 | 99.99 |
81U⁎ | 42.68 | 11.62 | 3.22 | 13.66 | 12.17 | 11.43 | 2.84 | 1.46 | 0.58 | 0.19 | 0.01 | 99.86 |
⁎ Data from [18].

Evolution in the silica content of the Tahiti-Nui lavas through time (modified from [18]). Black circles for lavas from the primitive shield; triangles for the post collapse second-shield activity; white squares for the late post-erosion activity. An ignimbrite sample (81K) as well as a volcanic bleb (81K2) are also shown (see Table 2).
Évolution des teneurs en silice dans les laves de Tahiti-Nui au cours du temps (d'après [18], modifié). Ronds noirs pour les laves du volcan bouclier principal ; triangles pour les laves du second volcan bouclier ; carrés noirs pour l'activité post-érosionelle tardive. Un échantillon de l'ignimbrite (81K) ainsi qu'une bille basique (81K2) sont également reportés (cf. Tableau 2).
4 Discussion
4.1 Comparaison avec d'autres données et implications pétrogénétiques
Dans un diagramme T.A.S. (Fig. 7), les produits ignimbritiques différenciés montrent des similitudes avec des syénites néphéliniques [2] affleurant actuellement dans le centre de l'île, du fait de l'intense dissection de la structure volcanique par l'érosion. L'éruption ignimbritique semble donc être liée à la vidange d'un réservoir magmatique superficiel actuellement partiellement exhumé.

Total alkalis-silica diagram for selected volcanic and plutonic rocks from Tahiti-Nui.
Diagramme T.A.S. pour quelques roches volcaniques et plutoniques de Tahiti-Nui.
4.2 Déclenchement de l'explosion pyroclastique
Deux mécanismes principaux sont susceptibles d'expliquer le lien entre l'immiscibilité magmatique observée et le déclenchement de l'éruption ignimbritique :
- (1) une injection tardive de magma basique dans le réservoir superficiel en cours de différenciation (ouverture du système par la base) ;
- (2) une sursaturation graduelle en fluides lors de la différenciation du réservoir par cristallisation fractionnée (système fermé).
L'éruption ignimbritique, unique dans l'histoire éruptive de Tahiti-Nui, marque la fin de la phase de construction majeure de l'île. Une activité basique a perduré pendant environ 50 ka avant de décliner, tandis que l'activité volcanique majeure liée au fonctionnement du point chaud de la Société s'est décalée vers le sud-est.
1 Introduction
Volcanic islands of hot-spot origin include differentiated magmatic stages within their late evolution. Hawaii (e.g., [24,26,31]), La Réunion [11,19,25] and Tenerife [9,14] are such examples. In this study, we describe for the first time a major differentiated ignimbritic eruption on Tahiti-Nui (French Polynesia). Specifically, we have dated products of this eruption and compared their chemical characteristics with those of plutonic rocks from the centre of the island [2,20,23]. The petrogenetic and vulcanological implications of this ignimbritic activity are discussed.
2 Geological framework
Tahiti belongs to the Society Archipelago (Fig. 1), which is one of the five linear volcanic chains of the south-central Pacific Ocean. Located at the southeastern end of this alignment, the island is composed of two eruptive complexes: Tahiti-Nui and Tahiti-Iti. Tahiti and its neighbour island Moorea constitute the higher and younger inactive islands, in agreement with the northwestward movement of the Pacific plate over a fixed hot-spot over the last millions years [3,7]. The currently active hot-spot zone is located around 50 km farther southeast (Fig. 1), as indicated by volcano-seismic crisis linked to the recent growth of several seamounts [1,29]. Although deeply eroded and subsided, Tahiti Island nevertheless is among the younger and best-preserved volcanic islands of the Archipelago, with a subaerial eruptive history encompassing the last two millions years (e.g., [8,16,18,22]).
The geology of Tahiti was recently reviewed ([18], Fig. 2). The volcano-structural evolution is characterized by the persistence of magma ascents through a main east–west rift zone [15], which was responsible for the lateral collapse of the northern and southern slopes of the main shield [6,15,18]. A main northern depression was thus created, in which grew a second shield. The flows rapidly overflowed the depression, capping the preserved slopes of the pre-collapse main shield, and filling existing valleys. The flows then gradually reached the southern part of the island and filled the southern landslide depression. Finally, a late post-erosion effusive activity occurred at around 200 ka [18].
3 Evidence for a late differentiated ignimbritic activity
The end of the second shield building-stage of Tahiti-Nui is marked by the existence of a massive ignimbrite-like formation [16,17] exposed as a 200-m-thick unit in the upper part of the Orohena massif (Fig. 3). This unit cannot be directly reached in the field, since the Orohena massif is cut by 1000-m-high cliffs, which is a consequence of the intense dissection of the volcanic structure by erosion. However, the apparent preserved geometry of the ignimbritic unit shows a direction of pyroclastic flow to the northeast. Moreover, the great thickness of this formation most probably resulted from the infilling of a pre-existing valley, suggesting that the explosive eruption possibly occurred after a volcanic quiescence in the ultimate stage of the second shield-building activity (Fig. 4).
Related pyroclastic products have been sampled as blocks in the Vaitamanu Valley, which is incised just to the south of the Orohena massif (Fig. 4), at the inferred limit between the two nested shields [15,18]. At the base of the cliff, in the river, lie large prismatic blocks fallen from the columnar-jointed ignimbritic formation 1000 m above. These volcanic rocks are vesicular (Fig. 5a) and differentiated, as marked by the occurrence of alkali feldspars, amphiboles, clinopyroxenes and sphene. In addition, the presence of abundant nepheline crystals indicates a high alkaline character associated to relatively low silica content. Most of the nephelines are dispersed in the groundmass, but some of them are included in the feldspars, indicating relatively early crystallization.
The Orohena ignimbritic products have another striking feature. They typically show thin zones of basic magma blebs within the more evolved host liquid (Fig. 5b), indicative of an incomplete mixing process. In thin section, the contact between the two magmas is quite diffuse, although a little fringe of oxidation exists. The mineralogy of the blebs is dominated by augite phenocrysts, whereas its vesicular vitrified groundmass contains a few microcrystals of nephelines and amphiboles.
4 K/Ar dating and whole-rock chemical analysis
Concentrates of the main differentiated groundmass (81K) and 250–500-μm-sized cogenetic alkali feldspars phenocrysts (81Kb) were obtained by use of heavy liquids and magnetic separation for K/Ar dating. The analytical procedure for K/Ar analysis is described elsewhere [4,12]. Even though the differentiated groundmass may be more contaminated by atmosphere than the feldspars, its higher K-content (Table 1) is suitable to date accurately the ignimbritic explosion.
The ages of and (Table 1) obtained respectively on the groundmass and the feldspars are undistinguishable within the uncertainties at the 1-σ level. The K/Ar determinations thus yield a mean age of for the ignimbritic eruption.
Our data are, moreover, consistent with other radiometric data (e.g., [5,8,18]). In addition to the K/Ar determinations, a bleb (81K2) and the host liquid (81K) were sent to the CRPG of Nancy for geo-chemical ICP-MS analyses. These new results were added to data of Hildenbrand et al. [18] showing the evolution of the silica content in lava through time (Fig. 6). Besides the general decrease in SiO2 with time, one can observe a clear contrast in composition between the strongly undersaturated blebs and the evolved host liquid. This compositional gap may explain the incomplete character of the magma mixing because of a strong difference in viscosity and temperature between the two liquids involved.
5 Discussion
5.1 Comparison with other data and petrogenetic implications
The analyses for the ignimbrite and the blebs (Table 2) are plotted on a total alkalis–silica diagram (Fig. 7), in which analyses of a differentiated dyke (81I, [18]) and a plutonic rock (81L) found in the same Vaitamanu Valley are also shown. A late volcanic flow from the upper part of the second shield-building activity (sample 81U, dated at , [18]) is also plotted in Fig. 7, along with some plutonic rocks from the centre of the island [2,23] as well as the differentiated (volcanic?) rock analysed by Cheng et al. [5].
All these data plot into the strongly under-saturated alkaline series (Fig. 7). The blebs 81K2 are basanites, whereas the plutonic rock 81L and the dyke 81I are slightly more evolved. However, the ignimbrite is tephri-phonolitic in composition. Although containing phenocrystic nephelines, it has a silica content intermediate between Ne-syenites and syenites [2], whereas the alkali contents are similar. This may be explained by limited mixing between the injection of basic magma and the differentiated component. The former differentiated magma originally probably had a greater Na2O + K2O content, likely indicating an initial composition closer to the phonolitic field. Therefore, the Orohena ignimbritic products show similarities with Ne-syenites plutonic rocks exposed in the Maroto Basin. The ignimbrite thus likely comes directly from a shallow reservoir, the products of which outcrop to the south in the core of the Maroto basin, subsequently deeply dissected by erosion [16].
5.2 Triggering of the pyroclastic explosion
Two possible processes may be invoked to explain the link between the observed magma partial immiscibility and the triggering of the violent pyroclastic eruption:
- (1) a late re-injection of basic magma into the shallow differentiated reservoir (e.g., [9,10,27]);
- (2) a gradual fluid oversaturation linked to crystallization processes in a closed magma chamber [28]. The ascension of bubbles from a more mafic basal layer would then be responsible for the development of basic inclusions larger than 1 cm [30].
On Tahiti-Nui, the first hypothesis seems the more plausible, because basic effusive activity occurred synchronously with, or slightly after, the explosion [18]. Some of the basic lavas were erupted along the lateral rims of the northern collapse, as revealed by the presence of late intrusions defining a secondary rift zone [16,18]. Thus some of the ascending magmas were erupted through the major lateral discontinuity between the two nested shields, while a small fraction may have penetrated into the central reservoir, triggering the ignimbritic explosion. A similar process has been proposed for the neighbour island of Moorea [21] to account the lateral extrusion of late basic lavas together with magma mixing within an inferred magma reservoir. However, no associated pyroclastic deposits have been described at Moorea. In the case of Tahiti-Nui, the late and hot re-injection of basic magma enhanced here may have provoked the exsolution of fluids dissolved in the differentiated liquid to trigger the violent ignimbritic explosion, while a residual part of the differentiated magma remained in the reservoir and cooled slowly and gently.
The overpressure accumulated before explosion can be broadly estimated taking into account the pre-erosion geometry of the second shield. Assuming a formerly conical shape, a maximum summit altitude around 3000 m is obtained from extrapolation of the northern and southern slopes [18]. This elevation is comparable to the one of similar fill-in edifices from other hot-spot oceanic mature volcanic complexes, e.g., the ‘Piton de la Fournaise’ in the Réunion Island [13]. The inferred altitude involves an overlying volcanic pile of about 2500 m over the shallow reservoir. Assuming lithostatic purely pressure (mean density of 3), the critical overpressure responsible for the ignimbritic explosion can thus be estimated to be slightly less than 0.75 kbar.
Following the explosion, basic activity continued for about 50 kyr and then rapidly waned [18]. The late injection of mafic magma thus marks the end of the main shield-building phase on Tahiti-Nui, which also coincides in time with the shift of the main volcanic activity to the southeast [22].
6 Conclusions
The final shield-building stage of Tahiti-Nui is characterized by an ignimbritic episode marked by the presence of a thick pyroclastic unit in the upper part of the Orohena Massif. Related tephri-phonolitic products dated at a mean age of by the K/Ar Cassignol technique have chemical affinities with plutonic rocks exposed to the south in the central Maroto Basin. This indicates that the Orohena evolved as a separate edifice fed by a shallow reservoir, now exhumed by erosion to the south. The presence of basic magma blebs in the differentiated groundmass records an incomplete magma mixing process linked to a late injection of basic magma into the shallow reservoir. This injection may have induced the sudden exsolution of fluids dissolved in the evolved liquid, producing a maximum estimated overpressure of about 1 kbar and triggering the violent explosion. Also, some of the injected basic magmas have been directly erupted along the lateral rims of the main U-shape depression created by the previous collapse of the northern flank of the primitive shield-volcano.
After the explosion, basic activity rapidly waned, while the main volcanic activity shifted to the present active zone of the Society plume.
Acknowledgements
We are grateful to K. Maamaatuaiahutapu (University of French Polynesia) and D. Reymond (LDG/CEA) for their help on the field. Constructive suggestions from R.I. Tilling and J. McPhie were greatly appreciated. This is LGMT contribution 46.