Abridged English version
As many regions of the Mediterranean basin, the Languedoc-Roussillon is characterised by important karstic formations, which often represent the main groundwater resources for the local population. More than 600 karstic springs are known in these formations [17]. About 3.5% of these springs exhibit chloride contents higher than the French drinking-water quality standard ([Cl] = 250 mg l−1). According to Passavy [16] and Puradimaja [17], these waters could have two main origins, dissolution of evaporites or seawater intrusion for coastal karsts. However, the recent works of Aquilina et al. [1,2] showed the complexity of some of these saline karstic systems, whose waters could correspond to a three end-members mixing (both those cited above plus a geothermal one).
Many authors have worked worldwide on the identification of the origin of saline groundwaters in various hydrogeological environments, karstic or not [9,13,14,19–21]. Chloride (Cl), sulphates (SO4), bromide (Br), boron (B), and lithium (Li) are associated with saline environments and are often used to determine the origin of the groundwater salinity. Moreover, owing to the behaviour of Li and SO4 in hydrogeologic systems with potential evaporitic or deep-fluid end-members, the Li/SO4 ratio seems to be an interesting tool for the study of such systems. On the one hand, there is a Li enrichment of seawater with respect to the precipitated phases during seawater evaporation [10] (Fig. 1), and on the other hand, water–rock interactions at high temperatures favour Li mobility [4]. By considering Cl, SO4, Br, B, Li concentrations and the Li/SO4 ratio, the aim of the present study is to extend the works of Aquilina et al. [1,2] to the various saline karstic systems of Languedoc-Roussillon, in order to distinguish the different origin of the waters.
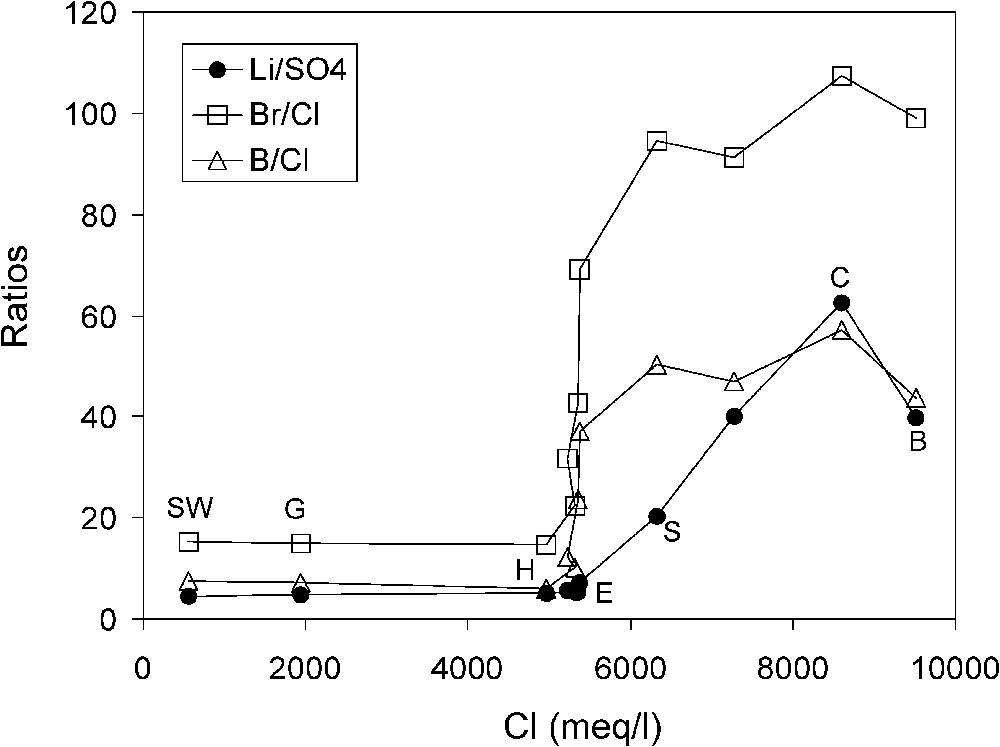
Évolution des rapports Li/SO4, Br/Cl et B/Cl (en 10−4 meq l−1/meq l−1) en fonction de la concentration en Cl (meq l−1) au cours de l'évaporation de l'eau de mer (SW), et précipitation des principaux sels (G : gypse ; H : halite ; E : epsomite ; S : sylvite ; C : carnallite ; B : bischofite). D'après Fontes et Matray [10].
Li/SO4, Br/Cl, and B/Cl ratios (in 10−4 meq l−1/meq l−1) evolution versus Cl (meq l−1) during seawater (SW) evaporation, and precipitation of the main salts (G: gypsum; H: halite; E: epsomite; S: sylvite; C: carnallite; B: bischofite). From Fontes and Matray [10].
The karstic formations of Languedoc-Roussillon are essentially made of Jurassic and Cretaceous deposits. Triassic evaporite formations often lie below these karsts. Many large tectonic faults are present in the region and have been active during each important tectonic phase. Chloride-rich karstic waters are observed over four areas in the Languedoc-Roussillon region (Fig. 2): (i) ‘Étang de Thau’, (ii) ‘Corbières orientales’, (iii) ‘Corbières centrales’, and (iv) ‘Garrigue de Montpellier’.
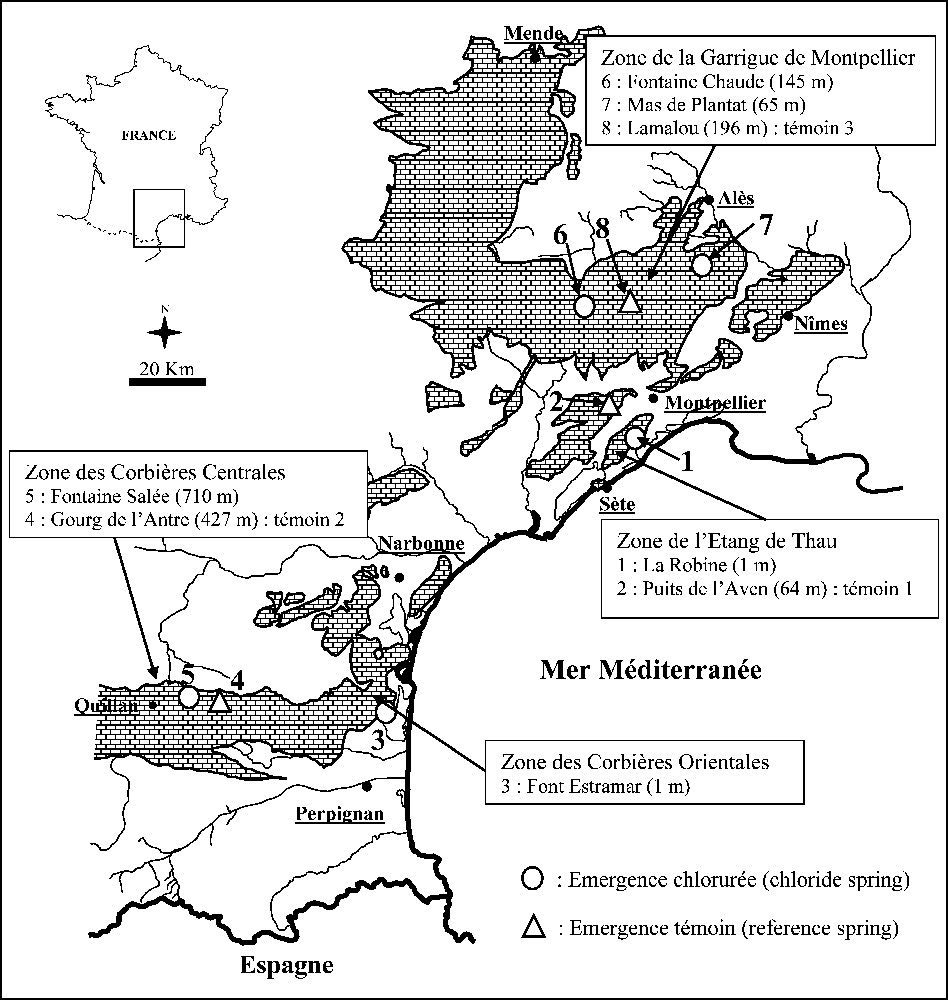
Principaux massifs karstiques crétacés et jurassiques du Languedoc-Roussillon, et localisation des huit émergences karstiques échantillonnées. Entre parenthèses sont indiquées les altitudes des émergences.
Principal Cretaceous and Jurassic karstic formations of the Languedoc-Roussillon region, and localisation of the eight sampled karstic springs. The spring elevation is between brackets.
Five characteristic chloride-rich karstic springs and three normal karstic springs representative of the region (named ‘reference’) have been sampled over the four surveyed areas (Fig. 2). Water samples have been collected over three days in April 2005, during high water levels. Temperature, pH and electrical conductivity were measured in the field. Major and trace elements have been determined at the HydroSciences laboratory (University of Montpellier-2) and at the laboratory of Hydrogeology of the University of Avignon. The data are presented in Table 1.
Paramètres physico-chimiques, concentrations en éléments majeurs et traces, rapports Br/Cl (en 10−4 meq l−1/meq l−1) et Li/SO4 (en 10−4 meq l−1/meq l−1) des huit émergences karstiques échantillonnées en avril 2005
Physicochemical parameters, major and trace element's concentrations, Br/Cl and Li/SO4 ratios (in 10−4 meq l−1/meq l−1) for the eight karstic springs sampled in April 2005
Physico-chimie | Éléments majeurs | Éléments traces | Rapports | ||||||||
(°C) | (μS cm−1) | % | (mg l−1) | (μg l−1) | 10−4 (meq l−1/meq l−1) | ||||||
Emergence | pH | T | CE | pCO2 | Cl | SO4 | Br | B | Li | Br/Cl | Li/SO4 |
Robine | 6,6 | 17,4 | 7220 | 13,9 | 1955,3 | 266,6 | 6644 | 269,5 | 31,6 | 15,1 | 8,2 |
Font Estramar | 7,4 | 18,5 | 8050 | 0,8 | 2411,2 | 383,0 | 8026 | 574,5 | 33,3 | 14,8 | 6,0 |
Fontaine Salée | 7,5 | 9,0 | 20 400 | 0,3 | 5433,7 | 2088,4 | 1056 | 187,8 | 42,4 | 0,9 | 1,4 |
Fontaine Chaude | 7,2 | 18,6 | 1357 | 2,3 | 187,1 | 112,0 | 135 | 51,7 | 28,7 | 3,2 | 17,8 |
Mas de Plantat | 7,0 | 20,8 | 1421 | 3,6 | 235,7 | 59,3 | 197 | 51,3 | 30,1 | 3,7 | 35,2 |
Témoin 1 | 7,7 | 12,9 | 693 | 0,3 | 11,5 | 7,3 | 22 | 10,8 | 0,3 | 8,5 | 2,6 |
Témoin 2 | 7,3 | 12,3 | 514 | 1,1 | 8,8 | 13,2 | 23 | 6,6 | 1,9 | 11,6 | 10,2 |
Témoin 3 | 7,2 | 13,5 | 596 | 1,9 | 8,4 | 7,5 | 30 | 8,4 | 0,3 | 15,8 | 2,3 |
Mer [5] | – | – | – | – | 19 354 | 2712 | 67 300 | 4500 | 173,5 | 15,4 | 4,4 |
The coastal springs of the Robine and Font Estramar exhibit similarities. Their SO4/Cl and Br/Cl ratios show that they both result from the mixing of karstic and sea waters (Table 1 and Fig. 3a and b) and confirm previous results obtained on this karstic system [1,2,16,17]. The seawater proportion, calculated from these ratios, is respectively 10% for the Robine and 13% for Font Estramar. Their respective contents in Cl, SO4 and Br indicate the absence of an evaporitic end-member. Br/Cl ratio is of 15.1 × 10−4 (meq l−1/meq l−1) for the Robine and of for Font Estramar, very close to the seawater ratio (). However, for the Robine spring, the B content indicates a different origin (Fig. 3c) and confirms the findings of Aquilina et al. [1]. These authors suggest a possible evaporitic end-member in the waters of the ‘Étang de Thau’ coastal system. This evaporitic end-member is however minor in the physicochemistry of the Robine system. The Li/SO4 ratio for the two springs is also quite similar and is slightly higher than the seawater ratio (). For the Robine, the Li/SO4 ratio is coherent with a deep-component of the water as shown by Aquilina et al. [1,2]. For Font Estramar, the Li/SO4 seems to confirm the possible deep-fluid contribution through the faults in this system, suggested by the high temperature of the water.
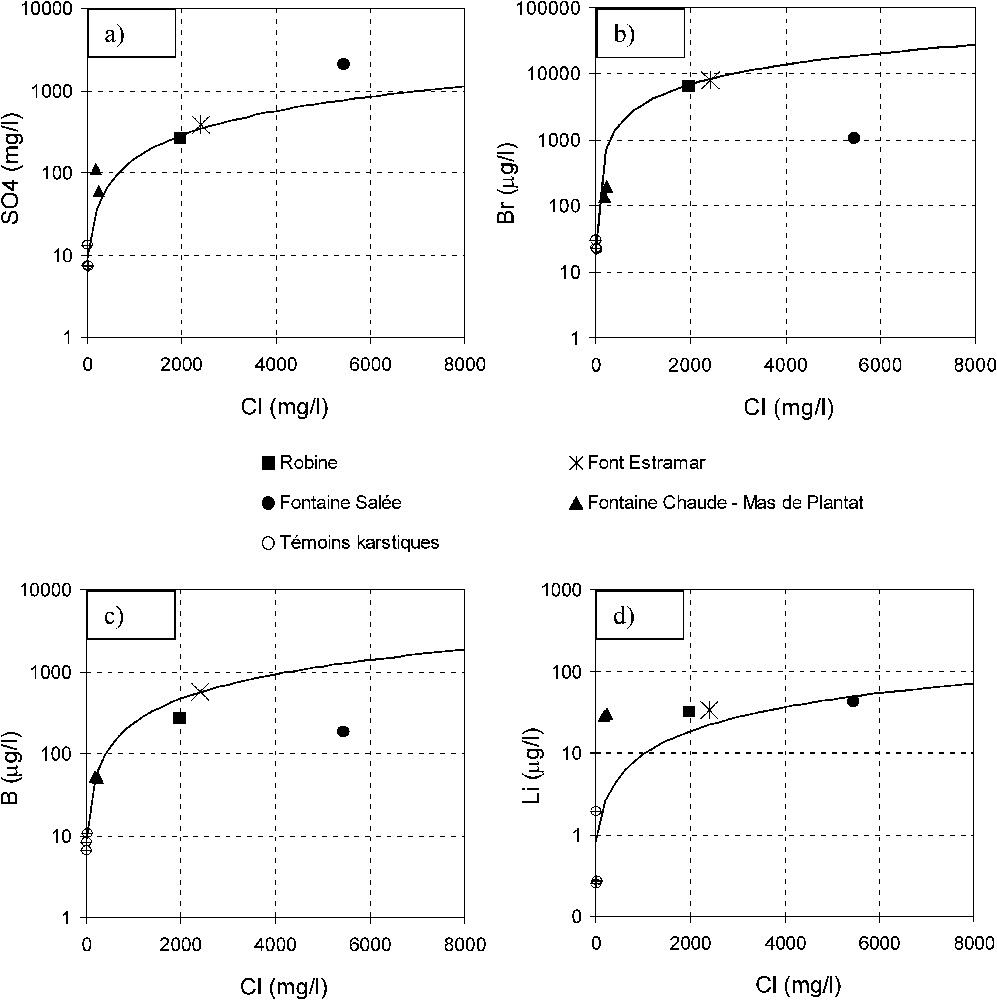
Pour les huit émergences échantillonnées : (a) relations entre SO4 et Cl, en mg l−1 ; (b) relations entre Br en μg l−1 et Cl en mg l−1 ; (c) relations entre B en μg l−1, et Cl en mg l−1 ; (d) relations entre Li en μg l−1, et Cl en mg l−1. La courbe en trait plein correspond à la courbe de dilution entre l'eau de mer et les émergences témoins. Le pôle marin correspond aux valeurs des concentrations de l'eau de mer actuelle [5].
For the eight sampled springs: (a) SO4 versus Cl, in mg l−1; (b) Br in μg l−1 versus Cl in mg l−1; (c) B in μg l−1 versus Cl in mg l−1; (d) Li in μg l−1 versus Cl in mg l−1. The solid curve corresponds to the seawater–‘reference’ springs dilution line. The seawater end-member values are from [5].
Waters of the three continental chloride-rich springs (‘Fontaine Salée’, ‘Fontaine Chaude’ and ‘Mas de Plantat’) are characteristic of evaporite dissolution (Fig. 3a and b), as shown by Passavy [16] and Puradimaja [17] from major elements and sulphur isotopes studies. Their Br/Cl ratios are very low (Table 1), and are largely lower than that of seawater. This evaporitic end-member is also well defined by the B content of these springs (Fig. 3c). However, their Li/SO4 ratios are very different (Table 1). The very low ratio of the ‘Fontaine Salée’ is in agreement with the dominant evaporitic end-member and suggests no contribution from deep-fluids. The high ratios obtained for the ‘Fontaine Chaude’ and for the ‘Mas de Plantat’ indicate an important enrichment in Li provided mainly by deep-fluids. This enrichment is consistent with the high temperature of these springs and with their location near major regional faults [15]. In the case of mixing between geothermal water and water marked by evaporites dissolution, the Li mobilized in the high temperatures water–rock interactions allows us to distinguish the geothermal contribution from the evaporitic one, previously inferred from SO4, Br and B.
The coastal situation of the Languedoc-Roussillon area and its complicated geological context explain the different possible water origins for the chloride-rich karstic springs. The combined study of Cl, SO4, Br, B, Li, which are the most often used major and trace elements in saline environments, made it possible to distinguish the participation of several end-members in these saline waters. Results obtained with Br and B confirm the interest to use these two elements for tracing such water types. Associated with these relevant tracers, the Li/SO4 ratio appeared as a complementary tool for the determination of water origins in systems with deep-fluids and/or with evaporite dissolution. These preliminary results have to be confirmed through the use of other tracers such as 36Cl, in order to better understand these complex systems involving deep circulations and marine intrusion in relation with the aquifer geometry. This work is linked with the emerging problem of the management of karstic water resources in the Mediterranean basin.
1 Introduction
Comme de nombreuses régions du Bassin méditerranéen, le Languedoc-Roussillon est largement occupé par des terrains carbonatés qui constituent généralement des réservoirs karstiques de première importance en termes de ressources en eau douce. L'histoire structurale et paléo-géographique régionale explique le morcellement des plates-formes carbonatées et la diversité des situations hydrogéologiques. Plus de 600 émergences karstiques y ont été recensées [17]. Pour environ 3,5% d'entre elles, la concentration en chlorures dépasse la norme française de potabilité qui est de 250 mg l−1. Selon Passavy [16] et Puradimaja [17], ces eaux peuvent avoir deux origines dominantes : (1) une origine marine (karsts côtiers), ou (2) un marquage évaporitique. Toutefois, des travaux récents [1,2] ont montré la complexité des systèmes karstiques salins, dont les eaux peuvent notamment correspondre à des mélanges impliquant trois types de signatures chlorurées : les deux signatures évoquées ci-dessus (marine et évaporitique), plus une troisième signature d'origine profonde correspondant à des fluides provenant du socle.
De nombreux travaux ont porté sur la détermination de l'origine des eaux chlorurées souterraines dans différents environnements, karstiques ou non karstiques [9,13,14,19–21]. Dans ces études, deux éléments majeurs et trois éléments traces sont fréquemment dosés, les chlorures (Cl), les sulfates (SO4), le brome (Br), le bore (B), et le lithium (Li), car ils sont présents en quantités importantes dans les environnements favorables à la salinisation des eaux douces (c'est-à-dire eau de mer, évaporites et eaux géothermales). En raison, d'une part, des processus de partage entre le lithium et les sulfates lors de l'évaporation de l'eau de mer [10] et, d'autre part, de la mobilisation du Li lors des contacts eau–roche à hautes températures [4], le rapport Li/SO4 semble être un outil intéressant dans la discrimination d'eaux évaporitiques et géothermales. Les objectifs de la présente étude sont d'étendre les travaux d'Aquilina et al. [1,2] aux principaux systèmes karstiques salins du Languedoc-Roussillon en s'appuyant sur les concentrations en Cl, SO4, Br, B, et Li, et sur le rapport Li/SO4 en particulier, afin de mettre en évidence les différents pôles des eaux chlorurées, notamment la contribution de fluides profonds en provenance du socle.
2 Le rapport Li/SO4
Le rapport Li/SO4 semble pouvoir être utilisé pour distinguer l'origine profonde ou évaporitique des eaux. En effet, au cours de l'évaporation de l'eau de mer, jusqu'au stade de la bischofite, l'eau de mer s'enrichit en Li au détriment des phases précipitées qui s'appauvrissent en Li [10] (Fig. 1). Dans le cas de la dissolution d'évaporites qui n'ont pas atteint ce stade, ce rapport est donc plus faible que celui de l'eau de mer, qui est de 4,4 × 10−4 (meq l−1/meq l−1).
À l'inverse, les interactions eau–roche à haute température favorisant la mobilité du lithium [4], on peut s'attendre à un rapport Li/SO4 supérieur à celui de l'eau de mer dans le cas d'une origine géothermale de l'eau. La concentration en Li est étroitement liée au temps de contact eau–roche et Li est souvent utilisé comme géothermomètre [7]. Néanmoins, le comportement des sulfates d'origine profonde est plus difficile à appréhender, car leur origine est souvent complexe [11,12]. Le rapport Li/SO4 ne doit donc pas être utilisé seul, mais doit être couplé avec d'autres traceurs tels que le brome ou le bore, afin de croiser les informations fournies par ces différents traceurs.
3 Cadre géologique
Au Mésozoïque, après une phase de dépôts continentaux et évaporitiques au Trias, une sédimentation marine s'est progressivement installée et s'est poursuivie durant tout le Jurassique et jusqu'au Crétacé. Les principaux aquifères karstiques de la région Languedoc-Roussillon se trouvent dans ces formations jurassiques à crétacées. Dans l'ensemble de ces massifs karstiques, des émergences fortement chlorurées ont été observées sur quatre grandes zones, que nous décrivons succinctement ci-dessous (Fig. 2).
La première zone, que nous avons nommée « Étang de Thau », est une zone littorale, constituée par les massifs karstiques jurassiques situés sur le pourtour de l'Étang de Thau. Elle se situe entre la faille de Nîmes, au sud, et le chevauchement du pli de Montpellier, au Nord.
La deuxième zone, également littorale, dite des Corbières orientales, s'organise sur le pourtour des étangs littoraux situés entre Perpignan et Narbonne. Les formations sont de nature jurassique et crétacée.
La zone des Corbières centrales correspond à un ensemble de formations essentiellement composées de grès et de calcaires crétacés, récifaux et deltaïques, ainsi que d'argiles salifères triasiques.
La quatrième et dernière zone, celle de la garrigue de Montpellier, est comprise entre la faille des Cévennes, au nord, et le pli de Montpellier, au sud. Les formations sont essentiellement composées de calcaires et de marno-calcaires, jurassiques et crétacés. Localement, la semelle triasique peut affleurer.
4 Prélèvements et analyses
Une campagne de prélèvements d'échantillons d'eau a été effectuée sur trois jours en avril 2005. Les différentes émergences se trouvaient à leur régime hydrologique moyen. L'échantillonnage a porté, d'une part, sur un nombre restreint d'émergences représentatives de celles présentant des eaux chlorurées pour chacune des quatre zones et, d'autre part, sur un nombre restreint d'émergences représentatives des émergences non chlorurées des massifs karstiques de la région (Fig. 2).
Le pH, la température et la conductivité électrique ont été mesurés in situ. La pression partielle de CO2 équilibrante (pCO2) a été calculée à partir des équilibres calco-carboniques, à l'aide du pH et de l'alcalinité mesurés. Les anions et les cations majeurs ont été dosés par chromatographie ionique, au laboratoire HydroSciences de Montpellier. Les éléments traces ont été déterminés par ICP-MS (service commun de l'Isteem, université Montpellier-2), excepté le brome (bromures), dosé au laboratoire d'hydrogéologie d'Avignon par chromatographie ionique. Les résultats de l'ensemble des analyses sont reportés dans le Tableau 1.
5 Résultats – discussion
5.1 Zone de l'étang de Thau : exemple de la source littorale de La Robine
La concentration en chlorures relevée sur la source de la Robine (1955,3 mg l−1) est environ 180 fois supérieure à celle des eaux karstiques de la région. Les rapports SO4/Cl et Br/Cl (Tableau 1 et Fig. 3a et b) placent cette source sur la courbe de dilution de l'eau de mer. La proportion d'eau de mer, calculée à partir des chlorures, des sulfates ou des bromures, est de l'ordre de 10%.
Le rapport Br/Cl est souvent utilisé pour discriminer une eau ayant été en contact avec des évaporites, d'une eau correspondant à un mélange avec de l'eau de mer [3,6,8,9,13,19]. Dans le premier cas, ce rapport est toujours inférieur à celui de l'eau de mer, qui est de . Le rapport Br/Cl relevé sur la Robine () indique l'absence de contacts avec des niveaux évaporitiques. En revanche, le bore, qui est également un traceur pertinent des environnements évaporitiques [18,19], présente une anomalie (Fig. 3c), ce qui va dans le sens de la présence d'un pôle évaporitique et conforterait les résultats d'Aquilina et al. [1], selon qui un pôle évaporitique semble probable.
La contribution géothermale en provenance du socle a déjà été largement mise en évidence dans ce système [1,2]. La température relevée sur la source de la Robine atteste cette contribution profonde. De même, la valeur élevée de pCO2 (13,9%) suggère un transfert profond de ce gaz (dissous ou libre). Dès lors, nous avons considéré le rapport Li/SO4 afin de vérifier son lien avec les apports géothermaux en provenance du socle. Le rapport Li/SO4 () est supérieur à celui de l'eau de mer. En considérant les résultats précédents obtenus à partir des sulfates et des bromures, n'impliquant pas un pôle évaporitique, le lithium semble plutôt provenir des échanges eau–roche à hautes températures qui ont lieu au niveau du socle. Si un pôle évaporitique est présent comme le suggérerait la concentration en bore, au regard des sulfates, des bromures et du lithium, ce pôle n'est en revanche pas prépondérant dans la chimie des eaux de cette émergence et vraisemblablement de l'ensemble du système karstique.
5.2 Zone des Corbières orientales : exemple de la source littorale de Font Estramar
Avec 2411,2 mg l−1, la concentration en chlorures de cette émergence littorale dépasse celle de la Robine. Sur la Fig. 3a et b, la source de Font Estramar se place sur la courbe de dilution de l'eau de mer. La proportion calculée de l'eau de mer à partir des chlorures, des sulfates et des bromures est approximativement de 13%. Le rapport Br/Cl, qui est de , ainsi que l'absence d'anomalie en bore (Fig. 3c), semblent exclure tout contact significatif avec des formations évaporitiques.
En revanche, la température de l'eau de la source de Font Estramar est supérieure d'environ 5 °C à la température moyenne des eaux karstiques de la région et soulève l'hypothèse de probables remontées d'eau profonde via les accidents tectoniques qui affectent ce massif. Cette hypothèse est confirmée par une anomalie positive en lithium (Fig. 3d), ainsi que par le rapport Li/SO4, qui est de (), supérieur à celui de l'eau de mer. Sachant que les concentrations en chlorures, sulfates, bromures et bore semblent écarter un éventuel pôle évaporitique, dès lors, l'anomalie en lithium traduirait des apports profonds en provenance du socle.
5.3 Zone des Corbières centrales : exemple de la fontaine Salée
La concentration en chlorures de la fontaine Salée est très élevée (5433,7 mg l−1). Les concentrations en sulfates, bromures et bore (Fig. 3a, b et c) montrent la forte influence évaporitique de cette émergence, déjà mise en évidence par Passavy [16] et Puradimaja [17] à partir des éléments majeurs et des isotopes du soufre. Le rapport Br/Cl est relativement faible () et est très inférieur à celui caractéristique de l'eau de mer. Il se démarque très nettement de ceux relevés sur les sources littorales de la Robine et de Font Estramar. Cette nette signature évaporitique par les bromures et le bore, ainsi que la concentration très élevée en chlorures, témoignent de contacts importants entre l'eau de l'aquifère crétacé et le Trias salifère, qui joue localement le rôle de mur de l'aquifère.
Au regard de l'altitude de cette émergence (710 m), sa température ne présente aucune anomalie. Le rapport Li/SO4 est très faible () et inférieur à celui de l'eau de mer ; le plus faible de ceux de la série de sources échantillonnées dans cette étude. Compte tenu des précédents résultats, ce très faible rapport semble bien, d'une part, attester la dissolution de minéraux évaporitiques et, d'autre part, exclure des apports en lithium dus à d'éventuelles interactions eau–roche à hautes températures.
5.4 La zone de la garrigue de Montpellier : exemples des sources de la fontaine Chaude et du mas de Plantat
Ces deux émergences ont des comportements très proches, à la fois d'un point de vue physique et chimique. Leurs concentrations en chlorures sont respectivement de 187,1 mg l−1 et de 235,7 mg l−1.
Sur la Fig. 3a et b, ces deux émergences sont situées au-dessus de la droite de dilution de l'eau de mer. Les teneurs en Br et en B indiquent une forte influence évaporitique (Fig. 3b et c), comme cela avait démontré par Passavy [16] et Puradimaja [17] à partir des éléments majeurs et des isotopes du soufre. Les rapports Br/Cl sont respectivement pour la fontaine Chaude et la source du mas de Plantat de 3,2 et et sont donc bien inférieurs au rapport de l'eau de mer. Ainsi, les caractéristiques en chlorures, sulfates, bromures et bore sont typiques d'eaux ayant été en contact avec des terrains évaporitiques.
Les températures respectives de ces deux émergences, qui sont de 18,6 et de 20,8 °C suggèrent la contribution d'un pôle géothermal. Ces deux sources sont marquées par une importante anomalie en lithium (Fig. 3d). Leurs rapports Li/SO4 respectifs sont très élevés, 17,8 pour la fontaine Chaude et pour la source du mas de Plantat. Ces deux sources se distinguent très nettement de la fontaine Salée, autre source étudiée à signature évaporitique, qui présente le rapport Li/SO4 le plus faible. Toutefois, ces deux émergences sont situées dans des massifs jurassiques très fracturés, avec de nombreuses failles. La fontaine Chaude se situe notamment à proximité de la faille des Cévennes, accident tectonique régional majeur [15]. C'est donc à la faveur de ces accidents tectoniques majeurs que l'ascension d'eau profonde peut se faire, tout en se chargeant en sels en traversant le Trias salifère. Par conséquent, dans le cas d'un mélange d'eau d'origines évaporitique et géothermale, le lithium mobilisé lors des contacts eau–roche à hautes températures permet de distinguer la contribution géothermale de la contribution évaporitique, qui a pu être mise en évidence au préalable avec les sulfates, les bromures et le bore.
6 Conclusion
L'origine variée des eaux des émergences karstiques chlorurées du Languedoc-Roussillon s'explique par une situation géographique globalement littorale et par un contexte géologique complexe. L'approche hydrochimique menée, ciblée sur quelques sources karstiques chlorurées caractéristiques du Languedoc-Roussillon, a permis de mettre en évidence la contribution de plusieurs pôles dans les eaux chlorurées de certains de ces aquifères. Cette étude a permis de compléter les anciens travaux [16,17] qui avaient permis de mettre en évidence le pôle dominant de ces eaux (évaporitique ou marin), et a permis de mettre en évidence l'importance des circulations profondes dans les aquifères karstiques. Ces pôles sont en lien étroit avec la structure, la localisation et l'histoire de ces massifs karstiques. Les résultats obtenus sur les bromures et le bore confirment l'intérêt de l'utilisation de ces deux éléments en tant que traceurs des eaux à signature évaporitique. Associé aux autres traceurs, le rapport Li/SO4 s'est révélé très pertinent dans la discrimination des eaux d'origine évaporitique ou géothermale et semble donc pouvoir être utilisé comme un outil supplémentaire intéressant dans l'étude de ce type de système hydrogéologique.
Ces résultats devront être précisés par l'utilisation d'autres traceurs, tels que le 36Cl, dans le but d'établir des schémas de circulation karstique intégrant les apports profonds et les venues marines en relation avec la géométrie interne des aquifères. Cet aspect est un élément essentiel dans la gestion de la ressource karstique, en particulier au voisinage du littoral. À ce titre, la démarche pourrait intéresser d'autres régions du Bassin méditerranéen.
Remerciements
Les auteurs tiennent à remercier M.A. Cordier et S. Van Exter (laboratoire HydroSciences Montpellier), ainsi que Roland Simler (laboratoire d'hydrogéologie d'Avignon) pour leur aide dans le dosage des échantillons.