Version française abrégée
Introduction
Dans le Petit Caucase, les ophiolites de Stepanavan (Fig. 1) appartiennent à la zone de suture entre la marge eurasienne et le bloc sud-arménien, d’origine gondwanienne [5,9,16–18,20]. Un assemblage ophiolitique complet est décrit au nord du lac Sevan en Arménie (la zone ophiolitique Amassia–Sevan–Akera) (par exemple, [1,21]). Cependant, des affinités géochimiques alcalines et calco-alcalines ont été décelées dans les laves de l’ophiolite [25], et interprétées comme des séries d’arc volcanique, dont les relations avec le reste de l’ophiolite restent confuses. L’ophiolite de Sevan est datée du Jurassique moyen : 168 ± 8 Ma (K–Ar sur biotite et muscovite de plagiogranite) [19] et 160 ± 4 Ma (U–Pb sur zircon de tonalite) [26]. L’obduction et la collision continentale sont supposées se produire au Crétacé supérieur, si l’on considère la discordance de calcaires récifaux Coniacien supérieur sur l’ophiolite [16,22].
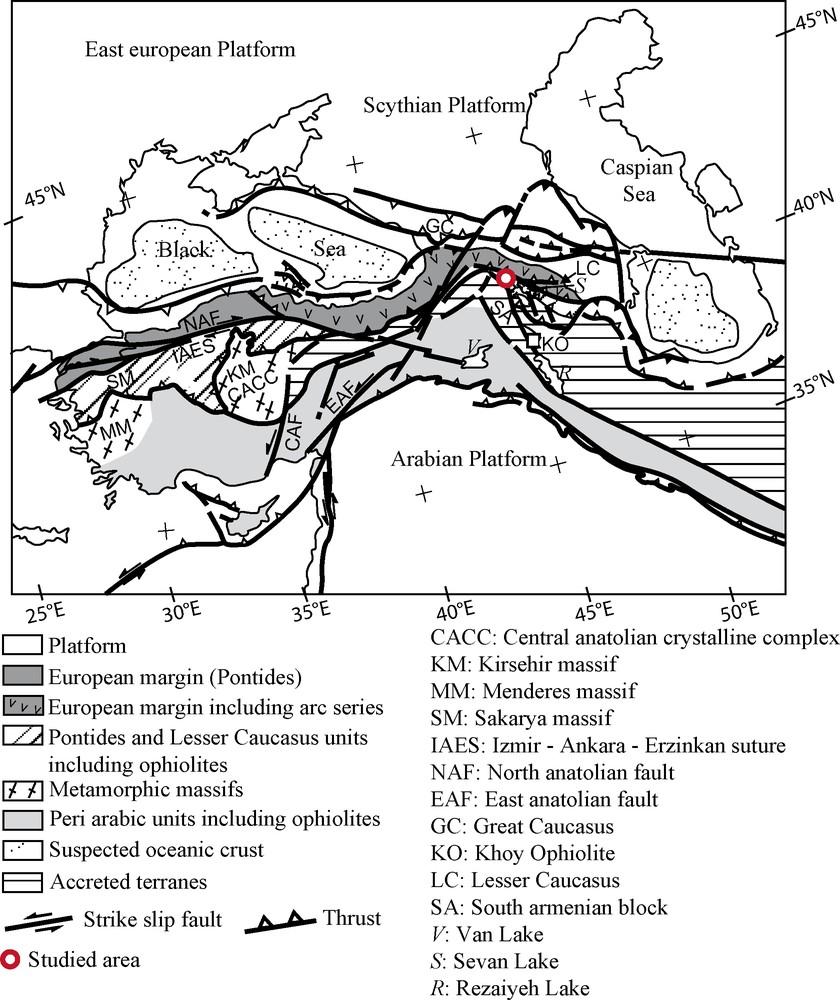
Structural map of the Arabia–Eurasia collision area, after [6].
Fig. 1. Carte structurale de la zone collisionnelle Arabie–Eurasie, d’après [6].
Le massif ophiolitique étudié se situe à 5 km au sud-ouest de Stepanavan (Nord-Ouest de l’Arménie, Fig. 2). Sur une coupe NE–SW, Aghamalyan [2,3] décrit la succession suivante, de bas en haut : (1) un para-autochtone constitué de pélites interstratifiées, avec des coulées de lave dacitiques, surmontées par des calcaires hydrothermalisés (« listwenite ») ; (2) une unité de schistes à glaucophane, incluant des blocs de schistes à grenat–amphibole, charriés sur (1) ; (3) des mélanges ophiolitiques comprenant des ultrabasites, des roches volcaniques et des radiolarites ; (4) des conglomérats, qui marquent une discordance, surmontés de calcaires ; (5) une unité constituée de roches volcaniques du Paléocène–Éocène, chevauchée au nord par l’ensemble (1–4). L’âge de l’ensemble (1–4) est présumé être Crétacé inférieur à supérieur, par analogie avec celui du massif voisin d’Amassia, pour lequel des brachiopodes de cet âge ont été décrits dans les lentilles de calcaires entre des volcanites [11] et pour lequel aussi des âges K–Ar sur roche totale mesurés sur les schistes bleus suggèrent un âge Crétacé supérieur (90–80 Ma) [4].
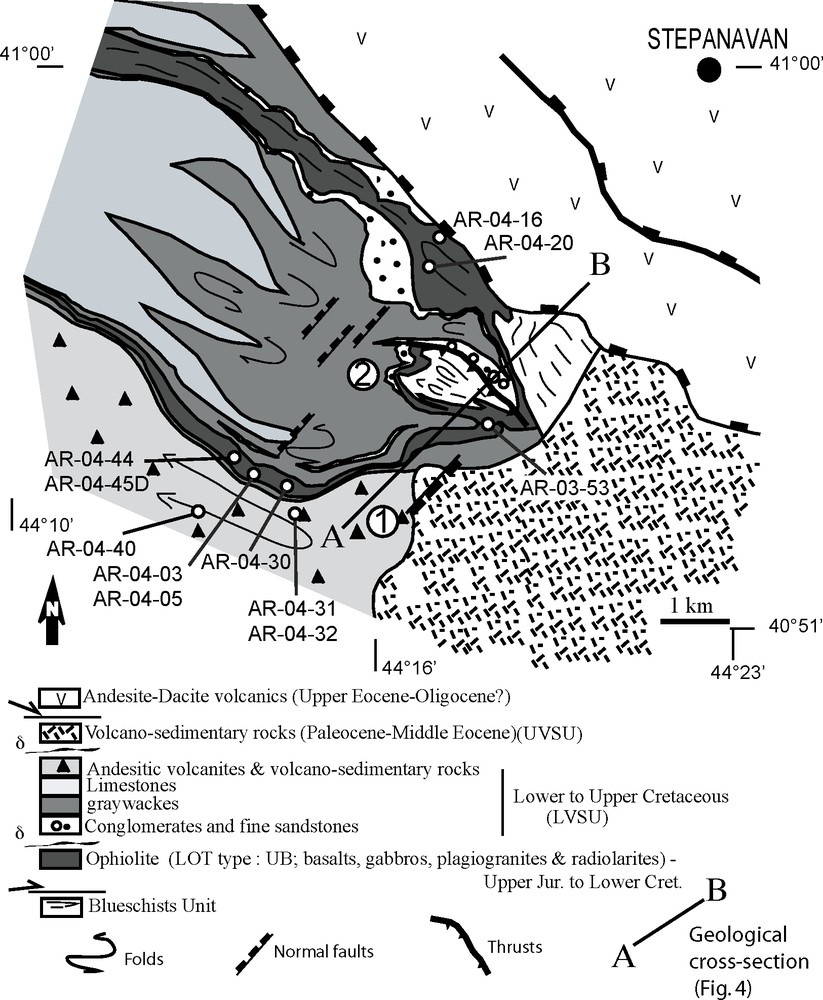
Simplified geological map of the Stepanavan ophiolitic complex (northwestern Armenia). 1 and 2 are the locations of the two lithological profiles (Fig. 4).
Fig. 2. Carte géologique simplifiée du complexe ophiolitique de Stépanavan (Nord-Ouest de l’Arménie). Les emplacements 1 et 2 sont les localisations des deux profils lithologiques de la Fig. 4.
Nouvelles observations
De nouvelles données structurales et pétrographiques (Figs. 2–4) montrent que (1) les schistes bleus apparaissent en fenêtre sous (2a) des ophiolites métamorphisées dans le faciès épidote–amphibolite, qui correspond (2b) à la semelle des ophiolites non métamorphiques, surmontées par (3) des pélites brunes intercalées de calcaires, passant latéralement à des coulées de laves en coussins et de tufs volcaniques, recouverts en discordance (4) par les séries volcaniques du Paléocène–Éocène.
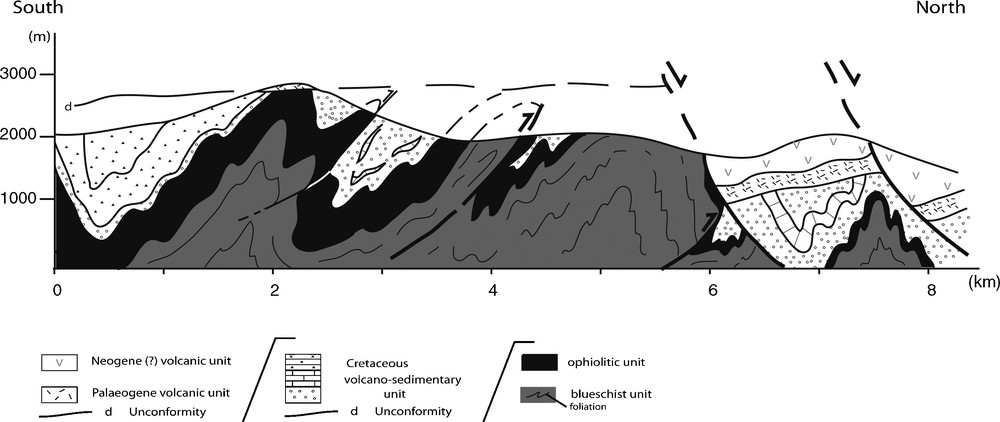
Geological cross-section of the Stepanavan ophiolitic complex (the location of the section is indicated in Fig. 2).
Fig. 3. Coupe géologique du complexe ophiolitique de Stépanavan (localisation de la coupe sur la Fig. 2).
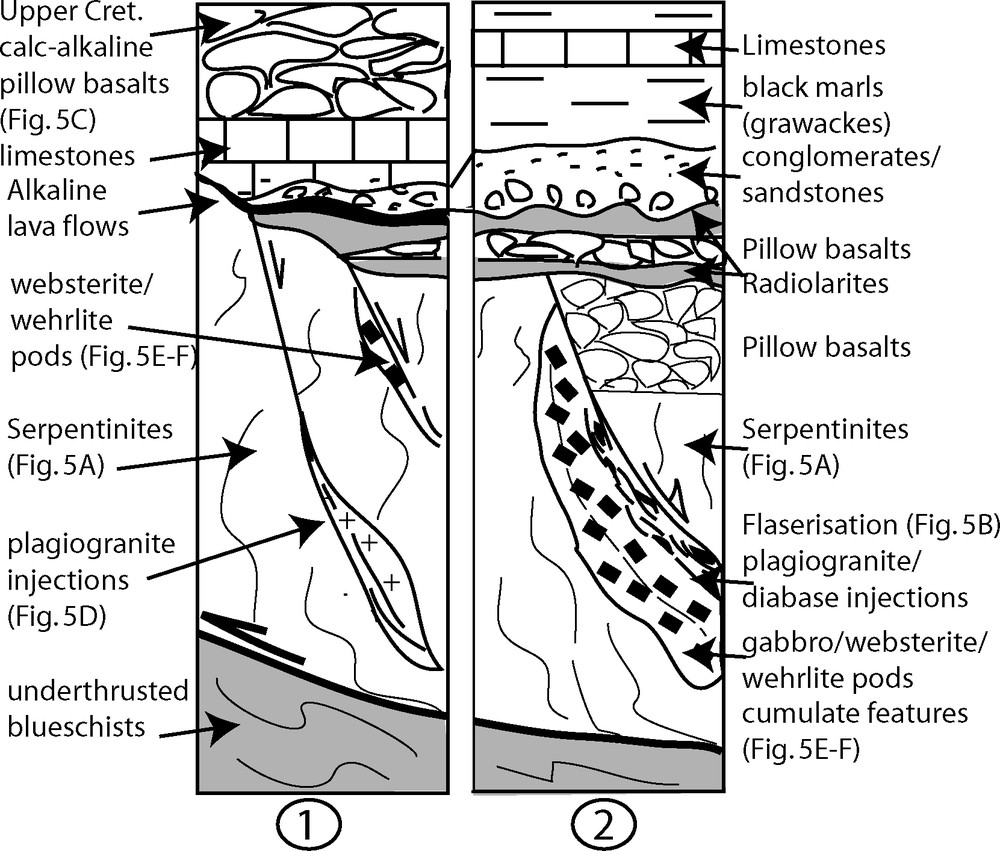
Lithological profiles of the two localities of the studied area, showing the structural relationships between units1 and 2 (the location of the profiles is indicated in Fig. 2).
Fig. 4. Profils lithologiques effectués à partir des observations de deux parties de la zone étudiée, 1 et 2 (localisation des profils sur la Fig. 2).
Les ophiolites se composent d’une séquence mantellique serpentinisée, de petits corps intrusifs de gabbros lités dans les péridotites serpentinisées ; le tout est surmonté par des coulées basaltiques en pillow lavas peu épaisses et très variables latéralement (Fig. 4). Le complexe filonien est très réduit. Les laves basaltiques reposent le plus souvent directement sur le complexe gabbro-péridotitique, tectonisé et hydrothermalisé. Elles sont elles-mêmes recouvertes par des radiolarites (Fig. 4). Une étude préliminaire des radiolarites a révélé un âge Jurassique supérieur [8].
Pétrographie, minéralogie et géochimie
La série ophiolitique
Les roches plutoniques de l’ophiolite correspondent à la série évolutive suivante : péridotites, gabbros (à olivine, à deux pyroxènes, à amphibole), gabbro-norites, anorthosites, plagiogranites. Les roches volcaniques présentes dans l’ophiolite ont une composition basaltique.
Les wehrlites sont à olivine Fo87–88 (>60–65 %) et diopside (Wo45–47En48–50Fs2–4). Les webstérites sont composées d’orthopyroxène (30–70 %) de type enstatite (Wo1–5En59–84Fs11–37), de clinopyroxène (70–30 %) de type augite (Wo35–42En36–40Fs15–19), et d’olivine (0–35 %, Fo84–88). Les gabbro-norites contiennent une majorité de plagioclase (>55–60 %, 1–3 mm, bytownite, An80–85) et une minorité d’enstatite (Wo2–5En59–61Fs34–37) et d’augite (Wo35–42En36–40Fs15–19). Les gabbros sont essentiellement à plagioclase labrador (55–85 %), à clinopyroxène (10–40 %, Wo40–41En33–42Fs11–19) et amphibole magnésio-hornblende secondaire. Le plagiogranite est formé de plagioclase sodique An20 (∼65–70 %), de quartz (25–30 %) et de traces de biotite et d’amphibole.
Les échantillons de basaltes en coussin présentent des structures intersertales à ophitiques microdoléritique et métadoléritique. L’assemblage minéralogique le plus fréquent est plagioclase (55–65 %), clinopyroxène (25–35 %), titanomagnétite, ilménite et hématite (∼5–12 %). L’olivine est absente. Les basaltes et les gabbros ont des spectres plats, avec des anomalies négatives légères en Nb–Ta (Fig. 6A et B).
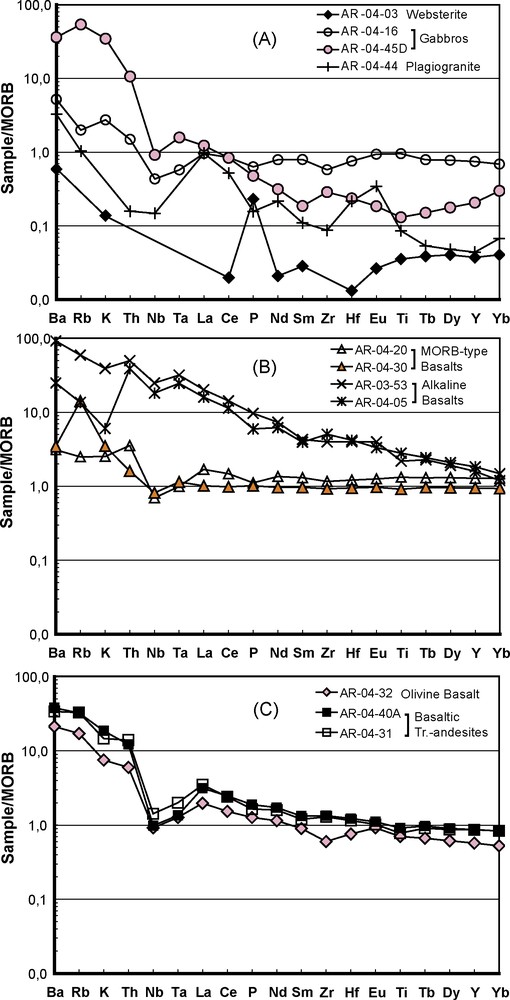
Spidergrams of Stepanavan magmatic rocks. (A), Spidergrams of plutonic rocks from the ophiolite sequence. (B), Spidergrams of volcanic rocks from the ophiolite sequence and from the alkaline lava flows. (C), Spidergrams of volcanic rocks from the calc-alkaline sequence. MORB normalisation values are from Sun and McDonough [23].
Fig. 6. Diagrammes étendus des séries magmatiques de Stépanavan. (A), Roches plutoniques de l’ophiolite. (B), Roches volcaniques de la série ophiolitique et laves alcalines. (C), Laves calco-alcalines. Les spectres sont normalisés par rapport aux compositions du MORB [23].
Les laves alcalines
Les laves alcalines sont des trachybasaltes formés essentiellement de plagioclase (55–65 %), de clinopyroxène (∼30 %) et de 5–10 % de titanomagnétite, avec quelques cristaux d’amphibole brune et d’apatite. Elles présentent des enrichissements prononcés en terres rares légères ((Ce/Yb)n = 4.9–7.6) et n’ont pas d’anomalie négative en Nb et Ta (Fig. 6B).
Les laves calco-alcalines
Les laves calco-alcalines sont des basaltes et des andésites basaltiques, comprenant des phénocristaux de plagioclase oligoclase-andésine et de clinopyroxène augite (Wo36–38En42–43Fs13–15). Elles présentent un enrichissement fort en éléments à grands rayons ioniques, modéré en terres rares légères, et des anomalies négatives marquées en Nb et Ta (Fig. 6C).
Discussion et conclusion
Les données structurales révèlent une structure complexe de nappes plissées. Les unités tectoniques se sont mises en place et ont été plissées au cours du Crétacé supérieur–Paléocène, postérieurement au métamorphisme « schistes bleus », daté entre 95 et 80 Ma [4] et avant la transgression qui a eu lieu au cours du Paléocène–Éocène.
- 1. L’ophiolite d’âge Jurassique peut être corrélée aux autres affleurements ophiolitiques jurassiques du Nord de l’Arménie. Les assemblages lithologiques et les caractéristiques pétro-géochimiques de l’ophiolite sont en accord avec une ophiolite correspondant à une lithosphère océanique, créée par une dorsale lente (SLOT) en contexte d’arrière-arc.
- 2. Cette ophiolite est recouverte par des laves alcalines, comme dans les autres ophiolites du Petit Caucase [10], correspondant vraisemblablement à la mise en place d’un plateau océanique.
- 3. La présence des schistes bleus et d’une série calco-alcaline interprétée comme une série d’arc volcanique indique la mise en place d’une subduction intra-océanique au cours du Crétacé supérieur.
- 4. La discordance marquée par un niveau d’érosion et des conglomérats, puis des calcaires récifaux coniaciens indique un soulèvement de la lithosphère océanique, peut-être en réponse à l’obduction de l’ophiolite sur le bloc Sud-Arménien.
Ces nouveaux résultats concernant cette chaîne téthysienne vont permettre de mieux contraindre son évolution géodynamique, qui est actuellement en cours de réinterprétation. Les caractéristiques pétrochimiques récemment mises en évidence dans l’ophiolite Crétacé supérieur de Khoy (Nord-Ouest de l’Iran) suggèrent un contexte géodynamique similaire [13–15]. Cependant, la corrélation entre ces deux ophiolites reste difficile, car (1) les caractéristiques SLOT de Khoy ont été acquises au Crétacé supérieur, et (2) une obduction de plus de 100 km sur le bloc Sud-Arménien est nécessaire. On peut aussi envisager que l’ophiolite nord-arménienne et l’ophiolite jurassique de Khoy [4] se soient formées en même temps, mais dans deux bassins parallèles, de part et d’autre du bloc Sud-Arménien.
1 Introduction
The age and petrogeochemical features of the Lesser Caucasus ophiolites in Armenia are of great importance for lateral correlations in the Tethyan ophiolite belts of the Middle East. Because ophiolite locations are reported in the Lesser Caucasus and because this zone is intermediate between the Mediterranean–Anatolian and Iran–Himalayan domains, key data on the structure, age and geochemical features of Armenian ophiolites are necessary for Tethyan palaeo-tectonic reconstructions.
2 Geological setting
The Stepanavan ophiolite is part of the Lesser Caucasus ophiolite belt (Amassia–Sevan–Akera ophiolites) (Fig. 1), striking SE–NW in northern Armenia [5,9,20], generally interpreted as a suture zone [18] between Eurasia and the Gondwanian-originated South-Armenian Block [16,17]. While a complete ophiolite sequence has been described in the Sevan area (e.g., [1,21]), some alkaline and calc-alkaline affinities have also been described and interpreted by Tsameryan et al. [25] as resulting from volcanic-arc environments. However, the relationship between these lavas and the rest of the ophiolite has not been clearly established. The Sevan ophiolite is dated at 168 ± 8 Ma (K–Ar on biotite and muscovite from a plagiogranite) [19], at 160 ± 4 Ma (U–Pb on zircon from a tonalitic intrusion) [26]. The ophiolite is generally weakly deformed, and it has undergone phrenite–pumpellyite- to greenschist-facies metamorphism and hydrothermalism. The age of oceanic closure and suturing is presumed to be Upper Cretaceous based on the unconformity of platform-type Upper Coniacian limestones over the ophiolite [16,22]. In the Stepanavan area, previous works by Aghamalyan [2,3] have shown the following structural succession on a NE–SW section: (1) a basal part comprising pelites interstratified with dacitic lava flows, and hydrothermalised limestones (‘listwenite’); (2) a glaucophane-schist unit including blocks of garnet amphibolites thrust over (1); (3) ophiolitic mélanges comprising ultramafites, volcanic rocks, and radiolarites; (4) a conglomeratic and overlying limestone unit overlying unconformably the unit (3); (5) to the southeast, Palaeocene–Eocene series thrust by (1–4). The series (1–4) are attributed a Lower to Upper Cretaceous age, by analogy with the neighbouring Amassia massif (located ∼30 km west of the Stepanavan massif), where brachiopods have been described in limestones interstratified within lava flows [11], and whole-rock K–Ar ages obtained on blueschist-facies metamorphic rocks range in the Upper Cretaceous (90–80 Ma) [4].
3 Results
3.1 Field geology
Structural observations and cartography allow us to clarify the geometry and structural relationships of the different units. Several units can be distinguished, which have been tectonically superposed (Figs. 2–4). (1) The lower part of the structural pile is formed by blueschists. These appear in small, ∼2-km2-scale, tectonic windows below (2a) epidote–amphibolite facies meta-ophiolites, which are the tectonic sole of (2b) unmetamorphized ophiolites unconformably covered by (3) brown pelites and limestones. The latter pass laterally to pillow-lava flows and volcanic tuffs, unconformably recovered by (4) Palaeocene–Eocene acidic volcanic series.
Ophiolites are formed by highly serpentinized and deformed peridotites, crosscut by small (100 m-large) intrusive bodies (Figs. 4 and 5), whose composition evolved from werhlites to laminated gabbros and more evolved melts (plagiogranites). Wehrlites and websterites display undeformed cumulative features (Fig. 5E–F), while gabbro-norites and gabbros (Fig. 5B and D) are generally strained and flaserized, and plagiogranites mark narrow high-strain zones that crosscut the gabbro pods (Figs. 4 and 5D). These deep plutonic parts are exposed at sea level, are hydrothermalised (‘listwenites’), and are unconformably overlain by pillow lavas and radiolarites (Figs. 4 and 5C). Lava flows are scattered, and it is not rare to observe serpentinized peridotites overlain by radiolarites. There is very scarce occurrence of diabase dikes; they are often strained and they crosscut flaserized gabbro (Fig. 5B). Dating of radiolarian faunas has provided an Upper Jurassic age [8].
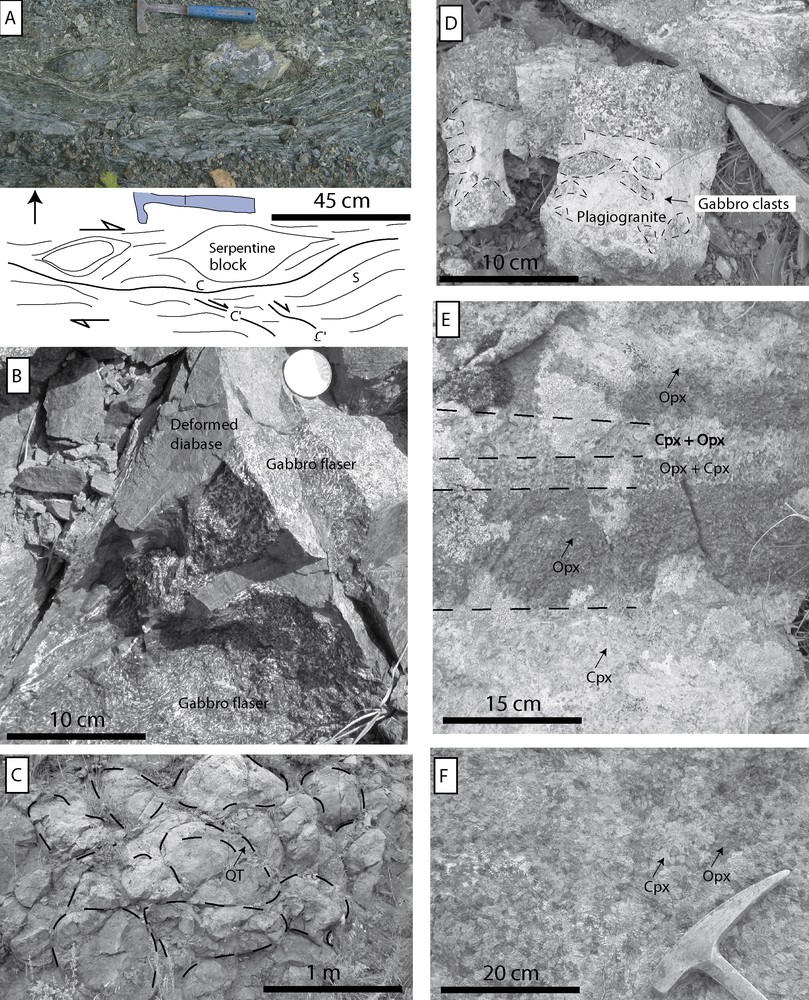
Representative photographs of the main lithologies of the Stepanavan ophiolite complex, described in the text, and lithological profile (Fig. 4).
Fig. 5. Photographies représentatives des lithologies principales du complexe ophiolitique de Stépanavan, décrites dans le texte et dans le profil lithologique (Fig. 4).
3.2 Petrography and mineral chemistry
Mineral chemistry analysis was undertaken at the OPGC (Clermont-Ferrand, France), with a Cameca SX 100.
Petrographically, the rocks from the Stepanavan ophiolite can be divided into three groups:
- • the ophiolitic suite: a magmatic series from ultramafic cumulates to gabbros and plagiogranites, associated with effusive pillow lavas;
- • alkaline lavas overlying the ophiolite;
- • andesitic pillow lavas.
3.2.1 The ophiolitic suite
Wehrlites have a poikilitic texture showing numerous clinopyroxene crystals with diopside composition (Wo45–47En48–50Fs2–4), included in large olivine Fo87–88 (>60–65%) porphyric grains. Websterites have a granular texture, with large 2–8 mm porphyrocrysts of orthopyroxene (30–70%), clinopyroxene (70–30%), and olivine grains (0–35%). Orthopyroxenes are enstatite-rich (Wo1–5En59–84Fs11–37); olivine is relatively rich in forsterite (Fo87–88). Gabbro-norites have a plagioclase-rich (∼60%, 1–3 mm) gabbroic texture, with less clino- and orthopyroxene. Plagioclase is of bytownite type (An80–85), while orthopyroxenes are enstatites (Wo2–5En59–61Fs34–37), and clinopyroxens are augite (Wo35–42En36–40Fs15–19), olivine being relatively rich in forsterite (Fo84–88). Most commonly found gabbros have an allotriomorphic granular texture (2–4 mm), with 55–70% Labrador plagioclase, 30–40% amphibole, 5–10% augite clinopyroxene (Wo40–41En39–42Fs11–14), and secondary amphibole. Amphiboles with magnesio-hornblende compositions [XMg (Mg/Mg + Fe) ∼ 0.6] appear at augite's rims. Anorthosite gabbros are found, with essentially altered plagioclase (∼85%) and 15% of augite-type clinopyroxene (Wo40–41En33–35Fs18–19). Plagiogranites are allotriomorphic microgranular rocks (0.1–2.5 mm), with almost 70% plagioclase of oligoclase composition (An15–25), interstitial quartz (25%), and traces of biotite and amphibole. Pillow lavas have intersertal to doleritic textures showing abundant feldspar phenocrysts and microliths (55–65%) and minor clinopyroxene (25–35%); no olivine has been observed.
3.2.2 Alkaline lavas
These are lying above the hydrothermalised surface of the ophiolite, in several locations, as large lava flows and pillow-lava basaltic sequences or dykes of diabase. They have intersertal to ophitic and doleritic textures and are formed by plagioclase (55–65%), clinopyroxene (∼30%), and 5–10% titanomagnetite, with small brown amphibole and apatite needles.
3.2.3 Calc-alkaline lavas
They consist of large pillow lavas of basaltic and basaltic andesite compositions with microcryptocrystalline to intersertal textures of large andesine-oligoclase plagioclase phenocrysts (2–7 mm) and microliths, and minor augite (Wo36–38En42–43Fs13–15) clinopyroxene. These lavas overlie Upper Cretaceous limestones, unconformably lying on the ophiolite s.s.
3.3 Geochemistry
Major and trace element analyses were undertaken at the CRPG Nancy, France. Major element analysis was undertaken by ICPAES and trace elements by ICPMS. For details on the equipment used and analytical errors, consult the website: http://www.crpg.cnrs-nancy.fr.
3.3.1 Ophiolitic suite
Major element analysis (Table 1) shows a calc-alkaline-to-tholeiitic composition, with slight alkaline affinity in SiO2 vs. alkaline elements and in AFM diagrams (not shown in this paper).
Representative whole-rock analyses of samples from the Stepanavan area. Major oxides in wt%, and trace elements and REE in ppm
Tableau 1 Analyses roche totale représentatives des échantillons du complexe ophiolitique de Stepanavan. Éléments majeurs en %poids, et traces en ppm
Sample | Webste-rite | Gabbros | Plagio-granite | Ophiolitic Basalts | Alkaline basalts | Olivine Basalt | Basaltic Trachyandesites | ||||
n° | AR-04-03 | AR-04-16 | AR-04-45D | AR-04-44 | AR-04-20 | AR-04-30 | AR-03-53 | AR-04-05 | AR-04-32 | AR-04-40A | AR-04-31 |
SiO2 | 53.24 | 4.30 | 53.77 | 75.35 | 51.53 | 48.55 | 48.54 | 50.19 | 49.15 | 49.79 | 52.20 |
Al2O3 | 1.03 | 14.39 | 14.00 | 12.20 | 14.69 | 13.29 | 15.01 | 13.91 | 18.53 | 15.80 | 17.05 |
Fe2O3 | 6.02 | 12.90 | 8.92 | 2.71 | 14.81 | 8.67 | 12.65 | 13.73 | 10.19 | 8.82 | 9.28 |
MnO | 0.15 | 0.21 | 0.15 | 0.03 | 0.23 | 0.15 | 0.27 | 0.24 | 0.16 | 0.15 | 0.16 |
MgO | 23.18 | 9.11 | 7.81 | 0.77 | 4.15 | 6.86 | 4.25 | 3.27 | 5.25 | 3.54 | 3.59 |
CaO | 16.52 | 10.14 | 6.98 | 2.05 | 4.86 | 10.49 | 5.33 | 5.85 | 8.25 | 9.12 | 6.56 |
Na2O | 0.12 | 2.93 | 3.34 | 5.03 | 5.74 | 4.74 | 3.93 | 5.11 | 4.36 | 3.54 | 4.61 |
K2O | – | 0.19 | 2.42 | – | 0.18 | 0.24 | 2.69 | 0.42 | 0.52 | 1.24 | 1.00 |
TiO2 | 0.05 | 1.18 | 0.16 | 0.11 | 1.62 | 1.08 | 2.64 | 3.39 | 0.86 | 1.07 | 0.94 |
P2O5 | 0.03 | 0.07 | 0.05 | 0.02 | 0.13 | 0.11 | 1.08 | 0.67 | 0.14 | 0.20 | 0.18 |
LOI | 0.54 | 1.76 | 2.44 | 1.07 | 1.89 | 6.01 | 3.16 | 2.94 | 3.12 | 7.27 | 5.36 |
Total | 100.9 | 100.2 | 100.1 | 99.3 | 99.8 | 100.2 | 99.5 | 99.7 | 100.5 | 100.6 | 100.9 |
Mg# | 90.0 | 60.4 | 65.6 | 38.1 | 37.7 | 63.1 | 42.3 | 34.0 | 52.7 | 48.4 | 44.5 |
Rb | – | 1.12 | 30.17 | 0.58 | 1.4 | 7.94 | 33.17 | 7.62 | 9.61 | 18.12 | 18.45 |
Sr | 11.79 | 125.4 | 213.4 | 91.04 | 61.01 | 95.7 | 322.6 | 198.8 | 520.3 | 303.8 | 282.3 |
Y | 1.05 | 20.91 | 5.79 | 1.23 | 35.91 | 26.52 | 44.41 | 51.24 | 16.0 | 24.4 | 24.19 |
Zr | – | 42.74 | 21.26 | 6.48 | 86.0 | 68.43 | 294.4 | 373.5 | 44.4 | 99.18 | 95.3 |
Nb | – | 1.01 | 2.14 | 0.35 | 1.62 | 1.9 | 57.95 | 42.33 | 2.14 | 2.29 | 3.32 |
Ba | 3.71 | 32.71 | 228.1 | 20.58 | 19.58 | 21.73 | 578.3 | 156.6 | 133.9 | 239.1 | 213.6 |
Hf | – | 1.21 | 0.63 | 0.15 | 2.54 | 1.83 | 6.51 | 8.01 | 1.25 | 2.69 | 2.61 |
Ta | – | 0.08 | 0.21 | – | 0.13 | 0.15 | 4.20 | 3.24 | 0.17 | 0.18 | 0.26 |
Pb | – | – | 3.61 | – | 2.29 | 1.93 | 2.54 | 2.24 | 7.22 | 3.42 | 5.66 |
Th | – | 0.18 | 1.27 | 0.02 | 0.43 | 0.19 | 5.98 | 4.65 | 0.72 | 1.46 | 1.67 |
U | – | 0.05 | 0.43 | 0.01 | 0.12 | 0.09 | 1.46 | 1.20 | 0.19 | 0.67 | 0.58 |
V | 135.1 | 324.7 | 94.47 | 30.74 | 459.8 | 305.4 | 94.35 | 201.6 | 241.5 | 279.1 | 263.7 |
Cr | 2804 | 236.4 | 324.2 | 421.5 | 99.23 | 316.7 | 25.77 | – | 21.05 | 31.91 | 73.83 |
Co | 55.82 | 51.46 | 31.5 | 7.71 | 38.85 | 42.98 | 16.75 | 31.93 | 29.51 | 29.05 | 27.36 |
Ni | 361.3 | 78.0 | 101.7 | 24.86 | 22.76 | 109.1 | – | – | 15.55 | 22.38 | 18.95 |
Cu | 340.3 | – | 189.9 | 189.5 | 64.58 | 132.8 | 9.48 | 14.94 | 12.6 | 188.1 | 170.9 |
Zn | 25.68 | 60.28 | 60.33 | 23.13 | 130.6 | 81.13 | 137.1 | 152.7 | 150.9 | 86.53 | 100.0 |
La | – | 2.40 | 3.06 | 2.42 | 4.23 | 2.53 | 50.59 | 40.02 | 4.93 | 7.87 | 8.69 |
Ce | 0.15 | 6.37 | 6.28 | 3.90 | 11.08 | 7.37 | 107.0 | 85.12 | 11.46 | 18.51 | 18.05 |
Pr | 0.02 | 1.08 | 0.65 | 0.42 | 1.88 | 1.31 | 12.97 | 10.85 | 1.74 | 2.68 | 2.53 |
Nd | 0.15 | 5.76 | 2.30 | 1.58 | 9.88 | 7.04 | 53.32 | 45.27 | 8.35 | 12.58 | 11.65 |
Sm | 0.08 | 2.09 | 0.49 | 0.29 | 3.44 | 2.55 | 11.26 | 10.35 | 2.36 | 3.47 | 3.15 |
Eu | 0.03 | 0.96 | 0.19 | 0.35 | 1.28 | 0.99 | 4.08 | 3.39 | 0.94 | 1.13 | 1.04 |
Gd | 0.13 | 2.98 | 0.55 | 0.24 | 4.69 | 3.49 | 10.41 | 10.3 | 2.60 | 3.89 | 3.57 |
Tb | 0.03 | 0.53 | 0.10 | 0.04 | 0.87 | 0.65 | 1.52 | 1.63 | 0.44 | 0.65 | 0.61 |
Dy | 0.18 | 3.53 | 0.81 | 0.22 | 5.93 | 4.34 | 8.69 | 9.50 | 2.80 | 4.09 | 3.96 |
Ho | 0.04 | 0.74 | 0.19 | 0.05 | 1.28 | 0.93 | 1.57 | 1.80 | 0.57 | 0.85 | 0.83 |
Er | 0.12 | 2.15 | 0.64 | 0.15 | 3.79 | 2.75 | 4.16 | 4.93 | 1.62 | 2.46 | 2.46 |
Tm | 0.02 | 0.32 | 0.12 | 0.03 | 0.58 | 0.42 | 0.57 | 0.70 | 0.24 | 0.38 | 0.38 |
Yb | 0.12 | 2.10 | 0.91 | 0.21 | 3.89 | 2.84 | 3.64 | 4.51 | 1.60 | 2.54 | 2.54 |
Lu | 0.02 | 0.32 | 0.17 | 0.04 | 0.61 | 0.44 | 0.56 | 0.69 | 0.25 | 0.39 | 0.40 |
Eu/Eu* | 0.85 | 1.17 | 1.12 | 4.05 | 0.98 | 1.02 | 1.15 | 1.0 | 1.15 | 0.94 | 0.95 |
(La/Sm)N | 0.0 | 0.72 | 3.94 | 5.25 | 0.77 | 0.63 | 2.83 | 2.43 | 1.31 | 1.43 | 1.74 |
(La/Yb)N | 0.0 | 0.77 | 2.27 | 7.92 | 0.73 | 0.60 | 9.38 | 5.99 | 2.07 | 2.09 | 2.31 |
Ultramafic cumulative plutonic rocks are featured by lower trace element contents than associated lavas due to their cumulative origin. The cumulative nature of plagiogranites is also featured by positive europium anomaly (Eu/Eu* = 4.05), ascribed to high plagioclase contents. Multi-element plots of basalts and gabbros show flat REE spectra (Fig. 6A and B), ascribed to an E-MORB-type signature [13]. However, relatively flat REE spectra and the presence of Nb–Ta negative anomalies are more typical of primitive arc lavas [24]. These features are interpreted as representative of a back-arc setting, as back-arc lavas commonly show weak-arc geochemical features (e.g., [22], Fig. 4).
3.3.2 Alkaline lavas
Alkaline lavas have basaltic trachyandesite compositions, showing neat LREE enrichments [(Ce/Yb)n = 4.9–7.6] and no clear Nb–Ta anomaly (Fig. 6B). These are features typical of intraplate magmas (Fig. 7). The intraoceanic affinity of such magmatism may be interpreted in terms of an oceanic plateau emplacement or more restricted OIB. However, ongoing work [11] on the other Armenian ophiolites (Sevan, Vedi) shows that similar and locally thick (∼1 km) alkaline successions are present on top of the Jurassic ophiolite, which suggests a large-scale oceanic plateau magmatic event.
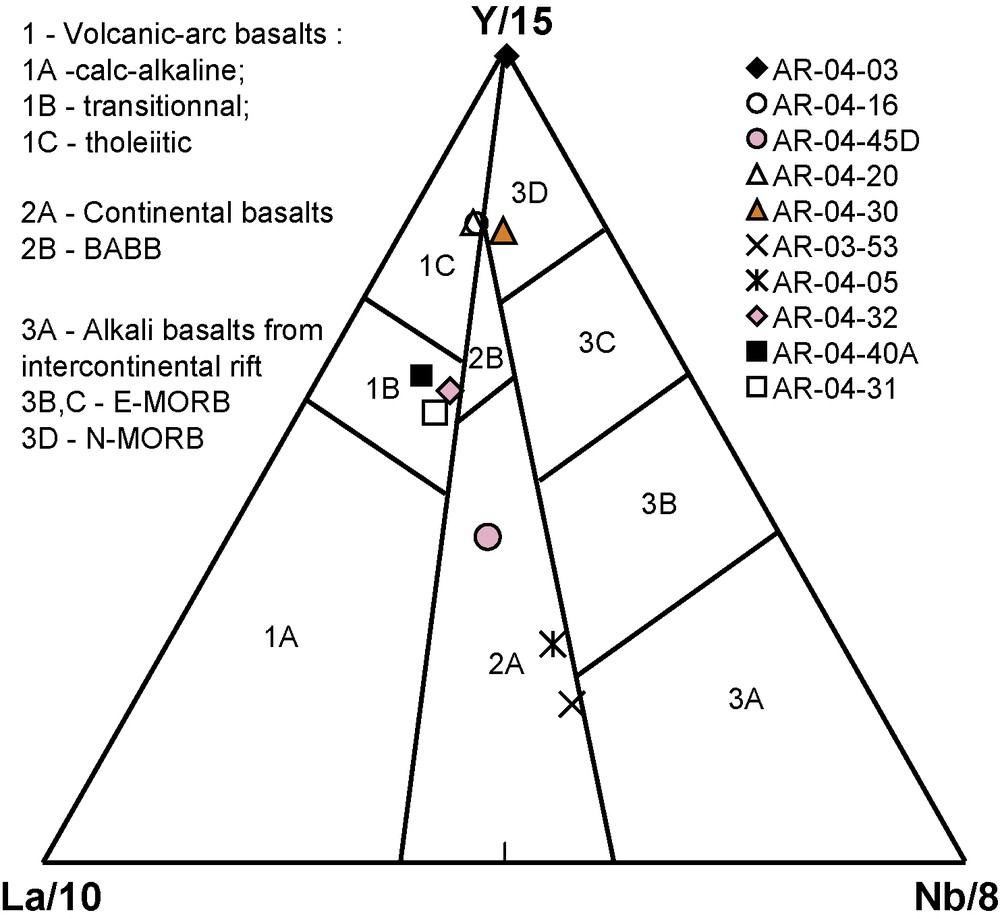
La vs. Y vs. Nb diagram of Cabanis and Lecolle [7], showing the geochemical affinity of the three magmatic series.
Fig. 7. Diagramme La–Y–Nb de Cabanis et Lecolle [7], montrant les affinités géochimiques des trois séries magmatiques.
3.3.3 Calc-alkaline lavas
Calc-alkaline pillow-lavas display LREE [(Ce/Yb)n = 1.8–1.9] and LILE enrichments, and marked negative Nb, Ta anomalies (Fig. 6C). These features are typical of relatively immature island arc subduction-related setting [24].
4 Discussion and conclusions
4.1 Superposition of three magmatic suites in one ‘ophiolite’
The new field and petrogeochemical data exposed in this paper highlight a complex tectonic structure, where several units of various geodynamic origins have been superposed. The tectonic units were superposed and the nappe contacts were folded before the Palaeocene–Eocene unconformity, suggesting that tectonic superposition events occurred in a north–south-shortening context between the Upper Cretaceous 40Ar/39Ar age of blueschists (95–90 Ma) (Rolland et al., in prep.) and the Palaeocene–Eocene. The Upper Cretaceous calc-alkaline island arc suite is thus interpreted as sub-contemporaneous with blueschist metamorphism. It may therefore originate from a north-dipping subduction zone, active during the Upper Cretaceous. The basal unconformity of calc-alkaline pillow lavas above platform-type limestones and the ophiolite s.s. suggests that the ophiolite has been exhumed previously to the arc emplacement. Interlayered alkaline lavas, interpreted as remnants of an oceanic plateau, might explain such an uplift, or it might be due to the onset of the subduction zone below the ophiolite. The extent and exact age of alkaline magmatism and its possible relationships with an oceanic plateau are still undefined. Finally, the ophiolite itself is only slightly metamorphosed, and its Jurassic age is in agreement with other ophiolites from the Amassia–Sevan–Akera suture zone.
4.2 The Stepanavan ophiolite is a slow-spreading type (SLOT)
The magmatic suite exposed in the Stepanavan area is typical of a slow-spreading ridge environment. (1) A reduced sheeted diabase dike swarm was observed. (2) Effusive lavas are scarce and phyric, with abundant plagioclase. (3) An overall fractional crystallisation sequence may be observed from lherzolites to biotite-bearing plagiogranites. (4) The exposure of the mantle and of deep zones of magmatic chambers at sea level is indicative of large extensional offsets along normal faults, and of minor magmatic accretion.
These petrological features suggest low partial melting rates, and significant fractional crystallisation of a relatively enriched mantle source. All the above features are typical of slow-spreading ridges such as the current Mid-Atlantic Ridge [12]. In addition, slight arc features such as the negative Nb-Ta anomaly are suggestive of a back-arc setting. Slow-spreading ridges are likely to be found in back–arc setting due to the small extent of opening rates in such marginal basins.
4.3 Correlations with other ophiolites from the Middle East
The petrogeochemical features and age of the Stepanavan ophiolite are in part similar to those of the Khoy ophiolite in northwestern Iran [13–15]; in particular, the Jurassic lower part of the ophiolite may be formed in a similar context. However, the upper part of the Khoy ophiolite is Upper Cretaceous in age, which is significantly younger than the Stepanavan ophiolite. In the Jurassic times, the same processes might be responsible for the formation of ophiolites in Khoy and Armenia, including a back-arc setting environment. Several hypotheses might be drawn at this stage of the study. (1) The North-Armenian ophiolite might represent the continuation of the Khoy ophiolite further north. This hypothesis is supported by the fact that the Armenian ophiolite is shown to be transported on a metamorphic sole, and is shown to be obducted onto the South-Armenian block [16,17]. This obduction might also connect to the basal contact of the Khoy ophiolite, as Jurassic ages are obtained in the basal part of the Khoy ophiolite [13–15]. However, this hypothesis would require at least 100 km of tectonic transport above the South-Armenian block. (2) The North-Armenian ophiolite might have been emplaced sub-contemporaneously, separated from the Khoy ophiolite by the South-Armenian block. It might connect to ophiolites outcropping in northern and northeastern Iran, from which age and petro-geochemical constraints are still lacking for comparisons.
Acknowledgements
The authors are grateful to the Geological Sciences Department of the Armenian Academy of Sciences. Financial support from the French Embassy in Armenia and the French Ministry for Foreign Affairs has allowed Mr Ghazar Galoyan to complete his PhD in France. This publication is contribution of ‘Geosciences Azur’, University of Nice–Sophia Antipolis, CNRS, France.