1 Introduction
The Sun ultimately defines the climate on Earth, supplying the planet with energy via radiation, which is received by the terrestrial system, but the role of the solar variability in climate variations is far from clear. Variability of the total solar irradiance (TSI) measured during the last decades is known to be too small to explain the observed climate variations [24,25,27,45]. On the other hand, there are other ways that solar variability may affect climate (see [34] for a review), e.g., an unknown long-term trend in TSI [68,95], a terrestrial amplifier of the spectral irradiance variations [33,66], or an indirect mechanism also driven by the solar activity. The latter can be realized by cosmic rays (CR) via the ionization effect in the atmosphere. Cosmic-ray-induced ionization (CRII) is the principle source of the ionization of the low and middle atmosphere and can slightly modulate cloud formation [20,52,71,73,81]. Even a small change in the cloud cover modifies the transparency/absorption/reflectance of the atmosphere and affects the amount of absorbed solar radiation, even with no changes in the solar irradiance. Since the flux of CR is modulated by the solar magnetic activity, this provides a link between solar variability and climate [47]. It is noteworthy that the CR flux on Earth is modulated not only by solar activity but also by the slowly changing geomagnetic field which does not allow the least energetic but most abundant CR particles to impinge on the Earth. Since the two CR modulation mechanisms are independent and act on different time scales, this gives an opportunity to study the CR effect on Earth. Here we present an overview of recent experimental evidence and theoretical considerations of the CR–climate link.
2 Cosmic ray variability
The CR intensity (energy-integrated flux measured by a certain device) is commonly measured by ground-based neutron monitors (NMs) which are effectively sensitive to CR with energy of a few tens of GeV [1]. The world network of NMs routinely measures the CR intensity since 1951. A usual proxy for CR intensity for longer time scales is the abundance (converted into the production rate in the atmosphere) of cosmogenic isotopes and in independently dated terrestrial archives such as tree rings, ice cores or marine sediments. While the effective energy of CR for the production of these isotopes is somewhat lower than that for NM, cosmogenic isotope data can still be roughly considered as an extension of the NM record in the past [6,61]. Variability of CR on different time scales is shown in Fig. 1. Panel a) depicts a ground level enhancement (GLE) due to a strong flux of solar energetic particles associated with the extreme solar flare of 20/01/2005. Such severe GLEs, when the CR intensity increases by a factor of 3–10 within an hour, occur 1–2 times per solar cycle. Panel b) shows a Forbush decrease caused by the passage of an interplanetary shock, when the CR intensity is suppressed by 10–20% for several days. There is a dozen such decreases per solar cycle. Solar modulation of CR on a decadal time scale is shown in panel c). One can also see a GLE in 1989 and a number of Forbush decreases. The CR flux, as measured by NMs, varies by about 25% in anti-phase with the solar activity, i.e. it is maximum during a solar minimum. The CR changes due to long-term solar variability are shown in panel d) and depict the 11-yr cycle superimposed on a long-term trend of 15% since the Maunder minimum in the 17th century. The multi-millennium CR variability, quantified in the production rate of radiocarbon cosmogenic isotope [79], is shown in panel e) for the Holocene (the 11 000-yr long period characterized by a pretty stable warm climate). The fast variability is caused by the solar activity, while the slow changing CR level is mostly related to the changing geomagnetic shielding. Panel f) depicts smooth variations of CR, measured as the concentration in deep marine sediments [26], that are caused by geomagnetic changes. Panel g) shows hypothetical changes in the CR flux (normalized to the modern flux ) due to the changing galactic environment of the solar system, as suggested by [64].
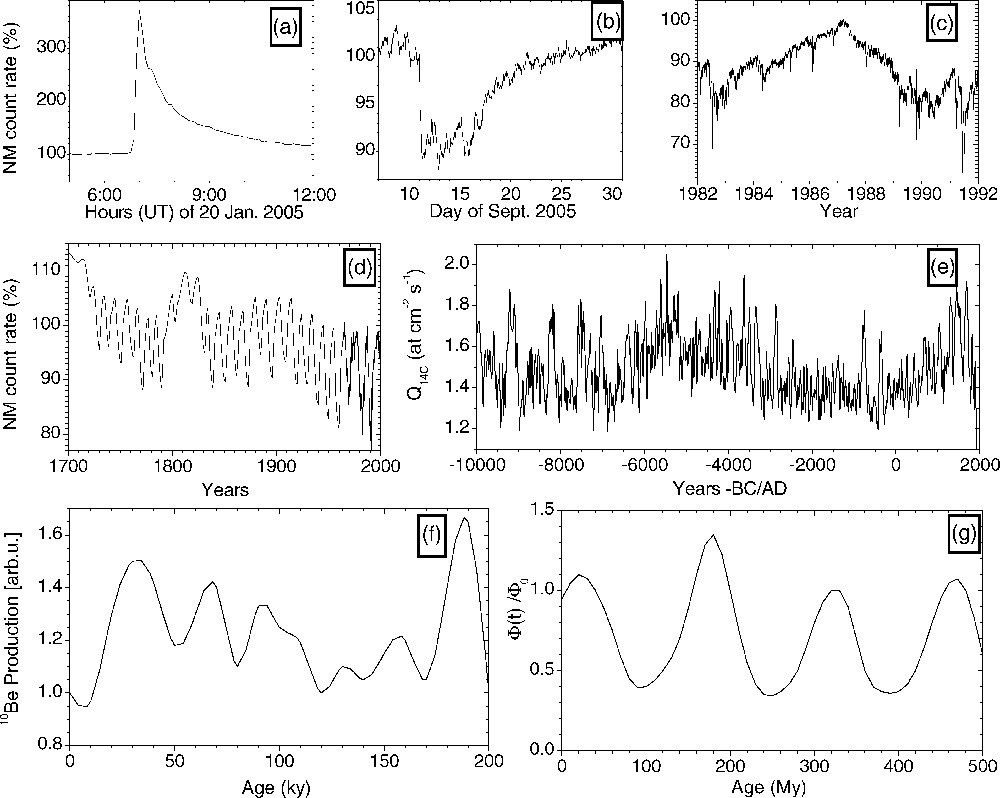
Cosmic ray variability on different time scales: (a) Event of solar cosmic rays (GLE of 20/01/2005 measured by Oulu NM); (b) Forbush decrease (the event of Sept. 2005 measured by Oulu NM); (c) Solar cycle modulation of cosmic rays as recorded by Oulu NM; (d) Centennial variability (reconstruction of a polar NM count rate [82]); (e) Multi-millennium variability (atmospheric production rate of computed for the Holocene in [79]); (f) Long-term variability (normalized production measured in deep marine sediments [26]); (g) Geological scales (normalized CR flux proposed in [64]). Note the different time scales.
Variabilité du rayonnement cosmique à différentes échelles de temps. (a) Événement GLE du 20 janvier 2005 du rayonnement cosmique solaire, mesuré par Oulu NM ; (b) diminution Forbush (événement de septembre 2005 mesuré par Oulu NM) ; (c) modulation du cycle solaire du rayonnement cosmique enregistré par Oulu NM ; (d) variabilité centenaire (reconstitution du taux de comptage polaire NM[82]; (e) variabilité multimillénaire (taux de production atmosphérique de 14C estimé pour l'Holocène[79]; (f) variabilité à long terme (production de 10Be normalisé, mesurée dans les sédiments marins profonds[26]) ; (g) échelles géologiques (flux Φ normalisé CR[64]; noter les différentes échelles de temps).
Thus, the CR flux varies on all time scales, from sporadic changes due to solar eruptive and transient phenomena on minutes-to-days time scale, to variations due to geomagnetic or even galactic changes on kyr and Myr scale. In subsequent sections, we will compare these CR changes with the known climatic reconstruction on different time scales and discuss their theoretical grounds.
3 Climate variability and its relation to cosmic rays
3.1 Daily/weekly time scales
In addition to regular daily variations (at the level of about 1%) of the CR flux due to the local CR anisotropy, strong transient phenomena sometimes occur (Figs. 1(a) and (b)) that either enhance or reduce the CR flux for hours – days. These phenomena are sporadic and their net contribution is tiny [85], but on the other hand, their rare occurrence and strong momentary effect give a good opportunity for case studies of solar–terrestrial relations. Many statistical studies have been performed, looking for a relation between such sporadic CR variations and the atmospheric/climatic parameters. For example, it was suggested that a small reduction of the mean cloud cover at high latitudes (N) [58], as well as changes in the atmospheric transparency at high latitudes (N) [60], could be associated with Forbush decreases. Another superposed epoch analysis [40,41] of ISCCP data suggests that the cloud cover is reduced at all latitudes over regions with relatively higher cloud cover and probably increased over ocean surfaces at middle and lower latitudes in regions with thinner cloud, in association with CR flux reduction. However, a recent thorough analysis [10] has not shown any statistically significant changes in the ISCCP-based cloud cover in correlation with six largest Forbush decreases. Also, formation of an aerosol layer, observed in stratosphere, has been associated with some strong solar particle events [50,51,67]. On the other hand, as suggested in [75,77], CR may primarily affect vorticity in polar/subpolar regions during the cold season, leading either to a reduction [77] after Forbush decreases or to an increase [89] during/after solar particle events. Also, a lowering of the tropospheric pressure layers has been reported in association with solar energetic particle events in North Atlantic [90].
In summary, although a global one-to-one relation between the cloud cover and CR variations is not confirmed [10] on the daily scale, much evidence implies that CR changes may affect the process of cyclogenesis through changing transparency and pressure, particularly in the North Atlantic during cold seasons.
3.2 Interannual variability
The 11-year solar cycle is the most pronounced variation of the CR flux on the time scale of decades, when there exist reliable satellite-based data on cloud cover. Accordingly, the CR–climate relations are mostly studied on the interannual time scale. A reported decadal cycle in the global coverage of low clouds was attributed to the CR flux variations [71,73]. This result initiated a dispute in the literature covering both arguments, pro [11,39,46–48] and con [30,43,44,70,78]. However, as mentioned by Gierens and Ponater [30], the globally averaged cloud data hardly shows a consistent correlation with the CR flux. On the other hand, a highly significant persistent correlation between the low cloud cover and cosmic rays was found in some geographical regions during the last 22 years [56,83,92]. Note, however, that low clouds can be partly masked by high clouds in the satellite data set, leading to spurious correlations in some regions [55,86]. Other mechanisms may also affect the cloud formation, especially at higher altitudes, e.g., via the global current system [74] or UV heating [33], which work in anti-phase with CR variations, as well as volcanos or ENSO variability. A recent thorough study [92,93] explored all these possibilities and has shown that a statistically significant direct correlation between low clouds and CR exists in a few climate-defining regions in mid-high latitudes (see Fig. 2), the largest being the North Atlantic+Europe and the South Atlantic. This pattern roughly corresponds to the model result [38] which predicts that the strongest response of the aerosol concentration to changes in CRII is expected at mid-to-high latitudes over oceans. An example of the temporal variations of low clouds and CRII in Europe is shown in Fig. 3, depicting the striking similarity between the two quantities. However, almost no correlation is observed outside these regions.

Geographical distribution of the partial correlation between low cloud amount [36] and solar indices, CRII and UV-index for 1984–2004 (see [92] for details). Only areas with statistically significant (at the 90 % level) correlation are hatched. The horizontal and vertical hatching depict areas where the correlation with UVI and CRII dominates, respectively (red/blue colour stands for positive/negative correlation). Inclined hatching indicates that the observed correlation may be spuriously induced by other types of clouds (middle or high clouds).
Distribution géographique de la corrélation partielle entre proportion de nuages bas [36] et indices solaires, CRII et UV pour la période 1984–2004 (voir [92] pour les détails). Seules les zones à corrélation statistiquement significative (au niveau 90%) sont hachurées. Les hachures horizontales et verticales correspondent aux zones pour lesquelles la corrélation avec UVI et CRII est dominante (respectivement rouge/bleu pour la corrélation positive/négative). Les hachures obliques indiquent que la corrélation observée peut être faussement induite par d'autres types de nuages (nuages de moyenne ou de haute altitude).
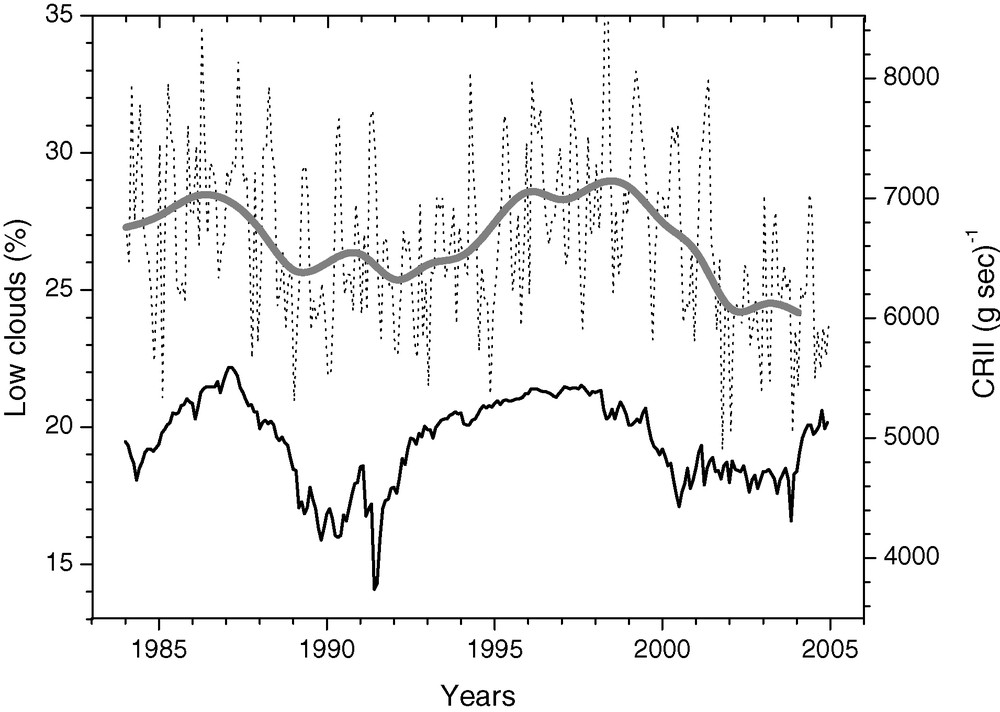
Variability of the low cloud amount [36] (upper curves, left axis) and cosmic ray-induced ionization at about 3-km altitude [80] (lower curve, right axis), averaged over the West European region (30–70N, 10W–15E). The grey curve depicts the annual mean of the cloud data.
Variabilité de la quantité de nuages bas [36] (courbes du haut, axe de gauche) et du rayonnement cosmique induit par ionisation à environ 3 km d'altitude [80] (courbe du bas, axe de droite), moyennée sur l'Europe occidentale (30–70° N, 10° W–15°E). La courbe grise correspond à la moyenne annuelle des données sur les nuages.
In summary, the link between low clouds and CR is statistically significant on the interannual time scale in some limited geographical regions since 1984. Use of global or even zonally averaged data may be misleading.
3.3 Centennial to millennial time scales
A detailed study [54] of a possible link between CR and cloudiness was performed by using the results of sunshine observations during the 20th century. Although the data is not easy to interpret and analyze, it suggests that a link between the total cloud cover and CR is unlikely. On the other hand, the data is in general agreement with the hypothesis of a link between low clouds and CR.
Numerous studies (see reviews [16,81,91]) confirm a relation between solar/CR activity and different indices of climate behaviour (e.g., or drift ice debris [8]) during the Holocene.
For example, there is an apparent agreement between the grand minima of reduced solar activity and cold/wet climate episodes (see [91] for review). Fig. 4 confronts a physics-based reconstruction of the solar activity from the data [69,87] with periods of identified sudden climatic shifts to cold/wet conditions in Europe during the last 7000 years [7,49]. One can see that they tend to appear during the grand minima periods. We note that 12 out of 14 climate shifts after 5000 BC occurred during grand minima of solar activity identified by Usoskin et al. [87], giving a 86% hit rate. On the other hand, only 3 (ca. 4200 BC, 700 AD and 1050 AD) out of 15 grand minima identified for the same period are not accompanied by climate shifts (80% hit rate). This also suggests, with high significance, a close relation between shifts of the climate type and solar activity (cosmic rays), at least in the European region. Although such studies cannot distinguish whether the primary effect is via CR or solar irradiation (e.g., [17]), an analysis of the geomagnetic field variation may help in disentangling the mechanisms (see a subsequent section).
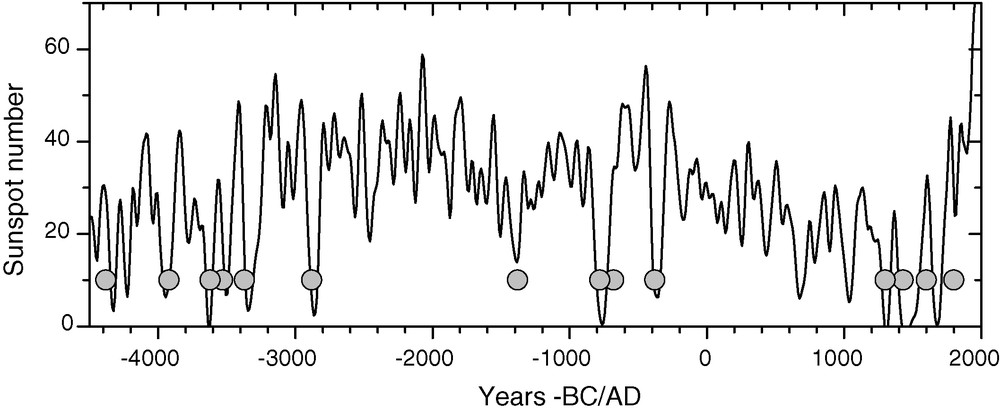
Sunspot number reconstruction (line – [87]) as well as known climate shifts to cold/wet conditions (dots – [7,49,88]) for the last 6500 years.
Reconstitution du nombre de taches solaires (ligne –[87]) ainsi que des dérives climatiques connues, dans les conditions de climat froid/humide (points –[7,49,88]) pour les 6500 dernières années.
In summary, there is a set of evidence that the solar variability affects the climate changes on centennial–millennial time scales, but it is hard to distinguish the role of cosmic rays, and the exact mechanisms need to be resolved.
3.4 Effect of the geomagnetic field
Although CR and solar activity variations are closely related, the former are also affected by another factor, the geomagnetic field, which changes independently of the solar variability, and with a different characteristic time scale. Therefore, a simultaneous study of climate, solar and geomagnetic proxies would help in disentangling the CR and solar irradiance effects on climate.
A detailed study [84] has revealed a weak but persistent correlation between the Northern Hemisphere temperature and the geomagnetic field intensity during the last millennium, implying that CR play a role in climate variations, although this correlation is not strong [94]. It is suggested that centennial climatic changes could be triggered by enhanced secular variation of the geomagnetic field [5,18], in particular by archeeomagnetic jerks [28,29]. It was found that on the multi-millennial time scale, periods of geomagnetic field reversal roughly correspond to cold episodes of the palaeoclimatic reconstructions [12–14,21,96].
It is important to note that not only the changes of the geomagnetic dipole strength affect CRII, but also fast migration of the geomagnetic axis can lead to dramatic changes in different regions [42]. Accordingly, studying regional data may shed new light on the possible mechanisms.
In summary, the relation between the geomagnetic field changes and climatic variations provides an evidence favouring a possible CR influence on climate.
3.5 Mega-year time scales
On the geological time scale (longer than a million years), CR variations are assumed to be determined either by the geomagnetic field changes or by the changing local galactic environment. For example, if the Solar System enters a galactic spiral arm during its evolution, a greatly enhanced flux of CR would be impinging on Earth [9,61]. Although the path of the Solar System relative to the Milky Way spiral arms is poorly known, CR variability on the geologal time scale (see Fig. 1g)) can be guessed [63–65] to depict a similarity with palaeoclimatic reconstructions [62,63]. This result has been both disputed [59] and supported [31] by other researchers. Note that, even if the correlation itself was real, its interpretation is not straightforward since it is based on an assumption that the climate and its drivers remain unchanged throughout millions of years. Also, there are concurrent effects which may lead to similar relations. E.g., a large amount of galactic dust would be loaded into the Earth’s atmosphere in galactic spiral arms, leading to enhanced opaqueness of the atmosphere and thus to a cool climate [57], in concurrence with the CR effect. Interestingly, the rate of geomagnetic field reversals also varies on the geological time scale [23,96] quite synchronously with the climatic variations. This itself modulates the CR flux impinging on the Earth also in synchronization with the CR effect due to spiral arm crossing. Moreover, the type of climate, seismic activity and physical-chemical properties of the atmosphere were essentially different on the geological time scale, and it is hardly possible to distinguish the role of indirect mechanisms.
In summary, although there is an indication of the climate changes synchronously with the putative CR flux on Myr time scales, these results remain speculative.
4 Possible mechanisms
Although the amount of energy deposited by energetic CR in the atmosphere is very small (about 10−5 W/m2), they affect, via their ionizing effect, the chemical-physical conditions of the atmosphere. This, in turn, can influence the ability of the terrestrial system to absorb/trap/reflect solar radiation through, e.g., the cloud cover [52,71]. Therefore, with a negligible amount of its own energy, CR may greatly modify the amount of solar radiation received by the planet, and change its radiation budget, thus being a very effective trigger/amplifier of the solar variability. Note that clouds, whose formation can be supposedly modulated by CR, lead to both the trapping of outgoing long-wave radiation and reflection of the incoming solar radiation. However, the net effect of these concurrent processes is cooling (i.e. more clouds correspond to a cooler climate).
Essential progress has been achieved recently in the development of a precise physical model of the CR-induced nucleonic-electromagnetic cascade in the atmosphere, leading to a full understanding of the ionizing effect of cosmic rays [19,53,80,83]. However, a detailed mechanism of how CRII can affect cloud formation is still not well understood, and two main hypotheses are discussed in the literature (see, e.g., reviews in [35,72,76]).
One hypothesis is the most ‘apparent’ and exploits the ionizing effect of CR [4,20,38,46,97,98]. It assumes that ions produced by CR rapidly interact with molecules in the atmosphere and are converted into complex cluster ions, which may grow by ion–ion recombination or ion–aerosol attachment and thus affect the number of aerosols acting as cloud condensation nuclei. A simple analogy for this mechanism is a cloud chamber (also known as the Wilson chamber), where charged particles leave visible tracks of droplets condensated along their paths. However, this analogy is not entirely valid, as the cloud chamber uses heavily supersaturated vapour and in this sense is different from the real atmospheric conditions, where vapour is not (or is marginally) saturated and there are other admixtures, like aerosols, also affecting the condensation process. The presence of aerosols, especially gaseous H2SO4, in the atmosphere is important to allow new particle growth under CRII [4,37]. The role of ions as nucleation agents was first established for the stratosphere and mesopause [2,3] and later [4,22] confirmed for the cold upper troposphere. However, this mechanism can work in different ways, depending on the ambient conditions [37,38]. This may lead to the geographical pattern of the observed CR-cloud relation (see Section 3.2).
An alternative hypothesis [74,75] is based on the assumption that cloud formation is affected by the atmospheric electric field via precipitation and ice formation in super-cooled water. On one hand, the electric field is influenced by CR since CRII controls the atmospheric conductivity. On the other hand, the same processes which modulate CR (interplanetary magnetic field, solar wind, interplanetary shocks, etc.) also greatly affect the planet’s global current system and thus the electric fields. Cosmic rays only play a partial role in this mechanism and work in concurrence with the direct solar activity.
There is also a possibility that solar variability may affect cloud formation even without the direct influence of CR, i.e. via circulation changes due to stratospheric heating caused by the ozone absorption of solar UV radiation [32,33]. In particular, such changes may lead to changes in winter circulation patterns that affect middle-latitude storm tracks.
All these mechanisms can work in different conditions (e.g., at different altitudes or geographical regions – see, e.g., [37,92]) or in concurrence, and it is difficult to distinguish between them. Unfortunately, none of these possible mechanisms has a solid support of a quantitative physical model. Despite the significant progress in modelling the aerosol nucleation [15,38], the predictions of current atmospheric nucleation models are quite uncertain, since the link between micro- and macro-physics is still missing. On the other hand, all experimental evidences, as described above, are based on statistical, often marginally significant, studies, lacking for a clear case study.
A new step has been made recently in modelling the chemistry of the lower atmosphere in laboratory conditions – the SKY experiment conducted at the Danish National Space Center [72] (an enlarged and improved version of this experiment is under construction at CERN [11].) The result of this experiment (Fig. 5) indicates that enhanced ionization of air in realistic conditions facilitates the formation of stable, ultra-small clusters of sulphuric acid and water molecules. This result is preliminary and waits to be confirmed. Even though further steps from micro- (molecular clusters) to macro- (cloud condensation nuclei) physics remain unresolved, it provides the first laboratory evidence that ionization can affect physical-chemical properties of the atmosphere. This complements the existing statistical relations and theoretical models linking ultra-fine aerosols and CRII [4,15,37,51] and confirms experimentally the first step of CRII-related mechanism linking CR to the cloud formation.

The results of SKY experiment [72] – a relation between ion density and aerosol nucleation. Asterisks depict the experimental data, while the line corresponds to a best-fit linear relation.
Résultats de l'expérience SKY [72] – une relation entre densité ionique et nucléation d'aérosols. Les astérisques correspondent aux données expérimentales, tandis que la ligne correspond à la relation linéaire la plus appropriée.
5 Conclusions
We have reviewed the experimental evidence and theoretical models relating cosmic ray variations to the terrestrial climate changes.
On a short time scale of a few days, there exists much evidence that CR changes may affect the process of cyclogenesis via the changing transparency and pressure, particularly in the North Atlantic during cold seasons. Although each individual piece of evidence is barely significant, in aggregate, they suggest that the relation can be real.
A link between low clouds and CR appears statistically significant on the interannual time scale since 1984 in limited geographical regions, the largest being North Atlantic + Europe and South Atlantic. We note that many reconstructions of the past climate are based on European data, where the CR–cloud relation is the most pronounced. Extension of this relation to the global scale may be misleading.
A relation between the geomagnetic field changes and climatic variations provides evidence favouring the possible CR influence on climate. A study of regional climate variations in relation to the geomagnetic dipole axis migration over the last millennium is also promising.
There is an indication of the climate changes synchronously with the CR flux on Myr time scales, but this result is not straightforward to interpret. Large uncertainties make it only indicative.
Essential progress has been recently achieved in theoretical modelling of both ionizing effect of CR and physical mechanisms relating CRII to cloud variations, but the link between micro- and macro-physics is still missing. A new experimental evidence, obtained by the SKY experiment team, confirm that enhanced ionization notably facilitates the production of small ion clusters in realistic atmospheric conditions.
In conclusion, a CR–climate link seems to be a plausible climate driver, as supported by the bulk of statistical studies and existing theoretical models. However, further studies, in particular a clear case study as well as improved model development, are foreseen to improve our understanding of the link between cosmic rays and the climate on Earth.
Acknowledgements
Support from the Academy of Finland and the Finnish Academy of Science and Letters Vilho, Yrjö and Kalle Väisälä Foundation is gratefully acknowledged. GAK acknowledges also the Program of Presidium RAS N16-3-5.4. E. Usoskina is acknowledged for suggestions on improving the manuscript style.