1 Introduction
Extreme natural disturbances – floods, fires, droughts, hurricanes and volcanism – fundamentally alter landscapes, leaving biophysical legacies that persist for centuries. Surprisingly, however, ‘extreme’ disturbances – though costly to human life and infrastructure – often are not ecologically catastrophic [62,63]. In rivers, for example, extreme floods and droughts are essential for maintaining common biological and physical characteristics, including ecological vitality. We use the term ’vitality’ here to reflect the integrity, health, resilience, and productivity underpinning the ability of an ecological system to thrive.
The importance of flow variability is well recognized by ecologists and water resource managers. Nevertheless, water is a valuable commodity as well as a destructive force. Human society requires water for life while seeking protection from floods and droughts. As a consequence, many rivers have been heavily modified to enable water managers to control flows to meet human needs (e.g., industry, agriculture, development of historical floodplains) while dampening or eliminating normal floods and droughts [10]. By trapping floods rather than conveying them downstream, many regulated rivers retain little of their original flow variability [52]. Human control of river flows is now nearly ubiquitous [35,71]. Today, rivers are managed to meet multiple human demands (e.g., steady and dependable water supplies, flood control facilities to protect populated areas). These factors severely constrain the flow variability which is required to meet ecological demands [32,55].
Although human manipulation of river flows provides many societal benefits, it also degrades and eliminates valuable ecosystem services [58]. River vitality and services (e.g., water purification, recreation) deteriorate when the natural routing of water, sediments and organic materials is substantially modified [51,57]. Remedies to these human-caused problems are often costly or difficult to implement. In the absence of manipulation, floods and droughts interact with the underlying geology to shape the river's physical and chemical templates; sites upon which the biotic communities develop over long time scales [23,31,33,34,73] (Fig. 1). Significantly modifying or depriving the system of flow extremes upsets patterns in material transport, resource availability, plant mortality and succession, and the composition of biological communities.

Schematic representation of the relationship among hydrogeomorphic processes, habitat dynamics, and riverine communities. Lithotopographic units are areas with similar topography and geology, and within which similar suites of geomorphic processes occur. Modified from Montgomery [28] and from Naiman et al. [31].
Fig. 1. Représentation schématique de la relation entre processus hydrogéomorphiques, dynamique de l’habitat, communautés fluviales. Les unités lithotopographiques sont des zones à topographie et géologie semblables, et à l’intérieur desquelles des suites similaires de processus géomorphiques apparaissent. Modifié d’après Montgomery [28] et Naiman et al. [31].
Our objective is to illustrate how variability in flow and water temperature shapes the biophysical attributes and functioning of river systems. We explain the ecological rationale for sustaining flow variability. We examine case studies from rivers in two contrasting climate regions – a semi-arid savanna river in South Africa and a temperate rainforest river in North America – that illustrate connections between flow variability, large wood, and the development of river-specific ecological characteristics. We conclude by exploring the importance of variability in establishing environmental flows for rivers – flows needed to sustain ecological systems. This latter subject is especially important in light of ongoing climate changes and the widespread need to balance environmental and human demands for water.
2 The ecological rationale for sustaining flow variability
2.1 Defining flow variability
The natural flow-regime paradigm postulates that the structure and function of riverine ecosystems, and the adaptations of their constituent riparian and aquatic species, are dictated by patterns of temporal variation in river flows [51]. In ecological terms, the primary components of a flow regime are the magnitude, frequency, seasonal timing, predictability, duration and rate of change of flow conditions (Table 1). From an evolutionary perspective, extreme events (floods and droughts) exert primary selective pressure for adaptation because they often represent sources of mortality [23,49].
Common terms used to describe variability in river flows
Tableau 1 Termes communs utilisés pour décrire la variabilité dans les débits fluviaux
Magnitude: the amount of water moving past a fixed location per unit time. The larger (or smaller) the magnitude of a flood (or drought), the greater the expected physical impact. |
Frequency: the number of events of a given magnitude per time interval (e.g., per year). For a given river or stream, frequency is typically related inversely to magnitude. |
Duration: the period of time associated with a particular flow event. Typically expressed in terms of number of days a flood or drought lasts. |
Timing: the date during the year that flood or drought occurs, often derived from long-term flow records. |
Predictability: the degree to which flood or drought events are autocorrelated temporally, typically on an annual cycle. Predictable events also might be correlated with other environmental signals (e.g., rainfall events, seasonal thermal extremes, photoperiod, sudden increases or decreases in flow). |
Substantial spatial variability exists in natural flow characteristics related to climate, geology and topography. Examination of flow gauge data for 806 free-flowing streams and small rivers across the USA by Poff [48] showed natural flow-regime parameter values ranging from 0.62 to 0.91 for flood frequency (overbank events yr−1), 0.44 to 0.96 for flood predictability (proportion of floods falling in a common 60-day period), 2.3 to 9.9 days for average flood duration, and 0.45 to 0.81 for drought predictability (proportion of droughts falling in a common 60-day period). Snowmelt-dominated streams in the Rocky Mountains have the highest predictability of seasonal flood timing (∼0.95; scale of 0 to 1), relatively high low-flow timing (∼0.75), and relatively low flood frequency (∼0.60). By contrast, some streams in the Great Plains of the USA have unpredictable flood (∼0.45) and low-flow timing (∼0.50), and very high flood frequency (∼0.90). Subsequent comparisons of river systems across the USA also illustrate substantial within- and between-continent variability in natural flow characteristics [52].
2.2 Ecological adaptations to flow regimes
The physical characteristics of flow variability have strong ecological consequences at local to regional scales, and at time intervals ranging from days (ecological effects) to millennia (evolutionary effects). Even though not all flow regimes favor the evolution of traits that enable flood or drought survival, life-history theory predicts that the magnitude, frequency and predictability of flow events can affect how organisms evolve or fail to evolve (see [23]). The theoretical predictions suggest that the timing of life-history events, such as age at reproduction, is optimized with respect to mortality-causing events, such as timing of flood or drought events [22]. When extreme flows are frequent, large in magnitude and predictable, selection favors life histories synchronized to avoid or exploit extreme flow events (the evolutionary zone). By contrast, extreme flows that are frequent and large in magnitude but unpredictable have low selection strength for life history timing, even though they might inflict high mortality on populations (the ecological zone). Although no optimal strategy for life-history timing can evolve to avoid unpredictables or droughts, bet-hedging strategies might evolve in this case (see [23]). For example, in the historically harsh arid environments of the Lower Colorado River basin (USA), evidence suggests that the life-history strategies of native fishes are positioned along an evolutionary bet-hedging axis representing a trade-off between the onset of reproduction and fecundity [37]. The lifespan of an organism might also affect, and ostensibly reflects, selection strength because disturbance frequency, and thus selection strength, occurs relative to the lifespan of an organism (e.g., a long-lived cottonwood tree experiences annual snowmelt-driven floods many times during a lifetime, whereas a mayfly larvae in the same river system might never experience one).
It has been well-demonstrated that the life history traits of individual species, and the emergent community characteristics, are strongly linked to annual variability in flow regimes (e.g., [17,50]). All parts of the annual hydrograph have consequences for riverine biota (Fig. 2). Four principles highlight important mechanisms linking flow variability and aquatic biodiversity, and serve to illustrate the generalized impacts of altered flow regimes [7]:
- • flow variability is a major determinant of physical habitat in streams, which in turn is a major determinant of biotic composition;
- • many aquatic and riparian species have evolved life history strategies that are responsive to the natural flow regimes;
- • maintenance of natural patterns of longitudinal and lateral connectivity is essential to the viability of populations of riverine species;
- • the success of non-native species in rivers is facilitated by the alteration of flow regimes, and the impacts are manifest across broad taxonomic groups including plants, invertebrates, and fish.
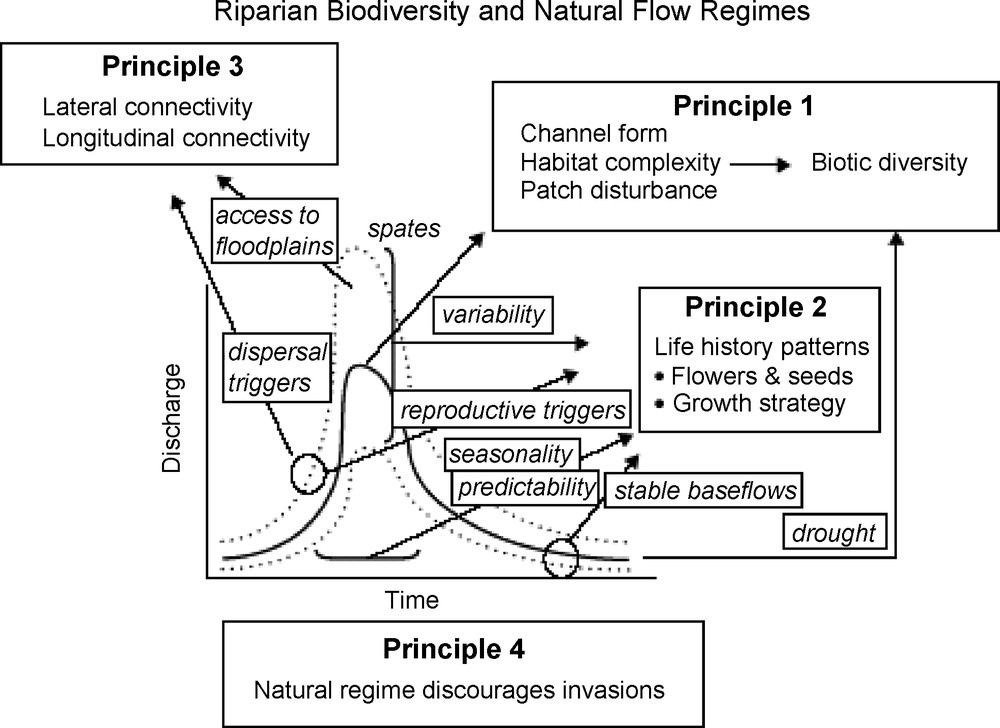
The natural flow regime influences riparian biodiversity via several interrelated mechanisms operating over different spatial and temporal scales. The relationship between biodiversity and the physical nature of the riparian habitat is driven primarily by large floods that shape channel form (Principle 1). However, droughts and base-flows also play roles by limiting overall habitat availability. Many features of the flow regime influence life history patterns, especially the seasonality and predictability of the pattern (Principle 2). Some flows trigger longitudinal dispersal of riparian organisms and other large events inundate otherwise disconnected floodplain habitats (Principle 3). The native biotas have evolved in response to the overall flow regime. Invasion by non-native species is more likely to succeed at the expense of native biota if the former are better adapted to modified flow regimes (Principle 4). Adapted from Bunn and Arthington [7].
Fig. 2. Le régime de débit naturel influence la biodiversité riveraine au travers de divers mécanismes étroitement liés, opérant sur différentes échelles spatiales et temporelles. La relation entre la biodiversité et la nature physique de l’habitat riverain provient en priorité des grandes crues qui façonnent la forme du chenal d‘écoulement (principe 1). Cependant, les sécheresses et les débits de base jouent aussi un rôle, en limitant les habitats disponibles. De nombreuses caractéristiques du régime d’écoulement influencent les modalités des cycles vitaux, en particulier la saisonnalité et la prédictibilité de ces modalités (principe 2). Certains débits entraînent une dispersion longitudinale des organismes riverains, tandis que d’autres, plus importants, rétablissent des connexions entre des habitats séparés de la plaine d’inondation (principe 3). Les espèces d’origine ont évolué en réponse au régime des écoulements en place. L’invasion par des espèces étrangères a plus de chance de réussir si ces espèces sont mieux adaptées que les espèces d’origine aux régimes d’écoulement modifiés (principe 4). Adapté d’après Bunn et Arthington [7].
2.3 Extreme variability in flow – droughts and floods
Uncommon flow levels – droughts and floods – occurring at decadal to century-scale intervals have long-term consequences for biota [60]. Whereas most investigations focus on extreme floods (see case studies below), droughts have equally important long-term effects through fundamental changes to biogeochemical processes and to plant and animal distributions [16]. This is well illustrated in semiarid South Africa where riparian communities are often subjected to multiyear droughts [33]. Normal wet periods are characterized by strong plant production in uplands and in riparian zones, organic litter of good quality, relatively light herbivory by vertebrates with abundant feces returning selective nutrients to the soils, and a general increase in N availability (Fig. 3). Excellent upland savanna conditions mean that herbivory is spread across the landscape rather than being concentrated along rivers or in riparian areas. However, savanna conditions deteriorate as drought severity becomes acute. Upland plant biomass and production decline and palatable plant species are over-grazed in riparian corridors. Concomitantly, litter quality and N availability are reduced, the incidence of severe fires in the riparian zone is more frequent, and terrestrial plant species extensively colonize the river corridor. The net result is a ‘terrestrialization’ of the riparian zone. It is characterized by a proliferation of successional pathways depending on fire severity, subsequent rainfall, sediment dynamics, and herbivory – and the ecological situation becomes even more complex when rains return (see savanna case study below). Additionally, there are many parallels in rivers with flood-control dams, which cause extended artificial droughts in the historic floodplain.

A conceptual model of the environmental responses of riparian corridors in the semiarid region of South Africa to increasing drought conditions. In effect, the riparian system becomes more and more terrestrial as species and processes normally associated with the savanna uplands move closer to the river (from Naiman et al. [33]).
Fig. 3. Modèle conceptuel des réponses des corridors riverains aux conditions de sécheresse croissante dans la zone semi-aride d’Afrique du Sud. En fait, le système riverain devient de plus en plus terrestre, lorsque les espèces et les processus normalement associés à des points hauts recouverts de savane sont plus proches de la rivière (d’après Naiman et al. [33].).
2.4 Flow variability and water temperature
Water temperature is a fundamental ecological characteristic – with special significance for maintaining a thriving riverine biota – that is tightly linked to flow patterns. Water temperature is defined by the amount of heat energy entering a stream (which is primarily influenced by climate, channel morphology, groundwater/hyporheic influences, and riparian canopy condition) and the volume of water flowing in the stream channel [54]. Therefore, variability in flow regimes affects stream thermal regimes by influencing the timing or magnitude of heat energy delivered to the stream channel (i.e., heat load) or modifying the amount of water (i.e., discharge) that influences the assimilative heat capacity of a stream.
The ecological significance of water temperature in riverine ecosystems is widely recognized. Water temperature directly influences metabolic rates, overall physiology, and life-history traits of aquatic species and helps determine rates of important ecological processes such as nutrient cycling and productivity [24]. Most freshwater organisms are ectotherms, utilizing a diverse array of thermal habitats to meet their specific temperature requirements for survival, growth and reproduction. They respond to the entire thermal regime, which is a composite of patterns of absolute temperatures, diel and seasonal amplitudes, and rates of change; all superimposed upon other related environmental components such as photoperiod [74]. Temporal predictability of a river's thermal regime influences many population parameters of aquatic insects, including egg and larval diapause, embryonic development, larval growth and metabolism [70]. The natural thermal regime of a river also provides temperature cues stimulating fish spawning behavior and reproduction as well as insect emergence and egg hatching.
During drought, water temperatures depend on degree of insolation, substrate type, ground- or rainwater inflows, wind and vegetation cover [16]. Resistance of insect and fish populations to drought disturbance hinges, in large part, on adaptations of species and the spatiotemporal arrangement of refugia from extremes in temperatures and in dissolved oxygen [6,26]. During extended drought, the distribution of refugia and the frequency and timing of severe temperature episodes play a crucial role in structuring biological communities. Extreme temperatures alter fish physiology and behavior, change competitive interactions, pathogen resistance, and even result in death. Nevertheless, over long time scales, stream populations and communities should be resilient with most species recolonising rewetted streams in days to years, depending upon physiochemical tolerances, distance to source of colonists and extent of drying [18]. In other words, the distribution of the population must exceed the spatial extent of the drought or they must persist in refugia. In essence, communities living in systems with recurrent drought are generally adapted to recover relatively quickly from extreme temperature excursions [49].
3 Flow variability and system vitality: case studies
3.1 Floods, droughts and the origins of large wood in a semi-arid river
The flow regimes of arid and semi-arid river systems are highly variable because climatic variability, chiefly rainfall or lack thereof, leads to episodic flooding and prolonged drought [59,72]. For example, the Sabie River, South Africa, experienced a large flood (approximately 100-yr return interval) in February 2000 [13], during which most riparian trees and shrubs were toppled or destroyed (Fig. 4). This created approximately 200,000 wood piles along a 105 km stretch of river within Kruger National Park [44]. Piles occupied 2–11% of the riverine landscape [39]. Wood debris was scarce or absent from most of the riparian corridor prior to the flood [39]. Subsequently, it became apparent that the flood was a primary event driving the long-term ecological characteristics through the conversion of the riparian trees to woody material in the river corridor. While infrequent, events similar to this appear to shape the structure of riparian forests for decades to centuries [63].

Oblique aerial view of the Sabie River, Kruger National Park. (a) Pre-flood where there were extensive areas of riparian forest in the macrochannel. (b) Post-flood after a large proportion of the forest had been destroyed. (Photo: F. Venter, Kruger National Park, South Africa.)
Fig. 4. Vue aérienne oblique de la rivière Sabie, Parc national Kruger. (a) Avant la crue, lorsqu’on observe de vastes zones de forêt riveraine dans le chenal. (b) Après la crue, lorsqu’une grande proportion de la forêt a été détruite. (Photo : F. Venter, Parc national Kruger, Afrique du Sud.).
The wood deposited in the Sabie River after the 2000 flood reflected recent terrestrialization of the riparian zone due to a decadal long drought, which allowed terrestrial trees to colonize the channel floor [59,65]. A previous flood of comparable size on the Sabie River occurred in 1925 [13] and there is some evidence that flood established conditions for a significant recruitment of some important riparian trees. Prior to the 2000 flood the age-class distribution of the large riparian tree Ficus sycamorus (Sycamore fig) indicated that there had been little recruitment in recent years and possibly a large episodic recruitment event occurred approximately 70 years previous [68]. This suggests that many of the mature trees present in 2000 were likely to have recruited from the 1925 flood. Wood piles are important for the regeneration of riparian trees such as F. sycamorus, as seedlings of this species establish preferentially in wood piles after floods [41] (Fig. 5).
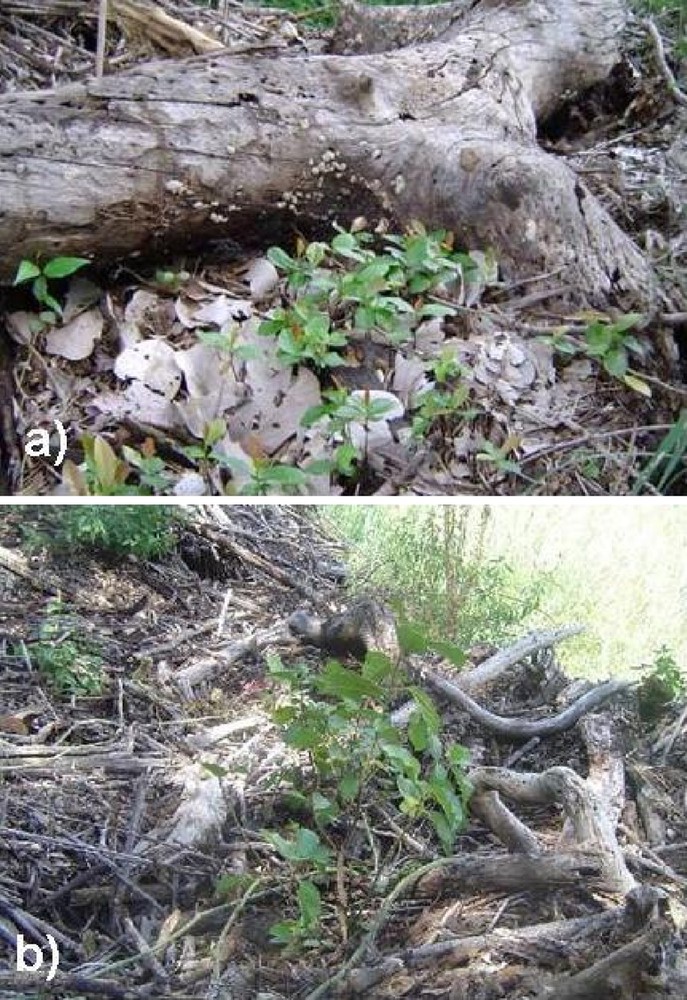
Development of tree seedlings in large wood deposited after flooding on the Sabie River in February 2000. (a) Diospyros. mespiliformis (jackal berry). (b) Ficus sycamorus (Sycamore fig). (Photos: N. Pettit.)
Fig. 5. Développement de repousses d’arbres sur du bois de grande taille déposé par une crue de la rivière Sabie en février 2000. (a) Diospyros mespiliformis (baie de chacal). (b) Ficus sycamorus (sycomore fig). (Photos : N. Pettit.).
3.1.1 Influence of large wood (LW) on initial successional pathways
Conditions created by LW debris in the post-flood environment suggest that type, abundance and position of wood piles shape the overall pathways of vegetation succession. Initial establishment from seed after the 2000 flood was dominated by ruderals (particularly grasses and annual forbs) in the channel and riparian areas [40]. However, woody plant regeneration (in terms of number of species and cover) within piles was significantly higher than outside piles (Table 2). This was due mainly to resprouting of toppled trees with intact roots and living tissues (36% of LW surveyed), although there was some regeneration from seedlings. This allows for the rapid re-establishment of vegetative biomass in the early stages of post-flood riparian forest renewal, and nearly all are associated with LW piles.
Mean values for LWD piles and adjacent reference (non wood) plots in soil nutrient, soil physical properties and vegetation across all landscape positions and LWD pile types. Values are mean ± standard error from a sample size of 119 paired LWD and reference plots
Tableau 2 Valeurs moyennes des nutriments du sol, des propriétés physiques du sol et de la végétation dans des amoncellements de bois « LWD » et des parcelles de référence sans bois pour toutes positions dans le paysage et tous types d’amoncellements de bois « LWD ». Les valeurs sont des moyennes plus ou moins l’erreur standard, à partir d’une taille d’échantillonnage de 119 paires « LWD » et parcelles de référence
LWD pile | Reference plot | t calculateda | p a | |
Soil nutrients | ||||
Total N (%) | 0.097 ± 0.001 | 0.081 ± 0.001 | 3.59 | 0.005 |
Available P (μg/g) | 10.4 ± 0.7 | 6.9 ± 0.54 | – | < 0.001 |
K (mg/l) | 196.1 ± 11.7 | 122.5 ± 8.3 | < 0.001 | |
Total C (%) | 0.87 ± 0.04 | 0.64 ± 0.05 | 3.91 | < 0.001 |
C:N ratio | 8.69 ± 0.89 | 7.11 ± 0.56 | 6.24 | < 0.001 |
Soil physical | ||||
Silt & clay (%) | 22.5 ± 1.2 | 16.2 ± 1.28 | 5.87 | < 0.001 |
Temperature (C) | 27.7 ± 0.21 | 33.4 ± 1.41 | – | < 0.001 |
Soil moisture (%) | 10.6 ± 0.32 | 5.7 ± 0.43 | 9.18 | < 0.001 |
Vegetation | ||||
Foliage cover (%) | 62.5 ± 8.2 | 50.7 ± 4.7 | 2.28 | 0.025 |
No. species | 11.4 ± 0.4 | 10.8 ± 0.41 | 1.39 | 0.17 |
Cover woody spp (%) | 33.2 ± 17 | 9.2 ± 6 | 6.33 | < 0.001 |
No. woody spp | 3.8 ± 0.5 | 2.6 ± 0.4 | 5.41 | < 0.001 |
No. tree seedlings (ha−1)b | 19.9 ± 5 | 16.6 ± 4 | 1.89 | 0.12 |
a Paired t test.
b Mean proportion of area for each substrate was wood pile = 10.25 ± 4.6%; non-wood (open or vegetation) = 65.4 ± 6.2%.
Deposited LW acts as localized focal points for accumulating fine sediments, soil nutrients and soil moisture that, collectively, results in patchy resource availability [40]. Favorable microclimates (e.g., increased soil moisture, reduced summer soil temperatures) and soil nutrients (e.g., elevated levels of N, P, K) within LW piles remain evident for years afterward (Table 2). These beneficial and stable conditions within LW piles make them particularly important as refugia for plants in semi-arid environments with long dry seasons, high ambient temperatures and considerable climatic variability. Even slight enhancement of microclimates and nutrient availability significantly improves the survival of germinating seeds and developing seedlings, particularly during stressful periods of low moisture and high temperature.
Large wood piles are also fuel sources that may allow seasonal savanna fires to penetrate the riparian zone; thereby elevating the risk of high severity fires, greater fire frequency and longer fire residence times. Dead wood accumulated around standing trees creates a particularly acute fire hazard for living trees, even in relatively mild fires. Patchiness of the riparian landscape (heterogeneity) is increased by fire and the development of resource-rich patches is enhanced by the presence of LW piles within the burnt landscape [42,43] (Fig. 6). This is seen in patterns of soil nutrient distribution (soil P 49% higher in burnt wood patches after 24 months) and vegetation cover with vegetation sparse in intensively burnt areas such as where LW was deposited (58% reduction in burnt wood patches). Fire patchiness also enables the continued existence of fire-sensitive species in riparian zones by providing unburnt refugia.

Pathways of influence of wood accumulations of successional processes in riparian vegetation and landscape heterogeneity after a large flood on the Sabie River, Kruger National Park, South Africa.
Fig. 6. Influence des accumulations de bois sur le processus de succession de la végétation riveraine et l’hétérogénéité du paysage après une crue importante de la rivière Sabie, Parc national Kruger, Afrique du Sud.
3.1.2 Large wood and riparian heterogeneity in semi-arid rivers
LW creates focal points for regeneration after large infrequent floods. These focal points provide a blueprint for patterns of regeneration as well as contribute to the spatial heterogeneity and biocomplexity [40] (Fig. 7). Despite the relatively brief retention time, the ecological legacy of LW deposition in the Sabie River appears to be preserved for long periods, manifested in vegetation structure, depositional features, buried wood deposits and local fire impacts on surviving vegetation. In arid and semi-arid environments, the patchy environment is effective at trapping and retaining resources; thus using them efficiently [21]. The dynamics and spatial variability of material inputs (including LW) also contribute to heterogeneity and hence the resilience of riparian landscapes.
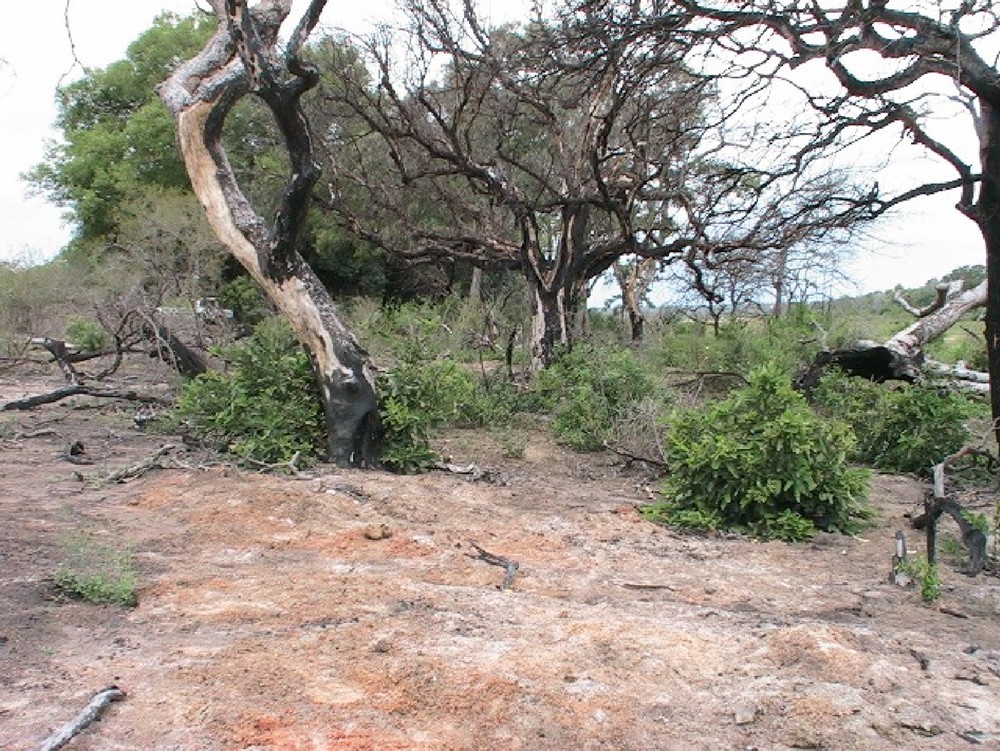
Burnt riparian area of the Sabie River. Note the ash bed from a burnt out large wood pile in the foreground, burnt trees resprouting from the base (mid-ground) and unburnt trees in the background. (Photo: R Naiman.)
Fig. 7. Aire riveraine incendiée de la rivière Sabie. A noter d’avant vers l’arrière: un lit de cendres, des arbres brûlés avec des repousses à partir de la base, des arbres non brûlés. (Photo : R. Naiman.).
3.2 Flow variability and the ecological characteristics of a temperate rainforest river
Flow variability supports temperate floodplain rivers in a manner similar to, but with slightly different mechanisms, from the semi-arid river described above. High flows capture LW by eroding stream banks and causing trees to fall into the channel; the resulting LW plays an important role equal to that in semi-arid rivers in shaping aquatic and riparian habitats. The ecological roles of LW in temperate regions are better quantified than in semi-arid regions but the functional roles are similar [12,45]. In-channel LW retains organic matter and moisture, forms pools, and promotes nutrient uptake [64]. Remnant logs function as habitat for terrestrial organisms and young plants. Many remnant logs contained in early-seral floodplain forests eventually re-enter the channel through ongoing bank erosion and continue to move downstream, creating habitat in numerous locations before disintegrating [19]. Some remain stable for decades or more and protect downstream forests from erosion for long periods [29]. The complexity of the river-floodplain system is supported by strong feedbacks and interactions between wood, pioneering vegetation, and sediments – with flow variability acting as a master variable.
Interactions between wood dynamics and flow magnitude have been widely demonstrated (e.g., [11,46,47,66,67]) and are commonly observed during floods. Though floods are ecologically important events, the physical characteristics of aquatic and riparian habitats in floodplain rivers reflect the consequences of the entire flow regime [51]. For example, channel migration in the Yampa River, Colorado (USA) is linked to the duration of flooding at 125% of bankfull discharge rather than simply the absolute flood magnitude [56]. In many other systems, such as the semi-arid river described above, large but infrequent floods erode riparian forests [38,47], generating massive quantities of LW [40,44]. In general, most lines of evidence indicate that high flows are important for recruiting, mobilizing and transporting wood in floodplain rivers [25], but transport also occurs during lesser flows [66]. Flow variability and resulting channel dynamics cause wood to ‘spiral’ downstream [19]. Wood, much like other particles, moves at irregular intervals that are, in part, flow dependent. At other times LW deposits at specific places in the channel until the return of necessary hydraulic conditions for movement. Instream supplies of wood therefore consist of a mixture of logs recently recruited to the channel from the surrounding forest as well as some from the distant past (e.g., [14]). The transient storage of LW in the floodplain likely buffers against climatic periods when delivery rates of new wood are low.
Observations from the Queets River in Olympic National Park, Washington USA, provide corroborating evidence that flow variability influences wood recruitment. Unlike the Sabie River, short duration catastrophic floods have not been observed on the Queets River, perhaps because of the relatively short period of record. However, rainfall-driven flow variability is substantial within seasons and among years. Reconstructions from sequences of historical air photos suggest that temporal variation in input rates of large trees from the erosion of mature terrace forests is positively related to the average magnitude of annual peak flow occurring in a given period of time (r2 = 0.92; p = 0.04; Fig. 8). Most LW originates where channel meanders undercut Sitka spruce (Picea sitchensis) and western hemlock (Tsuga heterophylla) in mature river terraces flanking the downstream edges of meander bends [19].
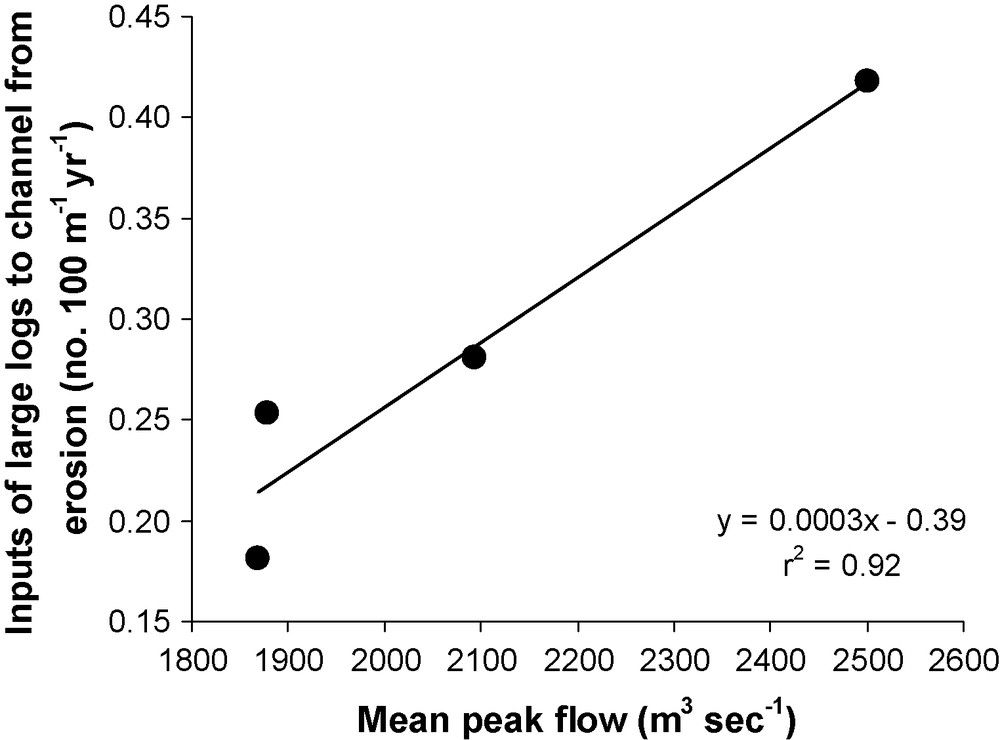
Relationship between annual input rate of large logs to the channel from erosion of mature forest terraces and mean peak flow rates over the entire Queets River mainstem in Olympic National Park, Washington, USA. Inputs were estimated from the area of forest eroded over an interval between aerial photographs (see Latterell and Naiman [19] for details). Mean peak flows were calculated from USGS records over each corresponding interval.
Fig. 8. Relation entre le taux d’apport annuel de grands troncs d’arbres au chenal d’écoulement, par érosion de terrasses couvertes de forêt et le pic moyen des débits sur le cours principal de l’ensemble de la rivière Queets du parc national Olympic, Washington, USA. Les apports ont été estimés à partir de la superficie de forêt érodée entre les photos aériennes (voir Latterell et Naiman [19] pour les détails). Les pics moyens des débits ont été calculés à partir des enregistrements USGS sur chaque intervalle correspondant.
Wood inputs resulting from flow variability aid in the initiation of a patchwork of forest regeneration on alluvial surfaces. As in the semi-arid rivers, successional processes reorganize the riparian plant community, gradually transforming bare alluvial deposits into forests over decades to centuries [8]. In the Queets River, pioneering vegetation is estimated to colonize over 95% of the channel area in a given year within a century – but often unsuccessfully as a result of ongoing flooding and channel movement [20] (Fig. 9). Flow variability interacts with the heterogeneous topography of the floodplain and the roughness created by LW and pioneering vegetation, influencing soil depth and stand structure. Ongoing interactions, combined with differences in site history and the time elapsed since patches were formed, cause patches to have contrasting vegetation age and size distribution, species composition, microclimate, soil characteristics and LW deposits [20,33,34,69]. Eventually, many patches are destroyed by channel movements. In the Queets River, half of the floodplain forest area is eroded within two decades after being formed, though four centuries are estimated to pass before half of the mature fluvial terraces are eroded [20]. Destruction of forest patches provides LW, sediment, and propagules (seeds, roots, and branch fragments) that initiate new patches downstream, where floodplain development begins again.

Variation in channel position in the Queets River, Washington, USA at 11 different years over a 63-year period: 1939, 1950, 1954, 1962, 1968, 1973, 1981, 1993, 1997, 2000, 2002. The scale of the image is roughly 2.5 km from left to right. (From Van Pelt et al. [69].)
Fig. 9. Variation de la position du chenal de la rivière Queets, Washington, USA pour 11 années différentes réparties sur une période de 63 ans : 1939, 1950, 1954, 1962, 1968, 1973, 1981, 1993, 1997, 2000, 2002. L’échelle de l’image est à peu près de 2,5 km de droite à gauche (d’après Van Pelt et al. [69]).
Ongoing patch destruction and periodic rearrangement of human-placed LW by floods is sometimes viewed as an undesirable outcome from the point of river restoration. This is understandable when engineered structures are intended to provide bank protection, or the river system is so constrained by human pressures that insufficient resources exist upstream to replace LW deposits and forest patches destroyed by the river. However, we argue the natural destruction and creation of some floodplain patches is actually needed to sustain the dynamic patch mosaic and may contribute to system resilience by stabilizing the availability of certain habitats at large spatial scales (e.g., [1,12,20,67]). For example, the distribution of pools [30] and pioneer bars [20] changes substantially over time in the Queets River, as patches are destroyed and re-created. These changes are fundamental characteristics of the system; the distribution of individual channel units or habitats fluctuates over time but the patterns of change are asynchronous among reaches, dampening the degree of change at the valley scale. As a result, they contribute to the resilience of riverine species that utilize these habitats for parts of their life cycle.
4 The challenge ahead: establishing environmental flows
The act of explicitly managing water flows for rivers – environmental flows – allows an appropriate volume and timing of water flows to support the ecological health and the livelihoods of dependent organisms, and in an ideal world this would include appropriate flow extremes (Fig. 10). The case studies illustrate the important influences of flow variability on fundamental, long-term ecological processes in natural rivers. A fundamental challenge for managers is to balance human and environmental needs for water [32]. Hundreds of approaches have been tried in the past few decades with varying degrees of success with respect to flow extremes [61]. At first blush, creative solutions abound. In practice, entrenched human demands on rivers create nearly insurmountable obstacles to achieving true balance. Establishing environmental flows promises to be effective because the imbalance is rectified by strategically re-aligning key human flow alterations rather than removing humans from the equation. In this way, the water management community may succeed in improving river vitality and permit society to enjoy the ecological goods and services that healthy rivers provide.
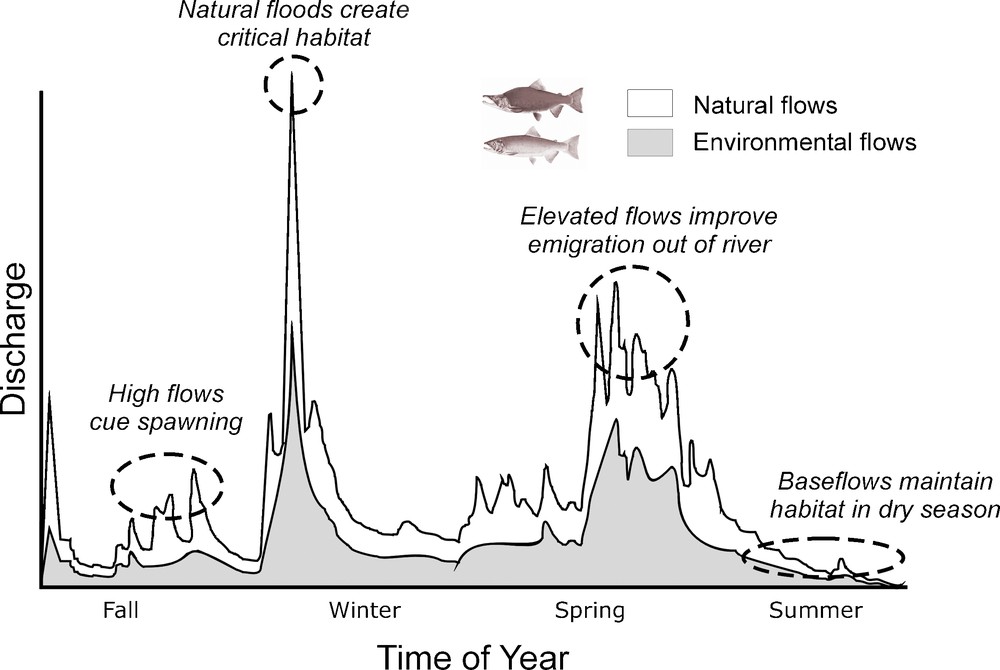
Comparative ability of natural and environmental flows to create and maintain U.S. Pacific salmon habitat and cue spawning. Shown is a hypothetical hydrograph that largely mimics temporal discharge patterns of the Trinity River, California (USA) and the life-history requirements of Chinook salmon (Oncorhynchus tshawytscha).
Fig. 10. Capacité des flux naturels et des flux environnementaux pour créer et maintenir l’habitat du saumon dans le Pacifique des États-Unis et donner le signal du frai. Le schéma correspond à un hydrographe hypothétique qui mime les débits de la rivière Trinity, Californie, États-Unis, et les exigences de vie du saumon chinook (Oncorhyncus tshawytscha).
The primary challenge to balancing human and environmental needs for water is that flow alterations, channel modifications, and land use changes are often inseparably linked, particularly in large rivers where flow modification is most pervasive. This practical constraint is unavoidable in many human-modified landscapes (e.g., agroecosystems to urbanized and industrial). Successful flow rehabilitation will often require simultaneous efforts to restore channel form and reconnect riparian areas, including floodplains, which in turn requires transformation of existing land uses. Land use transformation will require great care to avoid causing irreversible social hardship. Existing human demands of many large rivers are so great that striking a balance with the needs of the environment require a level of technical effort and social investment that is as yet unprecedented.
Knowledge gaps also present significant challenges to recovering flow variability in modified rivers. Natural differences in flow variability among rivers need to be better quantified. Empirical relationships between flow variation and ecological responses (e.g., protection of freshwater biodiversity and the maintenance of goods and services that rivers provide) remain elusive. Solving these knowledge gaps remains a great challenge for water managers and scientists alike [2]. The technical challenges and the practicalities of planning or implementing extreme flow events are not trivial.
Despite considerable progress in understanding how flow variability sustains river ecosystems there is, nevertheless, a growing temptation to ignore natural system complexity – especially the importance of flow variability – in favor of simplistic, static, environmental flow “rules” to resolve pressing freshwater issues. Such approaches are misguided and ultimately fail to sustain vitality in rivers. Water managers everywhere are increasingly required to provide reliable and affordable water supplies to a growing population while, at the same time, are expected to do so without degrading freshwater ecosystems [55,57,61]. Fortunately, this challenge is recognized, and many scientists and managers are working closely to develop a process (described below) that can be implemented at regional scales [2,53].
Determining and implementing effective environmental flow regimes for rivers acknowledges the need for appropriate variability in discharge [2,58] and temperatures [36]. Defining ‘appropriate variability’ will be increasingly difficult, because climate change renders the past an imperfect analogue of future conditions. Environmental flow guidelines must therefore explicitly incorporate likely consequences of climate change on the hydrology of managed systems to reduce the likelihood of future crises. Sustaining healthy freshwater ecosystems requires that adequate water flows – and associated temperatures – are maintained within the ecosystem while other human uses of water (e.g., urban, agricultural, power generation, flood control) are accommodated.
Generic approaches for determining environmental flow requirements are being developed. The most promising ones incorporate essential aspects of natural flow variability shared across particular classes of rivers that can be validated with empirical biological data and other information in a calibration process [2]. One approach under development is the “Ecological Limits of Hydrologic Alteration,” or ELOHA [53]. This method appears to satisfy several essential scientific requirements for successful application even though the social and political dimensions require further development. In addition to being grounded in mechanistically-based, flow-ecology linkages that are subject to empirical testing and validation, it is applicable:
- • at a regional scale;
- • across a spectrum of worldwide social, political and governance contexts, and useful regardless of the stage of water resource development and historical status of environmental flow protection;
- • across an array of flow alterations, from modified land use to river regulation by dams;
- • across a wide range of available data and scientific capacities.
There are four steps general scientific steps in ELOHA [53]. They are: (1) building a “hydrologic foundation” of stream flow time series for both baseline (undeveloped) and developed conditions, throughout the region of interest; (2) classifying rivers throughout the region into “river types,” using hydrologic time series that represent baseline conditions; (3) estimating the degree of hydrologic alteration that has occurred throughout the region, based on comparison between baseline and developed conditions, resulting in measures of hydrologic alteration; and, (4) developing flow-ecology response curves for each river type by associating increasing degrees of hydrologic alteration with increasing or decreasing ecological change.
The need for practical approaches to environmental flows, like ELOHA, is global. Despite hundreds of international treaties, conventions, and national and state policies and laws for ecosystem protection, the global degradation of freshwater biodiversity and environmental quality is ongoing, even accelerating [9,27]. Much of this degradation is a direct result of flow homogenization of the world's rivers by dams and by water withdrawals that undermine natural flow variability [10,52,71]. Nevertheless, it is recognized that flow regulation, land fragmentation and development are a suite of tightly interacting factors, often implemented simultaneously, making it difficult to assign cause and effect to one or the other. The issue becomes even more complex when one considers the ongoing and widespread changes to flow regimes from climate change [3,15] and the enormous efforts expended in river restoration that largely ignore the fundamental importance of flow variability [4].
Sustainable water resource management is constrained by three pervasive myths; that societal and environmental water demands always compete with one another; that technological solutions can solve all water resource management problems; and that environmental solutions to protect and maintain freshwater resources are more expensive and less dependable than technological solutions [5]. While conservation and good stewardship of water resources can go a long way toward meeting societal demands and values, new approaches to sustain ecosystem health and biodiversity in rivers and their associated systems can be well aligned with options for human use. These options can deliver a suite of ecosystem goods and services to society – but only if ecologically-appropriate flow variability is maintained.
Acknowledgements
We thank Henri Décamps and LeRoy Poff for constructive comments on early versions of the manuscript. Financial support for our portion of the research reported on here is from the Andrew W. Mellon Foundation, the U.S. National Science Foundation, and the U.S. Forest Service Pacific Northwest Research Station, and the Weyerhaeuser Company.