1 Introduction
Extreme climate events have a wide impact on society as well as on biophysical systems. They arise unfortunately and unpredictably in a stochastic way. Knowledge of extreme events is often rather fragmentary, and recorded experience is limited. Indeed, climate scientists do not really understand what causes extreme events, how they develop, and when and where they will occur [38]. Ecologists and managers aim at understanding direct impacts of extreme events and persistent after-effects, as well as the ability of ecosystems to recover their properties despite disturbances and to return rapidly to their original state. From a functional point of view, extreme events, particularly climatic (droughts, ice, storms) and biotic (pests and diseases), affect the physiology, development, competition, phenology, carbon balance [17,33,39] and can cause severe damage to forest ecosystems, including selection against less resistant species. It was reported that year-to-year variability is more important than climatic parameter averages [41]. The impact of extreme events is determined to a greater or lesser extent by the antecedent conditions [5]. In a recent review, Gutschick and BassiriRad [34] assess that extreme events affect physiology, ecology, and possibly evolution of plants; they observe the lack of a precise and biologically meaningful definition of extreme events. They propose that “an extreme event is an episode in which the acclimatory capacities of an organism or population are substantially exceeded”, leading either to death or to a prolonged recovery phase impacting physiological and developmental responses. An extreme event acts either as an external disturbance for which forest systems can resist, or as a disturbance exceeding the resilience of forest ecosystems, i.e. their ability to return to their former dynamic state. Both situations will be discussed here from examples of temperate forest ecosystem response to drought or massive defoliation by insects. We did not address cases of extreme storms, late frost, bark beetle outbreaks, even if they all are frequent extreme events in Europe and able to induce large and sudden tree mortalities.
Historical extreme droughts, sometimes associated with excessive air temperature, are mentioned in France since 1137 during which there was a 7-month long exceptional drought, 1540 (dry and hot), 1676 (rainfall deficit), 1719 (dry and hot), 1859 (July temperature record), 1921 (dry), 1923 (August temperature record above 40 °C), 1976 (exceptional drought)… extreme dry years occurred about ten times per century… until the 20th century [2]. Trends in homogeneous time series of observed extreme weather and climate indices suggest changes in the climatology of extreme events as discussed by Planton et al. in this issue [64], in France [51], as well as over Europe [49]. Forest managers are aware of an increase in the frequency and intensity of climatic hazards predicted by some global climate change projections, but they are concerned by their inability to cope with such extreme events. Although forest ecosystems are usually assumed to respond to gradual change in a smooth way, sudden drastic switches may occur [68], and stochastic weather extremes or pest outbreaks may coincide with a loss of resilience resulting from both forest management and environmental gradual changes. As the occurrence of such extreme events is likely to increase, we need to include their potential consequences in our research on expected future tree species distribution, migration and production. The degree of climatic suitability for trees in both actual and potential locations has to ensure tree population viability [56]. Biogeographic distribution may be affected primarily by changes in weather extremes rather than by changes in mean climatic variables that are commonly used in biogeography predictions [3]. The aim of the paper is to give an overview of how forest ecosystems could be affected by some extreme events. We gathered from literature and our own research some examples of tree growth and dieback due to climatic or biotic hazards, illustrated from forest health monitoring networks and studies of both dendroclimatic and ecophysiological approaches.
2 Results and discussion
Most of the literature dealing with forest decline investigates whether climatic events predispose to or trigger current forest damage. To be sustainable, forest management needs information on how and to what extent tree growth, crown condition and tree mortality are affected by climatic conditions.
2.1 Tree rings respond to climatic condition, but cannot estimate ecosystem response to extreme events
Tree radial growth is another efficient indicator of forest response to climate [20,30], including recurrent or sustained droughts [50]. Depending upon growth ecology, annual rings data may help quantifying forest responses to climate variations and extremes [63]. Extreme events (climatic as well as biotic) impact tree growth in an as wide an area as was affected by the hazard [57]: if most of the trees reduce their annual growth, relative growth reduction depends on species sensitivity to the event. Dendrochronologists are very familiar with such growth response. They call “pointer years” years leading to annual rings that differ visibly and markedly from the preceding and subsequent rings, especially having larger or smaller total width. Local or regional pointer years [54], which are the basis for cross-dating ring chronologies, are also efficient tools to investigate the synchronous disturbances responses of trees [70].
Analyzing the tree ring database from INRA Phytoecology Team, we gathered 20 dendro-ecological studies, each including at least 150 trees from a species in a forest or in an ecological region. Our database included Quercus petraea and Quercus robur in plain regions, Fagus sylvatica in both plain and mountains, Fraxinus excelsior in alluvial conditions, several species of Pinus, and Abies alba in mountain context as more frequently studied species. We analyzed the presence/absence of pointer years in each master chronology (one region and one species averaged tree ring series) from 1908 to 1984, with no differentiation between wide or narrow rings, i.e. between positive or negative pointer years. Fig. 1 illustrates the factorial analysis of the 0/1 matrix. Since climatic conditions are key determinants of tree growth, the expected result was a geographical grouping of the studies. However, the species was the first discriminating factor: for example, a better agreement was found between beech in the Vosges Mountains or Lorraine Plain and beech in Brittany as confirmed by ecophysiological studies [59,60], than between beech and fir, growing both in the Vosges Mountains. This means that species with contrasting ecophysiology should not be mixed to analyze regional climatic impacts. As an illustration, if oaks and beech respond mainly to water balance and drought, fir and pine growths are more related to temperature in our dataset.

Factorial analysis of presence/absence of pointer years between 1908 and 1984 (77 years) in 20 dendroecological studies from Phytoecology Team Database, INRA Nancy. Sample size ranges from 150 to 1025 trees per study. Each symbol represents one species studied in a given ecological context: Quercus robur (QR); Quercus petraea (QP); Fagus sylvatica (FS); Abies alba (AA); Fraxinus excelsior (FE); Pinus uncinata (PU); Pinus sylvestris (PS).
Analyse factorielle de la présence/absence d’années caractéristiques observée dans les chronologies de cernes entre 1908 et 1984 (77 ans) dans 20 études dendroécologiques (base de données Équipe Phytoécologie, INRA Nancy). Chaque étude comporte entre 150 et 1025 arbres. Chaque symbole représente une espèce étudiée dans un contexte écologique : Quercus robur (QR) ; Quercus petraea (QP) ; Fagus sylvatica (FS) ; Abies alba (AA) ; Fraxinus excelsior (FE) ; Pinus uncinata (PU) ; Pinus sylvestris (PS).
In another study, we investigated the influence of climate on the radial growth of beech using 15 mature stands of the French Permanent Level II Plot Network, under different climatic and soil conditions. The three most frequent negative pointer years result from a particularly intense and durable drought (1959, 1976, 1989), whereas positive years coincide with wet conditions (1977, 1958) [43]. Schweingruber (cited by [19]) also analyzed pointer years in spruce (Picea abies), fir (A. alba) and beech (F. sylvatica) from northern Switzerland. He reported too that the different species reflect climatic influences in different ways. Of the 14 pointer years between 1920 and 1985, only two occurred in all three species and corresponded to an extreme climatic event (drought or frost). It should also be mentioned that some extreme events may lead to an absence of annual ring (so called missing ring) or false ring (including bands of abnormal tissue within the annual ring). Such missing rings could be detected by a cautious cross-dating of tree rings chronology, and are often interpreted as resulting from the occurrence of extreme environmental condition such as drought [19,30] or frost during the growing season. Pines, fir, spruce and beech are to a lesser extent affected by such growth abnormality, meaning strong physiological disorders leading to direct effect on cambial activity.
Tree rings associated to climate provide information about the average relationship between climate and tree growth. However, climatic forcing of a single extreme year in a tree ring series is not revealed for statistical reasons [26,27], and a suitable approach is to compare extreme growth events with instrumental data [69]. Another limit of using tree rings is that the retrospective analysis of tree growth may lead to underestimate the impact of extreme events, especially in managed forests. A retrospective analysis of radial growth is limited to a population of living trees and, if we assume that some trees die after each historical extreme event (and were harvested in managed forests), they cannot be included anymore in the studied sample. As a result, the growth decreases exhibited by the master tree ring chronology are underestimated because they concern only surviving trees. This bias presents a real difficulty when trying to understand the processes leading trees from decline to dieback [16]. To overpass this bias when studying managed forests, dieback studies should include both living and dead trees, implying a good coordination between foresters and researchers to core recent dead trees before harvesting.
Another important point to discuss is the tree memory of previous extreme events. A strong growth reduction is frequently reported, the duration of which depends on the species. This time lag leads to a period of growth recovery. Although a high missing ring frequency and strong growth reduction may occur, a prolonged drought does not preclude survival and recovery of trees. Either local site conditions or tree fitness may mitigate the impacts of a severe drought on the radial growth recovery. Nevertheless, some trees are so affected that they never recover their pre-extreme event growth level [21,58], due to irreversible changes in tree functioning leading in some cases to mortality [23]. The relative growth rate, calculated as the reduction of growth due to the stress [(pre-stress growth − post-stress growth)/pre-stress growth], is a useful indicator of tree death probability in mortality models [7,47].
A population of trees previously weakened by an unfavourable site, silviculture or climate conditions is more vulnerable to future stressing agents like extreme climatic events or pest attacks. Climate stress was reported as a precursor to forests decline in wide range of species and sites, including a higher susceptibility to borers (birch, [40], oaks [31]). Bud moth (Zaeraphera dinian) outbreaks severely affect tree ring in the host larch trees (Larix decidua) [4,28]. Abrupt growth changes and latewood events are detected in tree ring chronologies. Defoliation induces an immediate reduction in latewood, followed by a reduction in needle length and a significant decrease in radial growth in the subsequent year. In the case study presented here [25], oaks were totally defoliated by gypsy moth (Lymantria dispar) for two consecutive years (1993–1994) and replacement shoots were infected by mildew: this extreme biotic hazard led to abnormal dramatic and rapid mortality. In the following years, both living and dead tree populations showed a severe decrease in tree ring width, with no ring formation in 1995 or 1996 for the dead trees. Comparing the two tree ring series, the dead oaks series was lower than the living trees since 1949, which was a previous extreme dry year (Fig. 2). This population did not recover its growth, and was more vulnerable to following climatic or biotic events: the shift between the two tree populations increased at each abrupt growth changes. A sequence of negative pointer years persisting for some time reflects that strong environmental influences occurred in the 1975–1980 period. Such large and deep depressions in the ring-width chronologies reveal the nature of susceptibility of forest stands to drought disturbance. Defoliation was the extreme event leading to tree mortality, but caused mortality only in trees with a 40-year long-lasting memory of another previous extreme climatic event. In a forest chronically infested by gypsy moth on a drought-prone site in Quebec [53], drought was the more significant climatic driving factor of radial growth fluctuation in red oak, and the remaining variance of growth fluctuation was explained by the number of gypsy moth larvae per tree.

Year-to-year variation of radial growth of oaks (in North-East France) severely damaged by two successive gypsy moth defoliations (Lymantria dispar) in 1993 and 1994. The black line is radial growth of the dead trees consecutively to the defoliations; the grey line is the living trees in 1995) (from [25]).
Variation interannuelle de croissance radiale de chênes (en forêt de la France) sévèrement affectés par deux défoliations successives par le bombyx disparate (Lymantria dispar) en 1993 et 1994. La ligne noire est la croissance radiale des arbres morts à la suite de ces défoliations, la ligne grise est la croissance radiale des arbres vivants en 1995 (d’après [25]).
Pederson [59] also reported two decades typically passing before oak died in Midwestern USA. He observed from tree ring analyses that dead trees had growth patterns prior to mortality that included growth declines indicative of inciting stress, like five drought years. Pederson suggested a conceptual mortality process model in which tree physiology is affected prior to death through the reduction in photosynthetic efficiency and changes in allocation rules, leading to fine roots production reduction, nutrient and water uptake decrease, then affecting leaf biomass and finally affecting once again photosynthesis [34]. Prolonged recovery phases of water and nutrient uptake after a drought ends depend on the tree vigor prior to the extreme event. Similarly, growth from tree ring variation during the previous 20 decades in red oak species [37] (or 10–15 years in Pinus edulis [55]) had been used to predict the likelihood of drought-induced death. Dead trees exhibited 1.5 times greater climatic sensitivity in growth variation than live trees. In a recent analysis of the pine mortality in the Alps (Pinus silvestris), Bigler et al. [6] also reported a lagged mortality response after prolonged drought, either directly within a time lag of 1 or 2 years, or decades later, impaired with more complex processes and biotic agents involvement.
2.2 Tree vitality declines after extreme events
In 1985, an International Cooperative Programme (ICP Forest – www.icp-forests.org) was launched to monitor forest condition in Europe, thanks to a systematic transnational grid of 16 km× 16 km (6000 observation plots and actually 41 participating countries). At first, this network aimed at pointing out the negative effects of air pollution rather than climatic impact on forest condition [45]. Assuming that crown condition reflects the vitality of a tree, abnormal symptoms are visually assessed each year in order to date, to quantify and to interpret the health of the European forest. In the mid 1990s several main tree species recovered from their originally raised defoliation. Many papers reported a crown condition decline as a result of twigs mortality following severe droughts for beech (1976 drought for [67], 1989 drought for [35,36] and 1990 drought for [74]). Norway spruce is another highly responsive species to drought with crown density decline especially in case of early summer drought [73]. In Central Italy, the percentage of moderately or severely damaged trees displayed an upward trend after the 1989's drought, especially for Pinus pinea and F. sylvatica [15]. In Switzerland, relationships between crown condition and drought quantified by water balance and ecophysiologically-based drought index were analysed for both deciduous and coniferous species [77]. Drought was significantly related to crown condition in temporal analysis for all deciduous tree species and in the lowland forests. Solberg [72] reported that summer drought is a driver for crown condition and mortality of Norway spruce. Dry and warm summers were followed by increases in defoliation, discolouration of needles, cone formation and mortality. After subsequent years of a steady state, crown condition decline increased again following the 2003, this time consistently for all main tree species. Clearly more than one fifth of the 130,000 sample trees assessed in 2003 were classified as moderately or severely damaged. Results show that the unusual heat and drought in summer 2003 caused a severe reduction in water availability and transpiration of several forest stands in Central Europe. This led to leaf loss increase on these plots for many species as soon as 2004 and the following years (Fig. 3). A recovery of crown condition was reported in 2007 for the surviving trees (about 1% of the tree population died). The extreme heat wave and exceptional long and severe drought having prevailed in Europe in summer 2003 confirm that weather extremes are acting as predisposing or inciting factors of forest decline [71,75].
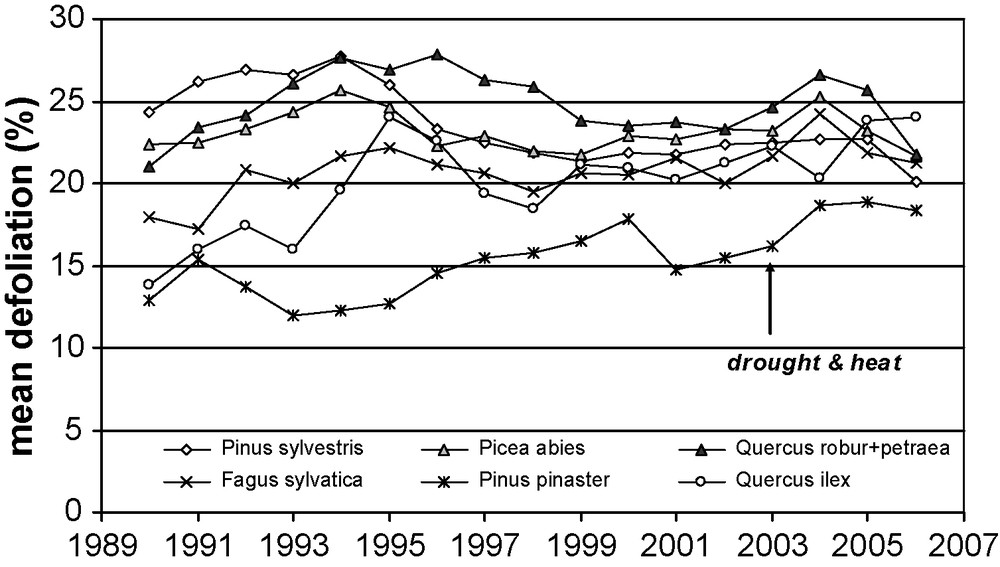
Annual changes in crown condition quantified by mean defoliation in the European Network for Forest Damage Monitoring for the most frequent tree species from level I plots in Europe. Sample size for the mean defoliation calculation depends on the species: 2530 for Pinus sylvestis, 6957 for Picea abies, 2739 for Quercus petraea and robur, 4313 for Fagus sylvatica, 2531 for Pinus pinaster and 3424 for Quercus ilex (from [46], ICP Forest, report 2007, available online: http://icp.forest.org/).
Variations annuelles de l’état des cimes, apprécié par la perte foliaire moyenne pour les espèces forestières les plus fréquentes et observées sur les placettes de niveau 1 en Europe. L’effectif d’arbres pour le calcul de perte foliaire moyenne est de 2530 pour Pinus sylvestis, 6957 pour Picea abies, 2739 pour Quercus petraea et robur, 4313 pour Fagus sylvatica, 2531 pour Pinus pinaster et 3424 pour Quercus ilex (d’après [46], source ICP Forest, rapport 2007, disponible sur http://icp.forest.org/).
2.3 From crown condition decline to exceptional mortality
Crown condition decline is a predictive index for year-to-year mortality, with an exponential increase in the mortality rate with increasing crown decline (i.e. leaf area reduction) [22]. Abnormal mortality was also observed, either soon after the climatic event (autumn 2003), or at the beginning of 2004 when spring budburst did not arise for a lot of trees. At the French scale, and always within the Level I plots, the Forest Health Department (Agricultural French Ministry) recorded a mortality rate of 1.3% for coniferous trees, representing a spectacular increase in comparison with the average normal level of 0.2%. Two reasons may be put forward:
- • the rainfall deficit led to a higher water stress for coniferous and, as the stand transpiration started early in the hot and sunny Spring 2003, the few precipitations were largely intercepted, including during the end of the season, leading to a delay for soil water content replenishment. Drought was then earlier, longer and more severe for species with evergreen crowns;
- • heat and drought also fostered bark beetle attacks in larger areas, especially damageable mainly for conifers (spruce and fir) physiologically weakened by drought. In addition, mortality was also heavy for broad-leaved species (0.4%), especially among old trees, more likely driven by extreme severe and sustained drought.
At the European scale, tree mortality varied from 0.8 to 1.2%, with a continuous increase up to 2006 after recurrent droughts, especially for broad-leaved species. Extreme drought basically triggers off terminal shoots embolism and crown dieback, but in some cases also tree mortality of drought-sensitive species like pedunculate oak, birch, poplar… Physiologically, the prolonged soil moisture depletion, in combination with generally high temperatures, might reduce carbohydrate storage, increase fine root mortality, cause a reduction of leaf area and cessation of cambial activity, and eventually predispose drought-sensitive trees to death. Mortality was especially observed during the 2004's budburst (Fig. 4): this phenological stage represents the highest carbon sink during the season, and the total carbohydrates of the trees were too low as a result of the previous drought and heat. The exceptional increase of coniferous species mortality in 2004 was the result of earlier, stronger and longer soil water deficit [13,14], direct impact of heat wave on crowns, and increase of tree vulnerability to bark beetle attacks.

Mortality rates in the French part of the European Network for Forest Damage Assessment during the 1989–2006 period (509 level I plots, including more than 10,000 trees) (from [29]).
Évolution de la mortalité moyenne pour les espèces forestières feuillues et résineuses observées sur la partie française du réseau européen de surveillance de l’état sanitaire des forêts (509 placettes de niveau 1, soit plus de 10 000 arbres)[29].
2.4 Extreme events as strong agents of natural selection
An oak decline in a 13,000 ha forest in the Alsace Plain (Rhine Valley, France) in 1994 was an opportunity to analyse growth response to recurrent and extreme droughts of two co-occurring oak species, sessile (Q. petraea) and pedunculate oaks (Q. robur). Predisposing, inciting and contributing causes need first to be identified before suggesting suitable new management to mitigate future extreme drought. Then a dendroecological study of this forest aimed at understanding the causes of oak dieback, dating the decline and comparing the growth response to climate of the two species [24]. The radial growth of oak trees was analyzed and pointer years were associated to soil water deficit. This latter was calculated retrospectively using daily climatic data (rainfall, Penman evapotranspiration), extractable water and stand leaf area index (leaf area per soil area) with a daily water balance model developed for forest canopies [32]. Fig. 5 shows the radial growth index of the pointer years as a significant function of seasonal soil water deficit: higher soil water deficits are correlated to lower growth indexes. Year-to-year growth index variation was analysed for both sessile and pedunculate oaks. Fig. 6 points out differential tree growth responses of the two co-occurring oak species [12]. From 1950 to 1970, both species exhibited similar growth and similar climatic response. However, since 1972, pedunculate oak growth declined as compared to sessile one and never recovered the latter one's growth. Overlapping soil water deficits indicate that 1972 was a drought year, affecting pedunculate oak to a larger extent. The drought intensity achieved in 1972 may be a threshold of probability to decline, especially interesting as it affected differently the two co-occurring species. A prolonged recovery phase was needed for Q. robur after the drought, indicating a lower fitness for drought tolerance due to xylem vulnerability to embolism [9], more superficial root water uptake [10] and lower water use efficiency [65,66]. As foresters reported oak mortality at this time, one may reasonably suppose that pedunculate oaks should preferentially die at this date. After a succession of years favourable for growth recovery without severe drought, a period of recurrent drought began in 1983, with the highest drought intensity in 1989, which was the more extreme event of the 30-year period under study. This extreme event resulted in a wave of abnormal mortality lasting several years and worrying forest managers. Unfortunately, foresters did not report which of the species was preferentially affected. Our study demonstrates that the 1989 drought was the inciting oak mortality, and the intensity of this extreme drought was suggested as a threshold for increasing probability of oak mortality.
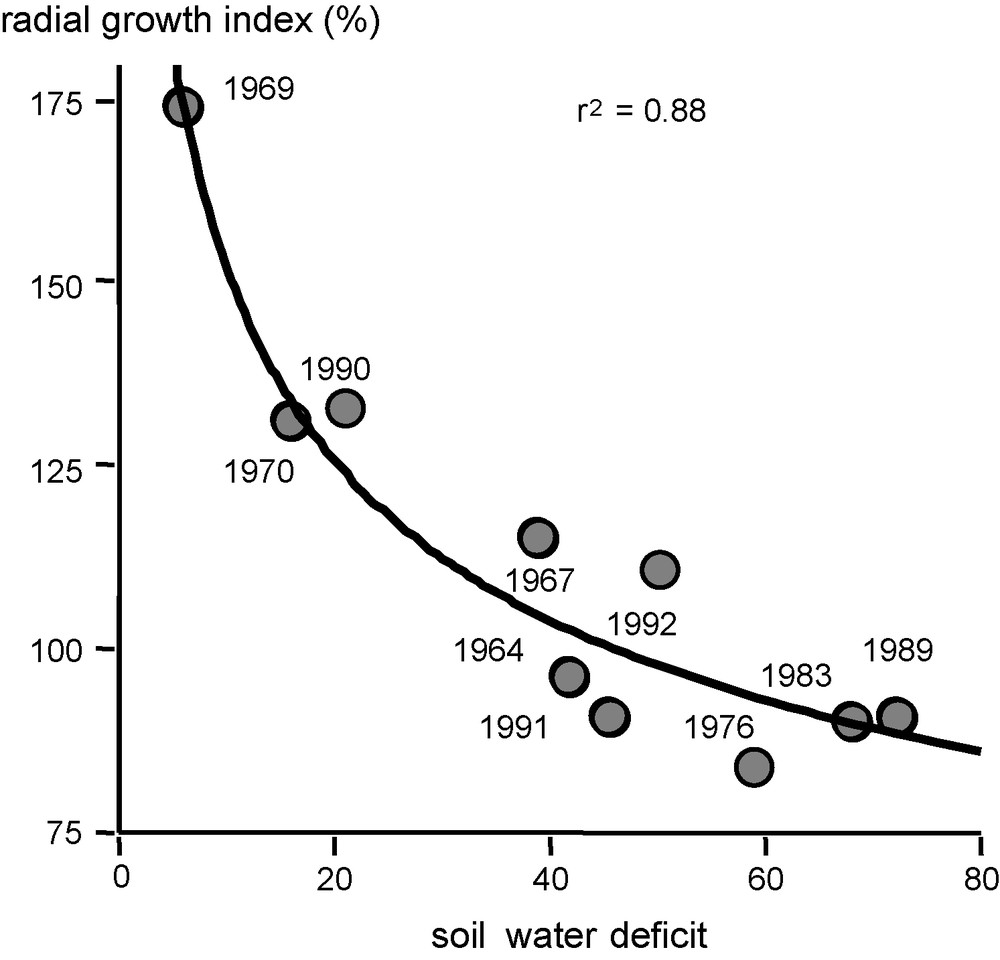
Radial growth index of pointer years for oaks’ growth from the Harth Forest (South Alsacian Plain, France) as a function of seasonal soil water deficit as calculated from bioclimatic water balance model [32]. Average parameters for water balance calculation: extractable soil water = 100 mm, leaf area index = 6, daily climatic data from Bâle-Mulhouse, Météo France. The study included 680 trees; the period of pointer years calculation is 1964–1994 (adapted from [11]).
Indice de croissance radiale des années caractéristiques pour la croissance des chênes en forêt de La Harth (Sud de la Plaine d’Alsace, France), exprimé en fonction du déficit hydrique du sol pendant la saison de végétation calculé par un modèle de bilan hydrique bioclimatique[32]. Les paramètres moyens utilisés pour le calcul de bilan hydrique : réserve utile du sol = 100 mm, indice foliaire = 6. Données climatiques quotidiennes de la station de Bâle-Mulhouse, Météo France. L’étude porte sur 680 chênes, la période de calcul des années caractéristiques est 1964–1994 (adapté de[11]).

Radial growth index variation (right axis) of sessile oaks (black line) and of pedunculate oaks (dotted line) from the Harth Forest (South Alsacian Plain, France). Seasonal soil water deficit as calculated from daily water balance model [32] was firstly computed for the period of growth measurements (1964–1994) (grey circles, left axis) and secondly for the recent time period until the exceptional drought in 2003 (open triangles). The intensity of the 1972 drought (black arrow) induced a differential radial growth decline between sessile and pedunculate oak, and this soil water intensity is considered as a threshold of risk of oak decline, represented by the grey area. The 1989 drought induced a severe oak mortality and its intensity is then considered as a threshold for risk of mortality (represented by the black area). The calculation illustrates that the intensity of 2003 extreme drought was never achieved since 1964 (period of daily climatic data availability) and was in the area of risk of mortality. Average parameters for water balance calculation: extractable soil water = 100 mm, leaf area index = 6, daily climatic data from Bâle-Mulhouse, Météo France. The study included 415 sessile oak trees and 262 pedunculate oak trees.
Indice de croissance radiale (axe de droite) de chênes sessile (ligne noire) et pédonculé (ligne pointillée) en forêt de La Harth (Sud de la Plaine d’Alsace, France). Le déficit hydrique du sol pendant la saison de végétation a été calculé à l’aide d’un modèle de bilan hydrique journalier[32], au cours de la période de croissance radiale (1964–1994) (cercles gris, axe de gauche), puis sur la période récente jusqu’à la sécheresse exceptionnelle de 2003 (triangles ouverts). L’intensité de la sécheresse de 1972 (flèche noire) a induit une réduction différentielle de croissance radiale entre chênes sessiles et pédonculés, et l’intensité du déficit hydrique de cette saison est considérée comme un seuil de risque de dépérissement (représenté par la surface grisée). L’intensité de la sécheresse de la saison 1989 a induit une mortalité sévère de chênes et cette intensité est considérée comme un seuil de risque de mortalité (représenté par la surface noire). Le calcul illustre que l’intensité de la sécheresse extrême de 2003 n’a jamais été atteinte depuis 1964 (date de disponibilité des données climatiques quotidienne) et que son intensité est dans la zone de risque de mortalité. Paramètres moyens utilisés pour le calcul de bilan hydrique : réserve utile du sol = 100 mm, indice foliaire = 6. Données climatiques quotidiennes de la station de Bâle-Mulhouse, Météo France. L’étude porte sur 415 chênes sessiles et 262 chênes pédonculés.
To anticipate the consequences of the exceptional summer in 2003, we computed the soil water deficit during the recent period, and concluded that the 2003 drought intensity was so strong that a new oak dieback episode was highly probable. This was unfortunately confirmed by an increase in mortality from 0.2 to 1.5% in 2005, to 0.6% in 2006 and to 1.0% in 2007, cumulated mortality reaching 3%; a new wave of forest decline is in progress according to the definitions of the French Forest Heath Department. An ecophysiological study of these oaks performed in autumn 2003 indicated that the probability of oak mortality may be expressed as a function of total carbohydrate content after the extreme event. For oaks, it seems that below a threshold depending on tree age, trees are unable to recover and survive after an extreme event. This suggests which kinds of functional traits are likely to be selected by extreme events. Carbon allocation strategy, i.e. partitioning among growth, maintenance respiration and storage, may be a key process for oak performance to recover one's carbon balance after an extreme event: carbon allocation may act as a strong contributor to fitness in an extreme event. The understanding of the oak decline processes in this forest allows us to draw vulnerability maps (soil, stand leaf area index and species composition i.e. sessile versus pedunculate oak) and to draw areas exhibiting a high probability of decline resulting from the 2003 extreme drought hazard. This study demonstrates that extreme drought may act as an important pressure selection against the less tolerant species. Extreme event themselves, leading to marked changes in fitness, may be strongest agents of natural selection [34]. The fitness of pedunculate oak is lower as compared to sessile oak. Pedunculate oak was historically expanded by both anthropic (sylviculture as coppice with standards widely applied in this forest, introduction of seeds) and ecologic factors (pioneer species) leading to pedunculate oak trees to regenerate under non-optimal site condition. Partial species replacement could occur, since the drought-tolerant species could be favoured to the detriment of the more mesic species. Nevertheless, thanks to the exceptional genetic diversity in oaks (including pedunculate oak) [62] and to the pioneer behaviour of pedunculate oak (important fruiting, high growth rate in young age), no species extinction risk is actually threatening. It is today still a matter of proportion in the mixed stand composition according to site condition.
According to a widely prevalent and intuitive idea, mixed forest communities could insure against climate change-related pressures [8]. Our study reporting the counterintuitive observation that a mixed (as compared to pure) oak forest (Q. robur and Q. petraea) is more vulnerable to decline after extreme drought is interesting. The more vulnerable species is more prone to decline and dieback, and mixed stands are more disturbed by sanitary sylviculture: soil could be strained and soil quality durably damaged. Wallace et al. [76] also reported an increase in oak mortality in a mixed-oak forest, after a nitrogen saturation experiment. Mueller et al. [52] reported that six drought years, including the extreme droughts of 1996 and 2002, led to widespread tree mortality across northern Arizona. Six-fold higher mortality occurs in large dominant P. edulis as compared to Juniperus monosperma. Differential mortality of large pinyons resulted in a vegetation shift such that the pinyon–juniper woodlands are becoming dominated by juniper. Severe and sustained drought was also mentioned as a triggering factor for the dieback of remnant forests [44]: tree ring analyses coupled with historical climatic records were used to investigate dieback of Meyer spruce (Picea meyeri) forest in the North China typical steppe. A persistent drought event from 1922 to 1932 implied that the extreme drought in the 1920s was probably the underlying cause of Meyer spruce mortality. Severe drought also affects wood flora in Spain according to functional types, leading to shifts in forests and shrubs composition [61]. All these examples are illustrations of possible changes in species assemblages from the current ones as a result of extreme events, especially drought [18].
2.5 Adaptative management should amplify the natural selection pressure by extreme events
We are rarely able to cope with the consequences of extreme events, due to lack of anticipation and to unpredictable stochastic events like droughts or disease outbreaks. Forest management is challenged by the increasing need to adapt practices to a climate likely to be characterised by a changing frequency of extreme weather events. As a result, forest stands will probably have to face more pronounced disturbances, both from climatic events, fire risks and parasite attacks. After the 1999 winter storms Lothar and Martin in France, some advice has been suggested for sylviculture practices aiming at increasing tree stability by increasing the height/diameter ratio and harvesting trees in a shorter rotation time to limit total tree height. For extreme drought hazards, we propose to foresters:
- • to recognize which of the co-occurring species is more drought sensitive (pedunculate oak for example);
- • to anticipate financial loss by harvesting more sensitive trees before they die (aging or crown condition damage) – this management advice will accelerate the regression of the less tolerant and less productive species due to decline;
- • to mitigate drought severity by adjusting stand canopy to a lower leaf area index by cutting more coppice trees and maintaining coppice below the dominant canopy [11].
The last advice is possible as the market for wood energy is expanding again. Lowering the duration and intensity of drought by thinning will also increase the resilience of the remaining stand to a future precipitating event. Forest management and utilization have a considerable influence on the stability and sustainability of forest ecosystems. Sustainable management practices and strategies should take care of provenances or species choice, tree breeding, harvesting practices, as well as the silvicultural system applied [42]. The challenge is to sustain a large stability of forest stands, keeping a high resilience which could be affected by gradual changes like climatic trends, acidification, nitrogen saturation [76] or pollution increasing the vulnerability of ecosystems. Building and maintaining resilience of desired ecosystem states is likely to be the most pragmatic and effective way to manage ecosystems in the face of increasing environmental changes [68].
3 Conclusion
Through several examples, we illustrate how forest ecosystems respond to some climatic or/and biotic extreme events according to acclimation or selection of trees population with lower fitness. We suggest that extreme events are able to select trees with water-conserving or water-preserving traits helping for tolerance of the extreme. Our research perspectives are to go deeper in this hypothesis [1], using multifunctional traits into account: gas exchanges (stomatal control, water use efficiency), but also traits for resources acquisition (allocation to roots, water uptake and nutrient efficiency) and carbon allocation patterns (carbohydrate storage, stem growth, leaf area). A better understanding of such an allocation adjustment, in short or long terms, is a key for extreme event functional response among different tree species [48].
The death of trees was the ultimate stage triggered by extreme drought that acts as a bottleneck event affecting changes in co-occurring species. Five years after the exceptional 2003 summer, forest declines are mentioned in many forests all over Europe. Analyzing in real time the processes involved in either mortality or recovery, especially in mixed stands of contrasting species, is an exciting challenge for forest ecophysiologist and ecologist scientists.
Finally, new insights for understanding and classifying the factors of vulnerability of forest stands to extreme events should help forest managers anticipate catastrophic mortality and reduction in productivity or biodiversity. Silviculture should, to some extent, limit the intensity of extreme events and decrease individual tree vulnerability. The resilience of forest ecosystems to extreme events has to be qualified in a different way than that to gradual climate change, as the response time is shorter, without possible acclimation. Moreover, drastic conjunctions of biotic and climatic extreme hazards for a number of years should become more damaging, in a context where forests stands are more vulnerable due to conservative sylviculture, species provenance or individual selections for increasing productivity at the expense of drought or pest tolerance. New tradeoffs between performance and resilience have to be adapted by forest managers in the more exposed area to extreme climatic hazards.
Acknowledgements
The authors would like to acknowledge the Académie des sciences which has given the opportunity to publish this paper and for the stimulating discussion of their results during the Ecosystems and Extreme Climatic Events workshop held in Paris, 4–6th July 2007.