1 Introduction
The North-West (NW) of the Iberian Massif is located at the hinge zone of the Ibero-Armorican Arc (Fig. 1) and preserves relicts of oceanic domains that once separated the Paleozoic continents [50,51]. A suture occurring in the hanging-wall of a large thrust system is rootless, which makes its interpretation difficult. However, the excellent exposure of the ophiolitic and associated allochthonous terranes permits the establishment of a sequence of emplacement, crosscutting relationships, and metamorphic gradients. In the absence of continental-scale strike–slip shear zones and faults, the Galician–northern Portugal section is retrodeformable, permitting qualitative palinspastic reconstructions of the Gondwana–Laurussia convergence.
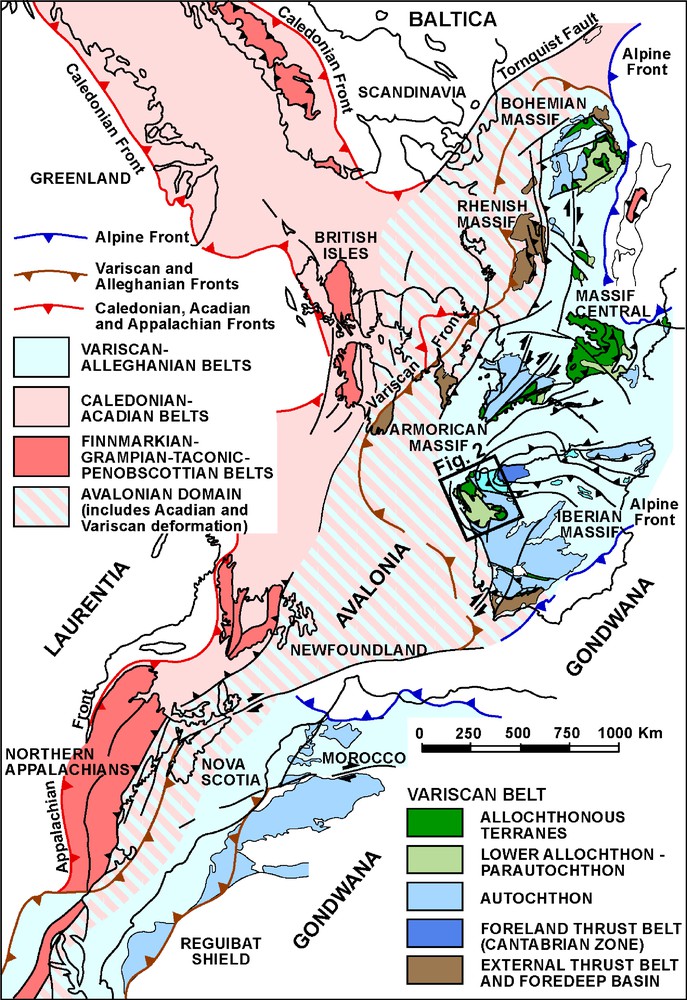
Location of Iberia in relation to the Paleozoic orogenic belts at the end of Variscan convergence. Modified after Martínez Catalán et al. [46].
Fig. 1. Situation de l’Ibérie par rapport aux ceintures orogéniques paléozoïques, à la fin de la convergence varisque. D’après Martínez Catalán et al. [46], modifiée.
These characteristics make of NW Iberia a key site to unravel the history of the Paleozoic plate evolution of the circum-Atlantic region, and specially that of the Rheic Ocean. This contribution aims to be a synthesis of the research carried out during the last 25 years, when the geology of NW Iberia has been considered in a plate tectonics and terrane perspective. It is based on previously published syntheses [38,48], and on data published elsewhere, being presented here in a very concise way together with the key references.
2 Geological setting
The NW Iberian basement consists of plutonic and metamorphic rocks, the latter ranging from very low- to very high-grade. The structural fabric (Fig. 2) alternates linear trends due to thrust faults and narrow folds, with closed structures corresponding to open domes and basins that are a product of late orogenic collapse and extension [48]. A distinction is made between the autochthon and allochthonous terranes. The autochthon consists of a thick metasedimentary and volcanic sequence and includes a foreland thrust belt, the Cantabrian Zone (CZ), and more internal zones where the Paleozoic succession is thicker and rather more complete (Figs. 1 and 2). The autochthonous sequence was deposited in northern Gondwana during the Late Proterozoic and Paleozoic, as indicated by sedimentary and faunal evidence [63] and by detrital zircon age populations [47].

Geological map and cross sections of North-West (NW) Iberia showing the allochthonous complexes and their units. For location, see Fig. 1. Modified after Dallmeyer et al. [21].
Fig. 2. Carte et coupes géologiques du Nord-Ouest de l’Ibérie montrant les complexes allochtones et leur unités. Pour la situation, voir Fig. 1. D’après Dallmeyer et al. [21], modifiée.
The allochthonous units (Fig. 2) are the remnant of a large nappe stack formed by exotic terranes cropping out in the complexes of Cabo Ortegal, Órdenes, Malpica-Tui, Bragança and Morais. Many are derived from peri-Gondwana, and many bear the imprint of Paleozoic subduction. They include fragments of a Cambro-Ordovician ensialic island arc (upper units) [8,18,71], and distal parts of the Gondwana continental margin (basal units) [44,72]. Ophiolitic units sandwiched between the upper and basal units include remnants of a Cambro-Ordovician back-arc [14,69], possible evidence of Ordovician oceanic crust [60], and suprasubduction type, Early–Middle Devonian ophiolites [60,70].
A thrust sheet several kilometres thick separates the basal, ophiolitic and upper units from the autochthon. It consists of Ordovician and Silurian metasediments and volcanics, and is known variously as the parautochthon [62], the Schistose Domain [26,77], and the lower allochthon [48], this last term describes well the superposition of older over younger rocks, the imbricate character, and the large displacement involved. The lower allochthon has stratigraphic and igneous affinities with the Iberian autochthon [26,77], and represents a distal part of the Gondwana continental margin.
3 Creation of a peri-Gondwana, ensialic island arc
The upper allochthonous units occupy the core of the allochthonous complexes (Fig. 2), and two different tectonometamorphic histories support a subdivision into intermediate-P upper units above, and high-P and high-T upper units below, but with both groups belonging to the same coherent terrane.
3.1 Intermediate-P upper units
These units occupy the highest structural position and consist of terrigenous sediments intruded by Late Cambrian gabbros and granitoids (Fig. 2). The metamorphic grade ranges from top to bottom between the greenschist and the granulite facies, and shows abrupt changes at extensional detachments [1,40]. High-grade rocks occur as large massifs of metaigneous rocks in the Órdenes Complex (Fig. 2). The Monte Castelo gabbro is similar in composition to modern island-arc basalts [8]. The Corredoiras orthogneiss is granodioritic to tonalitic [40], whereas a minor gabbroic intrusion in the Cabo Ortegal Complex is calc-alkaline and characteristic of a volcanic arc setting [18]. The Corredoiras and Monte Castelo massifs have yielded U–Pb ages of 500 Ma [3], and were affected by granulite-facies shear zones at ca. 480 Ma [3,5].
Mesozonal metasediments represent a Barrovian pile with metamorphic zones ranging from almandine to sillimanite [18]. Kyanite replacing andalusite indicates burial after heating, as in the Monte Castelo gabbro and the Corredoiras orthogneiss. Monazites from the sillimanite zone have yielded 493–496 Ma [3], reflecting Cambro-Ordovician, intermediate-P regional metamorphism.
Epizonal metasediments occupy the uppermost structural position and consist of metapelite and greywacke of turbiditic character, with alternations of quartzite and conglomerate. When considered together with the chemistry of the Monte Castelo gabbro, these terrigenous sediments, rich in volcanic components, suggest a volcanic arc environment, although the absence of truly volcanic rocks points to a dissected magmatic arc [31]. Detrital zircon ages in metagreywackes yielded three age populations of 2.5–2.4 Ga, 2.1–1.9 Ga and 610–480 Ma [31], establishing an Early–Middle Ordovician maximum depositional age.
3.2 High-P and high-T upper units
These units, in the complexes of Bragança, Cabo Ortegal and Órdenes, include paragneisses and ultrabasic metaigneous rocks, but the most characteristic are garnet–clinopyroxene granulites and eclogites retrograded to the amphibolite facies. Gabbros occur in several stages of transformation, from relatively little metamorphosed rocks to coronitic metagabbros, high-P granulites and amphibolites [10]. Subophitic and diabase textures indicate emplacement at relatively shallow levels. The basic rocks are tholeiitic metagabbros with a MORB geochemical signature [35], but geochemical studies of the ultramafic rocks are consistent with generation in an arc setting [71].
U–Pb data in metabasic rocks have yielded 520–480 Ma, viewed as protolith ages [54], but could also reflect the imprint of nearly contemporaneous metamorphism [30,32,57]. However, the high-P granulite and eclogite facies metamorphism is dated, by U–Pb and 40Ar/39Ar methods, between 425 and 390 Ma [30,32,37,54,66,73]. This event involved subduction [36], and was successively followed by decompression, partial melting, penetrative amphibolite-facies mylonitization, which was dated at 390–380 Ma [20,21,37], recumbent folding, and thrusting [35,42,52].
3.3 The island arc and its fate
The geochemistry of the plutonic and ultramafic rocks, and the abundance of volcanic components in the metasediments are features consistent with generation of the upper units in an arc setting. The metamorphic evolution of the intermediate-P units points in the same direction, as the P–T paths include isobaric heating related to the intrusion of large plutons, followed by isothermal burial implying crustal thickening [4,18,40]. Pressurization in the sillimanite field and a subsequent anticlockwise evolution are linked to active plate margins.
Late Cambrian–Early Ordovician (500–460 Ma) magmatism is widespread in the upper and basal allochthonous units and the autochthon, whereas inherited zircon ages from orthogneisses of all of them are similar, and suggest derivation from the West African craton [57,72]. Detrital zircon ages, from low-grade metasediments in the upper units, also record the major events in NW Africa [31]: this evidence points to a common basement and suggests that the island arc was ensialic (Fig. 3a) and may represent a peri-Gondwanan fragment drifted away to open the Rheic Ocean (Fig. 3b).

Proposed stages in the tectonic evolution of North-West (NW) Iberia. CZ: Cantabrian Zone; LAT: lower allochthon thrust; LD: Lugo dome; LFT: Lalín-Forcarei thrust; MBT: Mondoñedo basal thrust; OST: out-of-sequence thrusts; PSD: Pico Sacro detachment; PTSZ: Porto-Tomar shear zone; VF: Viveiro fault. Modified after Martínez Catalán et al. [48].
Fig. 3. Stades proposés pour l’évolution tectonique du Nord-Ouest de l’Ibérie. CZ : Zone Cantabrique ; LAT : chevauchement de l’allochtone inférieur ; LD : dôme de Lugo ; LFT : chevauchement de Lalín-Forcarei ; MBT : chevauchement de Mondoñedo ; OST : chevauchements «hors séquence » ; PSD : détachement de Pico Sacro ; PTSZ : zone de cisaillement de Porto-Tomar ; VF : faille de Viveiro. D’après Martínez Catalán et al. [48], modifié.
The ensialic arc later became involved in the Variscan collision. The Cambro-Ordovician arc-related metamorphism was preserved in the intermediate-P units, but was overprinted by a Silurian–Early Devonian (425–390 Ma) tectonometamorphic event in the high-P and high-T units [30,32,37]. This event involved subduction of part of the arc, and resulted from accretion to Laurussia [37,38,45,48] while the Rheic Ocean was still open (Fig. 3c).
4 Cambro-Ordovician rifting
4.1 Paleozoic succession of the autochthon
Two facts support Early Paleozoic extension:
- • the high rates of subsidence deduced from the thick preorogenic succession;
- • the voluminous Early Ordovician magmatism.
The dominant sediments are pelite, sandstone, quartzite, and limestone in formations and groups of large lateral extent. Lithologic associations and facies indicate deposition in a passive continental margin, which succeeded the Cadomian Andean-type activity [27]. Differences in thickness in Middle Cambrian to Late Ordovician deposits indicate extensional activity in the margin (Fig. 3b).
Basic to acid volcanism spans from the Cambrian to the Silurian, but is voluminous in the Early Ordovician, when the Ollo de Sapo Formation (Fm.) rocks erupted. It includes a volcaniclastic sequence, rhyolitic–dacitic tuffs, coarse-grained tuffs, welded ignimbrites, and augengneisses [24]. In the Sanabria region, two large volcanic domes have been identified, suggesting that the Ollo de Sapo Fm. includes several volcanic edifices replacing each other along the 570 km of outcrop [24]: it has yielded Early Ordovician ages of 495–472 Ma [24,53]. Granitoids with ages of 500–465 Ma were intruded in Early Cambrian and Neoproterozoic successions [15,76].
The Ollo de Sapo and the granitic orthogneiss are peraluminous and of calc-alkaline affinity, plotting into the field of the volcanic-arc granites. However, the apparent stability of the margin indicated by the contemporaneous deposits suggests that their geochemistry could have been inherited from the source rocks. The Early Ordovician felsic magmatism has been interpreted as the result of extension in northern Gondwana (Fig. 3b), where the crust and mantle, previously hydrated by long-lasting Cadomian subduction, would have molten during decompression [24].
4.2 Continental rifting in the lower allochthon
Metavolcanic rocks of basic to acid types are common in the Schistose Domain, although never voluminous. Metadacites derived from subaerial lavas and metarhyolites derived from ignimbrite eruptions have been described, as well as syenite intrusions and rhyolitic domes [7,9,33]. An alkaline rhyolite in the Cabo Ortegal Complex yielded an U–Pb age of 475 Ma [77]. That age and the chemistry of the lower volcanic levels compare with the Ollo de Sapo Fm., and the presence of alkaline and basic rocks here are additional arguments for Cambro-Ordovician rifting.
4.3 The continental rifting in the allochthonous complexes
Good evidence for Early Paleozoic continental rifting comes from the basal units, which include terrigenous metasediments and igneous rocks. In Portugal, the igneous rocks form a bimodal suite of rhyolitic tuffs, intrusive porphyries, metadiabases and metabasalts [61]. In Galicia, amphibolites of tholeiitic composition, some corresponding to alkali basalts [43], alternate with granitic orthogneisses showing meta- to peraluminous character, and calc-alkaline, alkaline and peralkaline compositions. The latter are A-type granitoids derived from the mantle and are genetically linked to alkali basalts [58]. The orthogneisses yielded U–Pb ages of 490–460 Ma [72], and the ensemble reflects the Early Ordovician rifting episode [58] associated with the separation of a peri-Gondwanan fragment and the creation a new continental margin in northern Gondwana (Fig. 3a).
5 Birth of the Rheic Ocean: witnesses of initial back-arc spreading
Ophiolitic units occupy an intermediate position in the allochthonous complexes (Fig. 2). The oldest Paleozoic units of ophiolitic affinity include metabasites, metapelitic phyllites and schists, with some serpentinites, metacherts and granitic orthogneisses dated at 497 Ma [14]. The dominant lithology is greenschist-facies metabasites with relict igneous textures indicating a metabasaltic and, to a lesser extent, gabbroic origin. These units are viewed as a thick oceanic sequence of basalts and interbedded pelitic and siliceous sediments, overlying in tectonic contact, serpentinized ultramafic rocks which represent the suboceanic mantle.
The basic rocks include island-arc tholeiites and suprasubduction basalts [14,69]. The orthogneisses plot into the field of volcanic arc granitoids. Both mark the opening of a back-arc behind the ensialic peri-Gondwanan island arc during the Latest Cambrian, possibly by slab roll-back linked to oceanic subduction (Fig. 3b). The subsequent spreading of the back-arc created the Rheic Ocean, but these units remained close to Gondwana during its opening, as indicated by their structural position, above the basal units, and with a comparable metamorphic evolution.
6 Closure of the Rheic Ocean
Two of the ophiolitic units bear the imprint of oceanic closure in their suprasubduction zone geochemical fingerprints and ages. Moreover, the high-P metamorphism of the basal allochthonous units also points to the closure of the Rheic Ocean.
6.1 Early Devonian intraoceanic, suprasubduction zone ophiolite
The Careón Unit is an ophiolitic sequence exposed in the Southeast of the Órdenes Complex. A harzburgitic composition can be inferred for the ultramafic section [22], whereas the gabbroic section is a complex network of multiple intrusions of gabbro, wehrlite, and diabasic to pegmatoid dikes. The metabasites have transitional characteristics between N-MORB and island-arc tholeiites. Their immobile trace element pattern bears a diagnostic negative Nb anomaly indicating a suprasubduction zone setting [22,70].
U–Pb geochronology in two gabbros yielded an age of 395 Ma [22,59], whereas ages of 405–396 Ma were obtained in a gabbro of the equivalent Morais–Talhinhas Unit of the Morais Complex [60], bringing evidence for oceanic crust generation by the Early Devonian. Initial ɛNd values, ranging between +7.1 and +9.2, obtained for a crystallization age of 395 Ma, from Sm–Nd isotopic data [59], indicate that the rocks were derived from the depleted mantle reservoir at the time of their formation. Thermobaric estimates on a metamorphic sole unit within the Careón Unit yielded T ≈ 650 °C and P ≈ 1.15 GPa, pointing to a subduction environment for ophiolite imbrication [22]. A well-foliated amphibolite was dated at 377 Ma [21], interpreted as a cooling age following the metamorphic thermal peak.
6.2 Metamorphic evolution of the basal units
Early Variscan, high-P metamorphism in the basal units is demonstrated by eclogites, jadeite-bearing orthogneisses, and blueschists, and is interpreted as the result of subduction [9,12,13,34,41,64,65,67]. Peak pressure ranges between 1–1.65 GPa in the Órdenes Complex, where P–T gradients along a large recumbent anticline indicate a west-directed polarity (in present coordinates for the subduction [44]). The paleo-dip of the subduction zone has been estimated from the P–T conditions and thermal modeling between 15 and 20 °C [6]. According to isotopic data, subduction started before 370 Ma ago and ended at ca. 365 Ma [64], being followed by a strong decompression, related to thrusting and tectonic denudation.
6.3 Landmarks of oceanic closure
While the Careón and Morais–Talhinhas ophiolites witness a stage of consumption of the Rheic Ocean by intraoceanic subduction (Fig. 3d), subduction of the basal units marks its final closure (Fig. 3e). Deformation and metamorphism progressed eastward and their imprint is reflected in the progressive younging of metamorphic ages from the upper to the basal allochthonous units. In the upper units, 425–390 Ma ages reflect Silurian to Early Devonian compression that created a thick metamorphic pile whose deep parts registered pressures of 1.8 GPa [35,52]. An accretionary wedge, developed at the Laurussia margin (Fig. 3c–e), involved thickening, underthrusting and subduction of the upper units. This orogenic event is pre-Variscan and linked to spreading of the Rheic Ocean, rather than Laurussia–Gondwana convergence [38].
Amphibolite facies metamorphism dated at 390–380 Ma was retrogressive in the high-P and high-T upper units, and prograde in the underlying ophiolites, where it reflects the imbrication of oceanic lithosphere during the closure of the ocean. Thrusts formed at that time (ca. 380 Ma) found the 395 Ma old oceanic lithosphere still hot, and produced metamorphic soles [22]. These thrusts are seen as the first manifestation of early Variscan convergence.
The Cambro-Ordovician ophiolites, that were present during back-arc spreading and which remained on the margin of Gondwana, were the next to enter the accretionary wedge [14]. Afterward, the Gondwana continental margin went into the trench and its edge became subducted between 375 and 365 Ma [64,72]. This Late Devonian event, that reached peak pressures of 1.5–1.7 GPa, marks the last stage of subduction-related early Variscan convergence (Fig. 3e).
7 Variscan collision
Laurussia–Gondwana convergence continued during the Carboniferous in a collisional regime considered, in a strict sense, to be the Variscan Orogeny.
7.1 Continuous shortening of the Gondwana continental platform
The first deformation event (D1) produced east-vergent recumbent folds (Fig. 3f) [49]. 40Ar/39Ar dating of the S1 cleavage yielded 359 Ma, close to the allochthonous complexes, and 336 Ma to the east [21], showing that shortening was diachronous and younger toward the external zones. D1 was the result of the stacked allochthonous terranes pushing against Gondwana as a backstop limited, at its bottom, by a west-dipping sole thrust. Once continental subduction became locked, shortening began in the inner parts of the continental platform, giving rise first to recumbent folds (D1), and then to large thrust sheets (D2).
7.2 Thrust propagation and loss of the suture root
Three large thrust systems are responsible for the emplacement of the allochthonous complexes. First, the Lalín-Forcarei thrust (LFT) (Fig. 3f) carried the basal units over the lower allochthon. Then, the upper and ophiolitic units moved over the basal units and the lower allochthon, becoming strongly imbricated in a new thrust system, which was developed out-of-sequence (OST) (Fig. 3g). During thrust emplacement, large recumbent folds developed in the upper units [38,42], the ophiolites [14], and the basal units [23,44].
The OST thrusts cut and dismembered the suture, which was steeper [46]. So, a part of it was emplaced over the lower allochthon and subsequently over the autochthon, whereas its deeper, inner parts were left behind, disconnected and hidden (Fig. 3g). Shortly after, the Schistose Domain was emplaced along the lower allochthon thrust (LAT) (Fig. 3g) carrying the allochthonous units piggy-back and using the weak Silurian carbonaceous slates to detach [26,39].
The LFT moved after 346 Ma, which was the age of migmatization in one of the basal units [2]. The S2 cleavage in the underlying Schistose Domain, dated at 340 Ma [21], was developed during emplacement of the LFT. The LAT is constrained between 340 Ma and 323 ± 11 or 317 ± 15 Ma, age of crosscutting Variscan granitoids [16,55]. The Mondoñedo basal thrust (MBT) (Fig. 3h) developed subsequently in the autochthon, and more thrusts developed progressively toward the foreland.
7.3 Extensional collapse, removal of the orogenic roots, and late shortening
Crustal thickening was followed by thermal relaxation causing increase in temperature and partial melting, and facilitating viscous flow that accommodated gravitational extension of the whole crust (Fig. 3i). Extension is marked by a pervasive flat-lying foliation, by thinning and disappearance of previous metamorphic zones at ductile detachments, and by gneiss domes [11,25]. Kinematic criteria indicate a noncoaxial component of deformation and extension normal, oblique and parallel to the orogenic trend. High-T and low-P rocks crop out in the domes, accompanied by many Variscan granitoids.
Extension, mid- and lower crustal flow and partial melting were responsible for the disappearance of the orogenic roots and the establishment of a new Moho. The amount of extension was important, given the high strains registered and the abundance of normal detachments. The apparently large displacement shown by the thrusts involved in the suture, ca. 200 km, may, to a large extent, be a consequence of orogenic extension [17], being less important in origin (Fig. 3h). The main phase of collapse and extension occurred between 320 and 310 Ma, which is the age of most of the synkinematic granitoids [28].
Extensional collapse was late but not postorogenic, as extension in the internal zones was coeval with the development between 312 and 300 Ma of a thin-skinned foreland thrust belt in the CZ (Fig. 3j), where large thrusts developed piggy-back, with a total displacement of around 200 km [56]. Moreover, extensional domes in the internal zones were overprinted by upright folds: these late upright folds (D3) interfere with early recumbent folds and fold the regional metamorphic isograds. The largest folds nucleated in domes and basins developed previously, although the Lugo (LD) (Fig. 3k) and Sanabria domes developed later [24,48]. Folds are associated with strike–slip ductile shear zones which moved at between 315 and 305 Ma [75], and displaced the suture [48], further contributing to its rootless character.
8 Summary of terrane dispersal, accretion and continental collision
The European Variscides are partly situated to the south of Baltica in a reconstruction (Fig. 1) that postdates Carboniferous dextral strike–slip movement between Laurussia and Gondwana [48,74]. Before dextral motion, the Variscan belt was situated more to the upper right corner of Fig. 1 lying perhaps entirely to the South of Baltica. This implies that the Variscides formed by collision of northern Gondwana with eastern Avalonia and Baltica, and further suggests that their ophiolitic units are relicts of the eastern branch of the Rheic Ocean, also that the upper allochthonous units were accreted to Avalonia and Baltica during the closure of the Tornquist Ocean.
A cartoon map reconstruction of continents and oceans during the Paleozoic, based on Winchester et al. [79] is shown on Fig. 4. Detrital zircon age populations in Neoproterozoic and Paleozoic sediments of NW Iberia are typical of the West African craton and the Avalonian–Cadomian–Pan-African belts, but also include a Mesoproterozoic population which could suggest proximity to the Amazonian craton [29], or NE Africa [38]. An oceanic terrane dated at 1120–1170 Ma is involved in the NW Iberian suture [68], suggesting the presence of Mesoproterozoic rocks in the northern margin of Gondwana prior to Cambro-Ordovician terrane dispersal. The peri-Gondwana arc preserved in the upper allochthonous units has been placed in the periphery of NW Africa, halfway between the two possible known sources of Mesoproterozoic zircons, in agreement with the apparent lack of zircons of that age [31]. The upper units would represent the lateral equivalent, or a continuation of Avalonia and the Gander Arc where, as in the upper units, separation took place around the Cambro-Ordovician boundary [48,79] and accretion occurred in the Early Silurian [19].

Distribution of continental masses during the Paleozoic, showing the suggested paleopositions of the European Variscides and the ensialic arc preserved in the upper allochthonous units. Modified after Gómez Barreiro et al. and Winchester et al. [38,79].
Fig. 4. Distribution de masses continentales pendant le Paléozoïque montrant les positions suggérées du Variscides européennes et de l’arc préservé dans les unités allochtones supérieures. D’après Gómez Barreiro et al. et Winchester et al. [38,79], modifié.
Once separated, the ensialic arc drifted away from Gondwana during the Ordovician, leaving behind the Rheic Ocean, and was in collision with Laurussia during Silurian to Early Devonian times. Although this lithospheric fragment drifted away from Gondwana and later became emplaced on it, the site of derivation probably differs from that of emplacement (Fig. 4). This may explain inconsistencies in the ages of magmatism and metamorphism as rifting, drifting, accretion and collision were likely to have been diachronous along the continental margins.
9 Discussion: importance of the Rheic Ocean
Pre-Variscan and Variscan orogenic events in the Iberian Massif are comparable with those in the Armorican, Massif Central and Bohemian massifs. However, the importance of the Rheic Ocean has not always been recognized in central Europe, and other oceanic realms separating Gondwana-derived microplates have been proposed to account for ophiolites and subduction-related metamorphism. The NW Iberian Peninsula is less affected by large strike–slip shear zones, permitting a qualitative palinspastic reconstruction that yields a simplified interpretation valid for this part of the Variscan belt, and which can shed light on the understanding of other areas.
According to sedimentary and faunal evidence [63], and on the evidence of inherited zircon population ages [47], the Iberian allotochthon was always part of the northern margin of Gondwana. Furthermore, the NW Iberian suture corresponds to a single major ocean, the Rheic, because:
- • the protolith ages of the involved ophiolitic units span a time interval of 105 Ma, similar to that of existence of the Rheic Ocean [79];
- • arc-related magmatism and metamorphism is 125 Ma older than oceanic closure, which supports the Rheic option, because the development of an arc inside the Rheic Ocean, when it was starting to open, is unrealistic. Arc development during the Ordovician was widespread in the Iapetus Ocean [78,79], and correlation of the upper units with an arc occurring outside of Gondwana and facing the Iapetus or Tornquist oceans is much more reasonable (Fig. 3b).
Our model shows the influence of the Rheic Ocean in the Paleozoic evolution of Iberia, an influence that can be extended to other realms of the Variscan belt. For instance, Woodcock et al. [80] link the Acadian deformation (400–390 Ma) in England and Wales, with subduction of the Rheic Ocean to the north beneath the Avalonian lithosphere. This is coherent with our hypothesis that the arrival of the island arc to the margin of Laurussia was followed by its accretion and partial subduction, dated at 425–390 Ma in the high-P and high-T upper units (Fig. 3c). Woodcock et al. [80] even suggest that the upper allochthonous units of NW Iberia are the best candidate for the missing segment of the Laurussian margin of England and Wales. However, discrepancies arise concerning the type of subduction of the Rheic lithosphere at 400-380 Ma, either flat subduction beneath Laurussia [80], or intraoceanic subduction (Fig. 3d). Assuming large strike–slip displacements between Laurussia and Gondwana [48,80], these differences simply represent slightly different scenarios taking place in areas separated by hundreds or thousands of kilometres.
Acknowledgements
This work was funded by the projects CGL2004-04306-C02/BTE and CGL2007-65338-C02/BTE of the Spanish Ministerio de Educación y Ciencia, and is a contribution to the IGCP 497: The Rheic Ocean: Its Origin, Evolution and Correlatives. Two reviewers, J.-P. Burg and M. Sintubin, have contributed to improve the manuscript, and their efforts are kindly acknowledged.