1 Introduction
A wide range of terrestrial and mainly marine climate proxies demonstrates that in the Late Cretaceous world greenhouse strong warm/humid and cool/arid fluctuations occurred (Gallagher et al., 2008). The Palaeocene shows a global warming trend, culminating in the abrupt thermal increase at the Palaeocene-Eocene boundary, which represents the warmest period during the entire Cenozoic era (Gibbs et al., 2006).
According to Thiry (2000), intense weathering has left a deep imprint (such as bauxites, laterites, ferricretes) on the world Cretaceous–Early Tertiary palaeosurfaces. Palaeoalterites rich in iron (known as ferricretes, ferruginous duricrusts, ironstones and, in French, paleocuirasses ferrugineuses) are usually interpreted as palaeoexposure crusts developed in a tropical climate.
Several small outcrops of Upper Cretaceous–Lower Palaeocene ferruginous palaeoalterites have been recently recognized in Sardinia (Murru et al., 2003). The two outcrops of Guardia Pisano and Nuxis (Fig. 1) contain a pisolithic horizon.
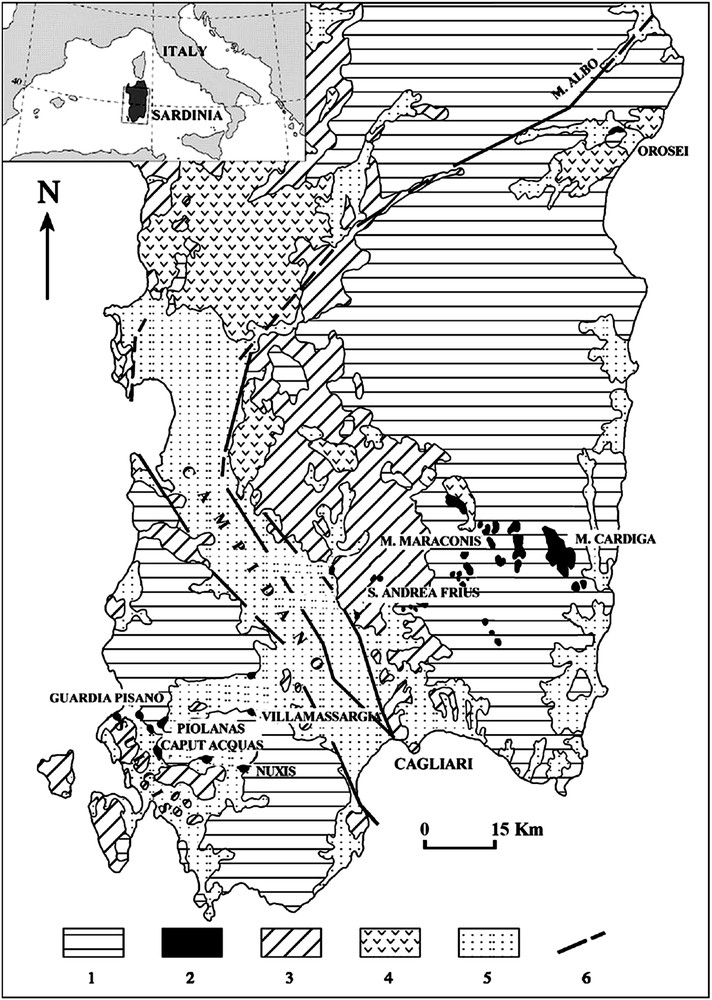
Geological sketch map of southern Sardinia: 1: Palaeozoic-Mesozoic basement; 2: Uppermost Maastrichtian, Palaeocene-Eocene outcrops; 3: Oligo-Miocene volcanic and sedimentary deposits; 4: Plio-Quaternary basalts; 5: Quaternary sediments; 6: faults.
Carte géologique simplifiée de la Sardaigne méridionale : 1 : socle paléozoïque-mésozoïque ; 2 : affleurements du Maastrichtien sommital et du Paléocène-Eocène ; 3 : dépôts sédimentaires et volcaniques de l’Oligocène-Miocène ; 4 : basaltes plio-quaternaires ; 5 : sédiments quaternaires ; 6 failles.
In southern France, ferricrete levels with pisolithic structures, ascribed to the Upper Cretaceous–Palaeocene, have been recognized and described in a number of places: Senas near Marseille-Provence (Cojan, 1989; Saurel et al., 1976) and Charentes in northern Aquitaine (Gourdon-Platel, 1980). The cuirasse gravillonaire of Senas correlates well with one weathering surface of regional extension (Cojan, 1989), whose 68 My age is supported by magnetostratigraphy (Cojan et al., 2000).
Geological, geophysical and palaeontological evidences (Cherchi et al., 2008) show that the Sardinian-Corsican block formed part of the southern edge of the European plate until the Aquitanian-Langhian spreading of the Provençal basin, with anticlockwise rotation of the block to its modern position. Therefore, during the emersion phase of the Late Cretaceous–Early Palaeocene, Sardinia shared the same palaeoclimatic and palaeoenvironmental conditions of the Pyrenean-Provençal region.
The purpose of this article is to study the Sardinian pisolithic deposits, focusing on their palaeoenvironmental and palaeogeographic significance in comparison with the similar ones in southern France. Furthermore, considering that the authigenic iron minerals form primarily through biological processes involving bacteria, and that the biogenic iron-rich minerals form in extremely varied environmental conditions (Ehrlich, 2002; Fortin and Langley, 2005; Konhauser, 1998; Préat et al., 2000), the role of iron bacteria in the formation of the Sardinian iron pisoliths has also been investigated.
2 Materials and methods
Two outcrops were studied in the field (Fig. 1): for each outcrop, a main vertical profile was sampled, along with numerous other lateral levels for a total of about 100 sampling points. Colours were determined according to the Munsell Soil Color Charts (2000). Thin sections of the collected materials were examined. Microscopic and submicroscopic observations were made on thin sections and broken pieces and single laminae using a SEM FEI Quanta 200. Petrographical, mineralogical and geochemical analyses were performed on the pisolithic concretions and the bulk rock. Chemical composition was determined by XRF analysis with three different measurements; mineralogical composition was performed by X-ray diffraction (XRD) using a Panalytical X’pert Pro diffractometer, with Cu-Kα1 radiation generated at 40 kV and 20 mA. XRD results were also confirmed by SEM/EDS analyses.
3 The outcrops
3.1 Nuxis
Sampling was carried out in the disused Bacchera quarry, situated about 200 m south of the hamlet Is Ollargius and about 1000 m southwest of Nuxis village (Fig. 1). The Cambrian basement of the quarry, about 18 m deep, is composed of dolomitic limestones mineralized by barytine and highly karstified. The karstic cavities are filled with red-brown ferruginous deposits, about 8 m thick, overlain by siltstones with intercalated arenaceous-conglomeratic levels of the Middle-Upper Eocene continental Cixerri Formation.
Three main facies are recognizable in the ferruginous sequence (NX mark for the samples): (a) dusky red (7.5R 3/3), massive, structureless, very well indurated horizon about 2 m thick; the ferruginous plasma contains small (0.5–10 mm) and relatively few (less than 10% by volume) angular fragments of barytine and small subspherical nodules, with sizes ranging from 1.0 to 3.0 mm (sample NX2a); (b) dusky red horizon (7.5R 3/4) chaotically textured, very well indurated, containing a large amount of barytine fragments (from 30 to 50% by volume) and numerous small nodules, ranging in size from 1.0 to 3–4 mm (NX2b1 bottom – NXb2 top); (c) pisolithic ferricrete, dusky red in colour (7.5R 3/3), not indurated; size of pisoliths ranging from 1.5 to 10 mm (NX2c); rare (less than 5% in volume), but large (10–40 mm) fragments of barytine; thickness about 3 m.
3.2 Guardia Pisano
The outcrop is located in the Guardia Pisano hills about 500 m northwest of Bacu Abis village (Fig. 1). The substratum is composed of a Lower Permian (Barca and Costamagna, 2006) continental sedimentary complex (siltstones, sandstones, conglomerates, volcanic deposits). The ferruginous palaeoalterite resting on the Permian substratum is capped by marine coastal sediments with miliolids, referred to the Upper Thanetian-Lower Ilerdian. Five levels can be observed along the main sampled section (GP mark for the samples): 1: Permian basement, composed chiefly of highly altered gray (N6/) metasiltstones, rich in quartz, barite and goethite; 2: floodplain deposits, made up of lenticular conglomerates (up to 100 cm thick) with well rounded clasts (1/8 cm in size) of quartz, of Palaeozoic metamorphic rocks, dolomites and limestone and of weathered marly siltstones (330 cm thick), poorly indurated, with colours ranging from yellow (10YR 8/6) at the bottom (sample GP2b1) to reddish gray (7.5R 6/1) at the top (GP2b2); 3: nodular layer made up of reddish grey (7.5R 6/1) strongly weathered and poorly indurated marly clays (GP3a), with yellow spots (10YR 8/6), containing numerous, chaotically dispersed, dusky red (7.5R 3/4) ferruginous pisoliths (0.5/15 mm in size), irregularly subspherical in shape, with smooth surfaces (GP3b1 bottom - GP3b2 top); thickness of the pisolithic horizon about 150 cm; 4: very weathered and poorly indurated marly siltstones (300 cm thick) from light red (7.5R 7/6) at the bottom (GP4a) to pale yellow (5Y 8/3) at the top (GP4b); 5: floodplain to peritidal sediments (80 cm thick) made up of coarse-grained sandstones and conglomerates composed of clasts of quartz, Palaeozoic metamorphic rocks and limestones, with microcodium, and rare miliolids; the unit is overlain by limestones (5/6 m thick) rich in miliolids (Upper Thanetian-Lower Ypresian “Miliolitico” Auct.).
4 Results
4.1 Thin sections (transmitted light and SEM/EDS)
Observation of thin sections of the pisolithic concretions of the Nuxis outcrop under transmitted light revealed their concentric structure and the presence of numerous craze planes. Concentric layers are generally very thin; only the outer ones usually appear massive. SEM/EDS observation on the same thin sections shows the concentric distribution of the main mineralogical components. The nucleus generally contains iron oxides (mainly hematite) and kaolinite, often in concentric layers around a small clast (frequently a quartz grain), or sometimes quite massive. The cortex is often thick and consists of layers of iron oxides-kaolinite, alternating mainly with the concentric planes (30/40 μm in thickness) of an often very complex system of crazes filled with microcrystalline barite precipitates (Fig. 2A).
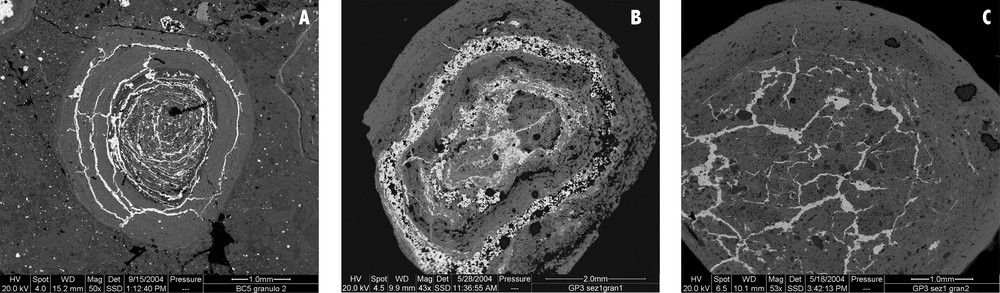
Thin sections of pisoliths: A. SEM image of Nuxis, showing the nucleus and the thick kaolinitic-Fe oxides cortex (dark), traversed by a craze plane system, filled by barite (white). Some radial voids continue in the skrews of the rock, rich in quartz grains and substratum rock fragments. B. SEM image of Guardia Pisano: the kaolinitic nucleus is covered with a thick massive goethitic cortex. The white concentric layers, irregularly alternating with the dark ones, are composed of barite. C. SEM image of Guardia Pisano showing the thick goethitic cortex traversed by rare radial cracks; the inner nucleus presents a septarian-like craze plane system, filled by barite. The largest mottles (right side) show a thin iron coating.
Lames minces des pisolithes: A. Photo au MEB d’un pisolithe Nuxis présentant le noyau formé de couches de kaolinite et d’oxydes de Fe (noir), entrecoupé par un système de vides radiaux rempli de baryte (blanc). Certains vides radiaux continuent hors du noyau et sont remplis de petits grains de quartz et de fragments du substrat. B. Photo au MEB d’un pisolithe de Guardia Pisano ; le noyau kaolinitique est recouvert d’une épaisse écorce goethitique massive. Les couches concentriques blanches qui alternent irrégulièrement avec les noires sont constituées de baryte. C. Photo au MEB d’un pisolithe de Guardia Pisano présentant l’épaisse écorce goethitique entre coupée de rares vides radiaux ; le noyau présente un système de vides en forme de septarias remplies de baryte. Les plus grandes taches noires visibles sur le côté droit présentent un fin revêtement de fer.
In the pisolithic concretions of the Guardia Pisano outcrop, the concentric structure is often missing in the central part (nucleus), which is characterized by a large number of craze planes. Embayed quartz grains and carbonate rock fragments are sometimes frequent. SEM observation shows the fine layered cortex to be composed of goethite surrounding a kaolinite nucleus. Moreover, an original concentric layered structure of the nucleus is also sometimes observed, made up of kaolinite layers more or less enriched in iron oxides, often encircling a central quartz granule (Fig. 2B). The quartz grains usually exhibit embayed morphologies. Sometimes, an outer thin cortex, rich in small quartz granules (40–500 μm), is composed of a layer of kaolinite/illite. Many pisoliths show large concentric crazy planes filled with calcite, or, less frequently, with barite (Fig. 2C).
4.2 Micromorphology of the internal layers (SEM/EDS)
SEM analysis of the internal surfaces of the thin concentric layers often reveals a well-developed microcrystalline structure and micromorphologies evoking biological activity. The thin laminae of the goethitic/hematitic pisolithic cortex consist chiefly of small-sized chestnut-like minerals, forming irregular chains, which often constitute sheety aggregates covering the substratum (Fig. 3).
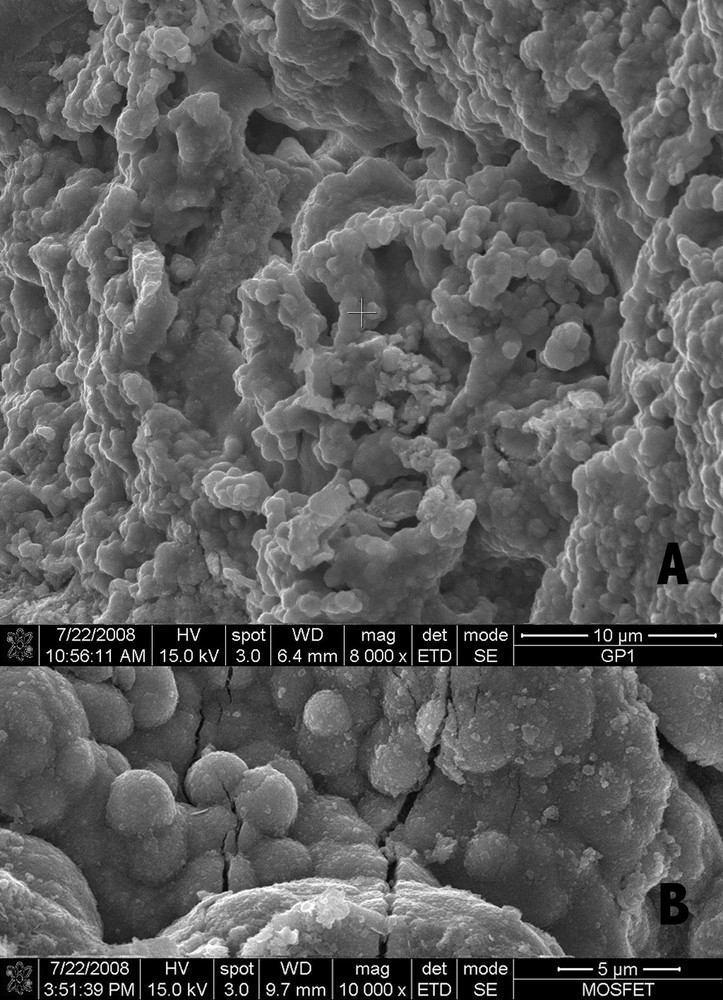
Surface of a thin internal layer (A) of the goethitic/hematitic cortex in a pisolith from Nuxis showing irregular chains of chestnut-like bodies (centre) and a continuous layer (B) of thin ball-like bodies (sides). SEM images.
Partie superficielle (A) d’une couche interne de l’écorce de goethite-hématite d’un pisolithe de Nuxis présentant des structures irrégulières de corps arrondis en forme de chaîne au centre et en couches ininterrompues sur les côtés (B). Photos au MEB.
Ferruginous coatings, which can be associated with bacteria activity, are usually observed. These exhibit a variety of morphologies: irregular filamentous reticulum, with irregularly curved filaments approximately 20 μm long and 2 μm wide (Fig. 4); twisted, slightly flattened stalks, 10 μm long and 2,5 μm wide (Fig. 5); elongated, straight or gentle curved bodies, mainly with round empty cross-section, 10 μm long and 2 μm wide (Fig. 6); elongated, gently curved, flattened segmented elements, 15 μm long and 3 μm wide (Fig. 7).
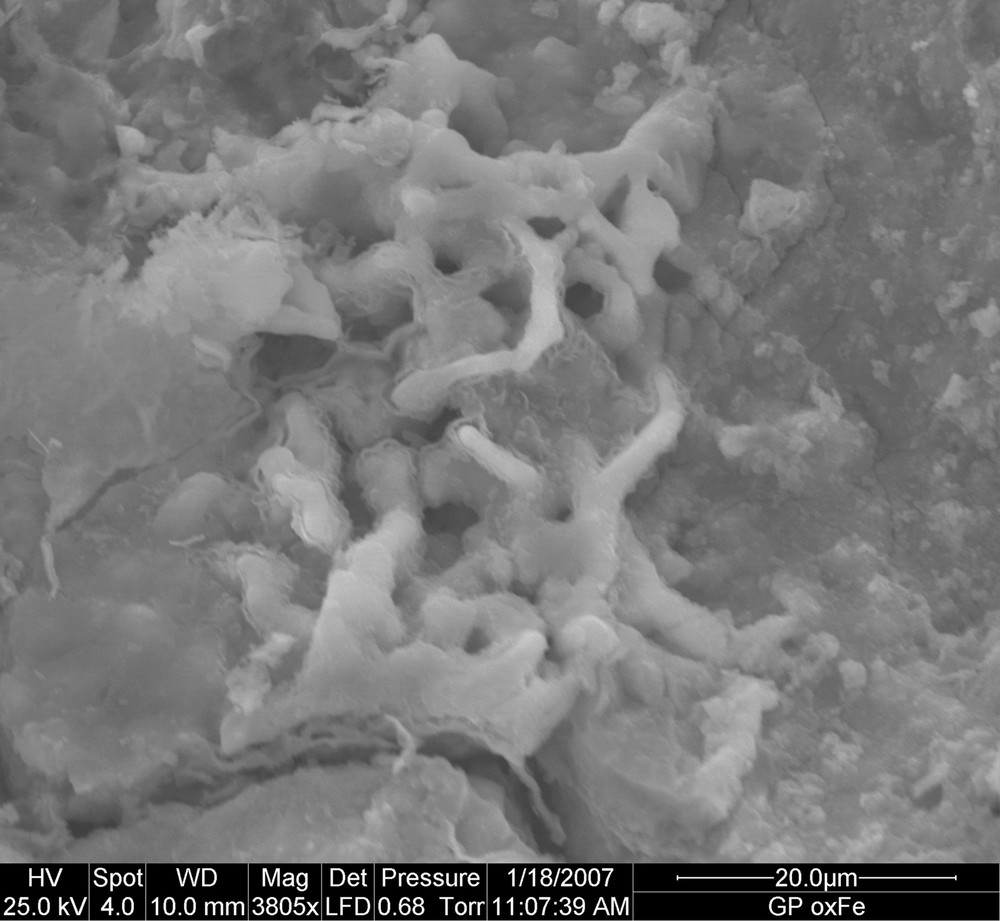
Irregular filamentous network on surface of thin lamina of the goethitic cortex of a pisolith from Guardia Pisano. SEM image.
Réseau filamenteux irrégulier observable sur une surface laminaire de l’écorce goethitique d’un pisolithe de Guardia Pisano. Photo au MEB.
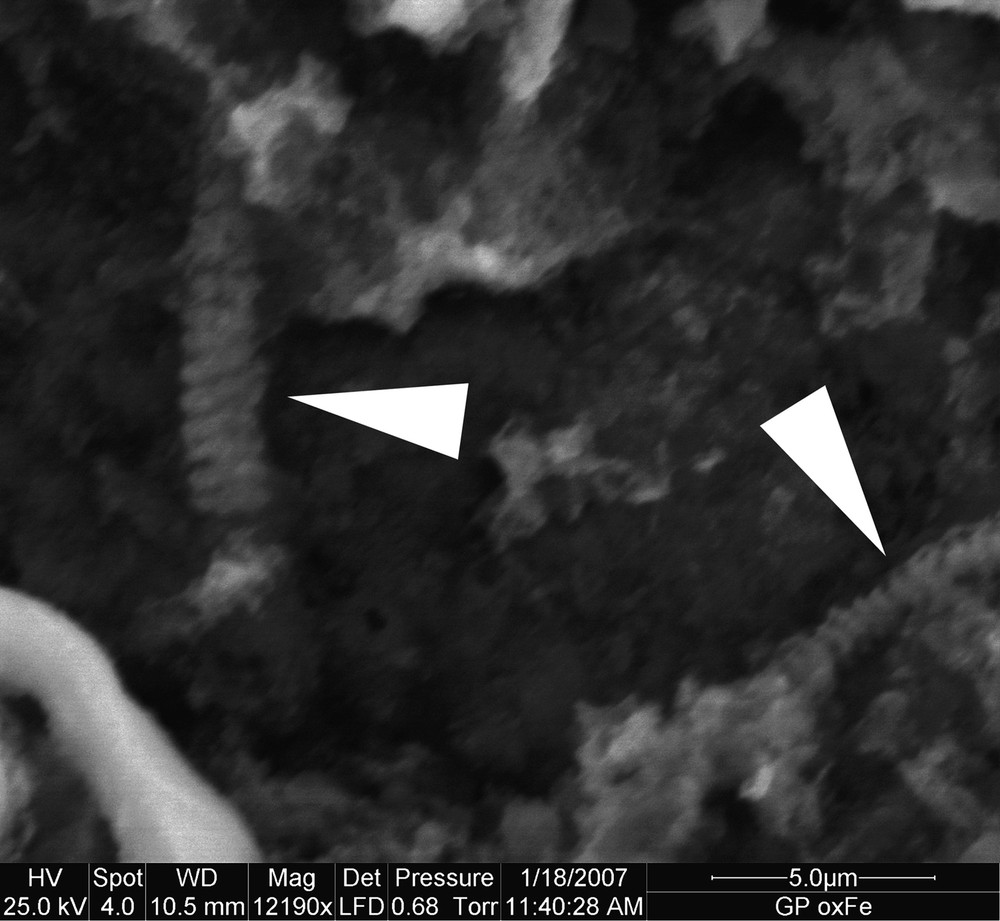
Two twisted, slightly flattened stalks (arrows) on surface of a thin lamina of the goethitic cortex of a pisolith from Guardia Pisano. SEM image.
Sur la surface d’une lamelle de l’écorce goethitique d’un pisolithe de Guardia Pisano, sont visibles deux spirales (flèches) hélicoïdales aplaties le long de l’axe. Photo au MEB.
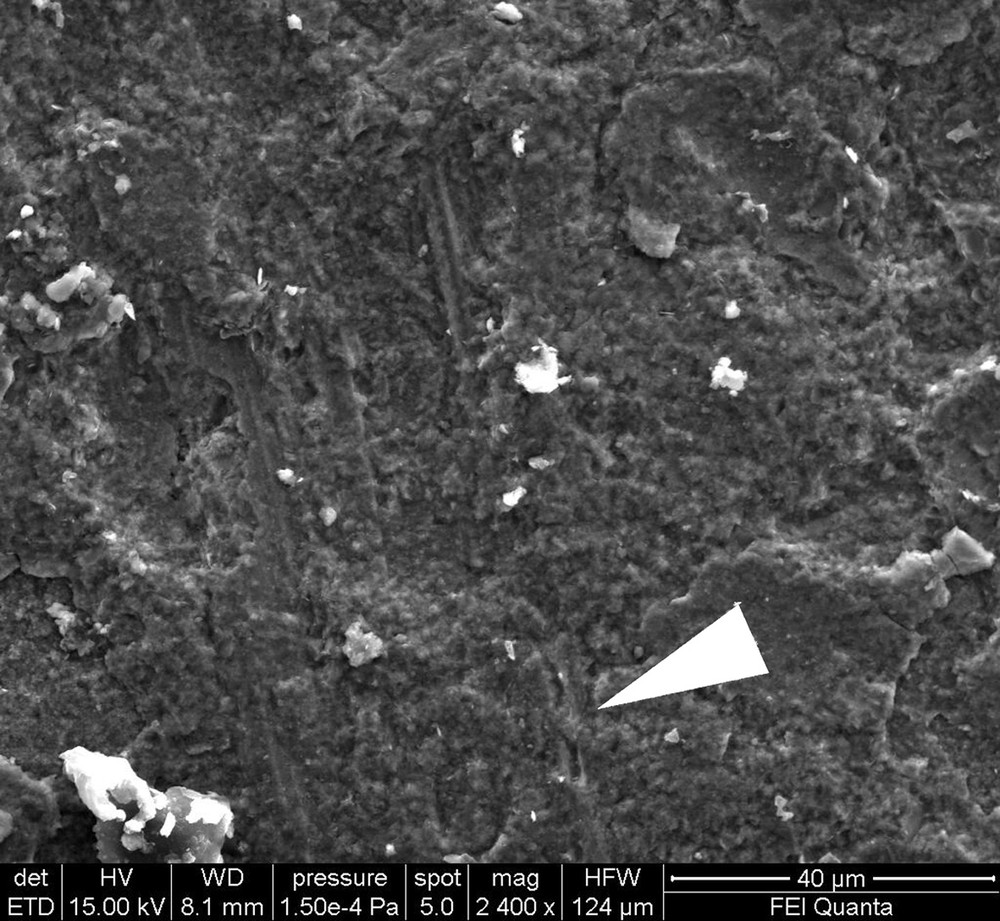
Elongated, right sheaths, mainly rounded cross-section and empty (arrow), lying side by side on surface of a thin lamina of the goethitic/hematitic cortex of a pisolith from Nuxis. SEM image.
Sur la surface d’une lamelle de l’écorce goethitique d’un pisolithe de Nuxis, il est possible de voir des revêtements allongés, disposés transversalement par rapport à la lamelle qui apparaissent arrondis et vides (flèche) en section. Photo au MEB.
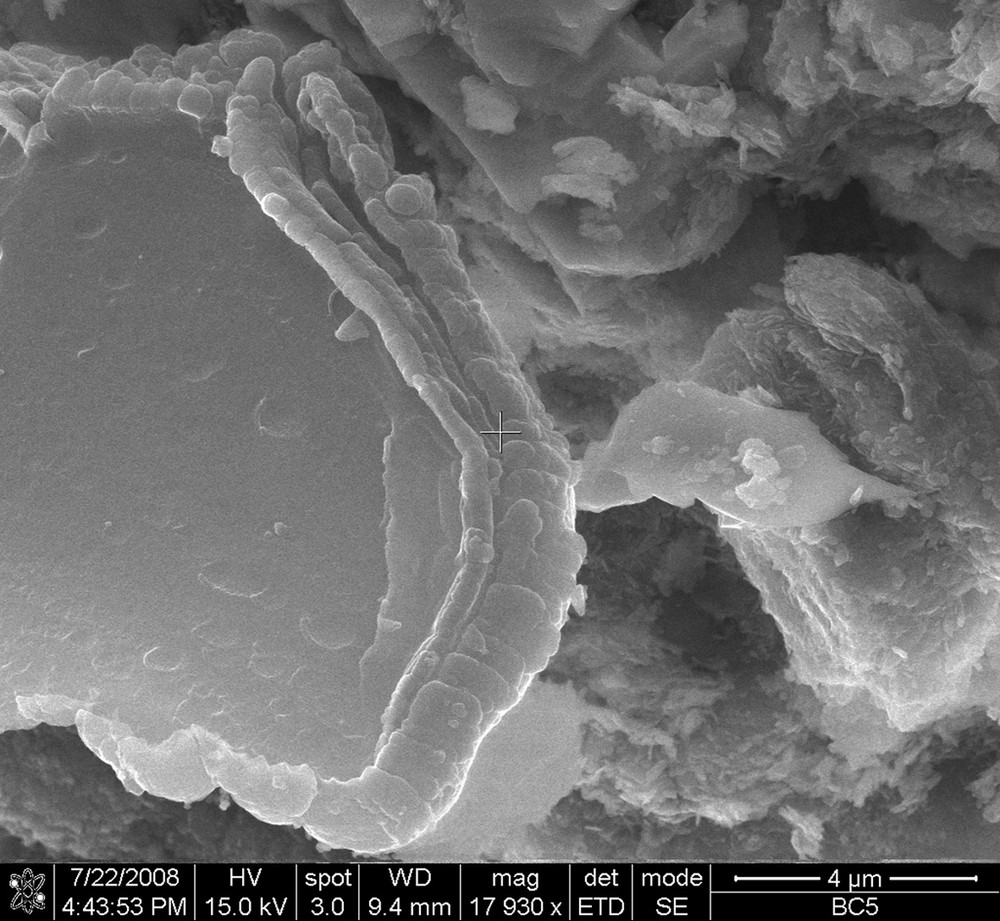
Elongated, gently curved, flattened segmented bodies on surface of a thin lamina of the goethitic/hematitic cortex of a pisolith from Nuxis. SEM image.
Corps allongés, segmentés, faiblement aplatis observables sur la surface d’une lamelle de l’écorce de goethite-hématite d’un pisolithe de Nuxis. Photo au MEB.
4.3 Mineralogy and geochemistry
The results of analyses performed mainly on the pisolithic concretions, but also on the bulk rock of the Guardia Pisano outcrop, are summarised in Tables 1 and 2.
Composition minéralogique d’échantillons de Sardaigne ; les sigles des échantillons sont les mêmes que ceux utilisés dans le troisième paragraphe.
Quartz | Calcite | Dolomite/Ankerite | Kaolinite | Illite | Hematite | Goethite | Barite | |
GP2b1 | ++ | ++++ | − | + | + | − | + | − |
GP2b2 | ++ | ++++ | − | + | + | − | − | − |
GP3a | ++ | ++++ | − | + | ++ | − | − | − |
GP3b1a | +++ | + | − | ++ | + | − | ++++ | + |
GP3b2a | +++ | ++ | + | + | + | − | ++++ | − |
GP4a | +++ | ++++ | − | + | + | − | − | − |
GP4b | ++ | ++++ | +++ | + | + | − | − | − |
NX2aa | ++ | + | − | +++ | − | +++ | ++ | +++ |
NX2b1a | ++ | − | − | +++ | − | +++ | ++ | ++++ |
NX2b2a | ++ | − | − | +++ | − | +++ | ++ | +++ |
NX2ca | + | + | − | ++ | − | +++ | ++ | ++++ |
a Pisoliths.
Composition chimique d’échantillons de Sardaigne et de France (Bassin de Provence) ; les sigles des échantillons sont les mêmes que ceux utilisés dans le troisième paragraphe.
Guardia Pisano | Nuxis | France | ||||||||||
GP2b1 | GP2b2 | GP3a | GP3b1 a | GP3b2 a | GP4a | GP4b | NX2a a | NX2b1 a | NX2b2 a | NX2c a | Range | |
wt% | wt% | wt% | ||||||||||
SiO2 | 22.13 | 13.35 | 17.72 | 25.28 | 22.74 | 21.34 | 13.94 | 27.71 | 25.21 | 26.15 | 10.64 | 11.7–14.4 |
TiO2 | 0.39 | 0.26 | 0.28 | 0.40 | 0.43 | 0.31 | 0.28 | 0.99 | 0.87 | 0.94 | 0.51 | n.r. |
Al2O3 | 7.05 | 4.59 | 5.12 | 9.85 | 8.70 | 4.49 | 4.21 | 18.23 | 17.53 | 19.34 | 7.98 | 8.5–11.5 |
Fe2O3 | 4.68 | 1.09 | 1.57 | 48.85 | 48.50 | 0.95 | 1.73 | 23.32 | 23.04 | 24.81 | 20.94 | 33.8–56.2 |
MnO | < 0.01 | < 0.01 | < 0.01 | 0.24 | 0.26 | < 0.01 | 0.06 | 0.23 | 0.13 | 0.19 | 0.24 | n.r. |
MgO | 0.41 | 0.50 | 0.85 | 0.25 | 0.71 | 0.46 | 8.89 | 0.14 | 0.09 | 0.09 | 0.07 | n.r. |
CaO | 33.60 | 42.82 | 38.82 | 1.17 | 3.84 | 39.33 | 32.40 | 2.67 | 0.06 | 0.13 | 1.94 | 0.8–12.3 |
Na2O | 0.01 | 0.01 | 0.10 | 0.01 | 0.01 | 0.01 | 0.01 | < 0.01 | 0.01 | < 0.01 | 0.21 | n.r. |
K2O | 0.74 | 0.55 | 0.89 | 0.85 | 0.79 | 0.45 | 0.58 | 0.45 | 0.20 | 0.22 | 0.14 | n.r. |
P2O5 | 0.03 | < 0.01 | 0.02 | 0.01 | 0.01 | < 0.01 | < 0.01 | 0.04 | 0.05 | 0.07 | 0.03 | n.r. |
ZnO | 0.04 | < 0.01 | 0.02 | 0.23 | 0.21 | 0.03 | 0.03 | < 0.01 | < 0.01 | <0.01 | < 0.01 | n.r. |
BaO | < 0.20 | < 0.20 | < 0.20 | 0.98 | < 0.20 | < 0.20 | < 0.20 | 13.41 | 19.38 | 15.73 | 32.30 | n.r. |
SO3 | 0.08 | 0.13 | 0.18 | 0.45 | 0.10 | 0.06 | 0.22 | 4.60 | 7.49 | 5.54 | 17.07 | n.r. |
LOI | 30.82 | 36.52 | 32.80 | 11.05 | 13.03 | 32.49 | 37.42 | 7.99 | 5.71 | 6.59 | 7.23 | n.r. |
TOT | 99.62 | 99.97 | 99.78 | 99.31 | 98.42 | 99.82 | 99.90 | 99.79 | 99.78 | 99.81 | 99.30 |
a Pisoliths.
The Nuxis concretions (samples NX2a, NX2b1, NX2b2 and NX2c) are composed chiefly of barite, hematite, kaolinite and subordinate goethite and quartz. The Guardia Pisano concretions (samples GP3b1 and GP3b2) are composed predominantly of goethite and quartz with minor calcite, kaolinite and illite; the host rock (samples GP2b1, GP2b2, GP3a and GP4a) is composed chiefly of calcite with abundant quartz and minor amount of kaolinite and illite; only in the sample GP4b dolomite/ankerite is abundant. Barite is just present in the GP3b1 sample.
As for chemical composition, the Nuxis concretions are composed chiefly of Fe2O3 (24.8/20.9 wt %), Al2O3 (19.3/7.9%), BaO (32.3/13.4%) and SiO2 (27.7/10.6%), with subordinate CaO (2.6/0.06%). In the Guardia Pisano concretions Fe2O3 (48.8/48.5%) prevails, followed by SiO2 (25.2/22.7%) and Al2O3 (9.8/8.7%), with subordinate BaO (0.98/< 0.20%) and CaO (3.8/1.1%). By contrast the analysed host rock is composed chiefly of CaO (42.8/32.4%) and SiO2 (22.1/13.3%), with subordinate Fe2O3 (4.6/0.9%) and Al2O3 (7.0/4.2%); BaO is usually < 0.20%.
5 Discussion
5.1 Pisolith formation
The genesis of the pisolithic concretions in the Nuxis and Guardia Pisano deposits can be best explained by taking as a reference the succession of occurred events, which was interpreted from the bulk of field and laboratory data, matched with the literature (Driessen et al., 2001; Meyer, 1977; Nahon, 1986; Nahon et al., 1977; Pédro, 1968; Tardy and Nahon, 1985). The well rounded outer surface of the pisoliths, consistent with their internal fabric, indicates their in situ formation in both outcrops and the lack of transport.
At Nuxis, in warm, humid tropical climate, a lateritic cover formed on the karstified and drained Palaeozoic carbonate basement. A seasonally contrasting climate favoured the concentration of the iron oxides, which formed a massive and well-indurated layer, partly preserved at the bottom of the soil sequence in the Bacchera quarry (facies a). The overlying brecciated iron layer (facies b) indicates a subsequent partial fragmentation of the indurated iron layer, probably by leaching of iron in a more humid climate. The topmost pisolithic layer (facies c) formed by re-hydratation of hematite, producing rings of hematite/goethite around the kaolinite-hematite nuclei and fragments or forming a cortex around sparse residual quartz granules. At the end of this secondary degradation process, cohesion diminished and the layer became less indurated; the pisoliths that formed around a kaolinitic-hematitic or quartz nucleus with a more or less thick hematitic/goethitic cortex, tended to detach from the surrounding plasma, forming a gritty facies on the outer surface (facies c). Desiccation processes, probably coeval with or subsequent to the cortication processes, produced crazes in the hardpan and in the single pisoliths, rapidly filled mainly with precipitated barite and, to lesser extent, calcite.
In the Guardia Pisano outcrop, the fresh parent rock is a marly siltstone. The mottled surface layer of the Permian substratum exhibits white and red blotches and contains kaolinite, hematite and goethite. The lateritization process, interrupted by coarse and fine-grained floodplain deposits, continued to involve these sediments, as testified by the abundant yellow and red mottles. The poor drainage of the marly layer influenced iron mobilization. Nodulation of the kaolinite-hematite mottles and their goethitic cortification were discontinuous, with the formation of scattered concretions in the top marly layer.
In modern tropical soils, the soil layers enriched in iron and poor in organic matter are defined as plinthitic horizons (IUSS Working Group WRB, 2007). These include: plinthite, for a subsuperficial level composed of a kaolinitic clay, with abundant iron and little organic matter, containing quartz and other minerals; petroplinthite, for an indurated ferruginous level, somewhat fractured, very stable and durable, which forms mainly by irreversible transformation of the plinthic level or, directly, in the soil; pisoplinthite, for a level containing iron-rich subspherical concretions, iron-cemented, which mainly forms by transformation of plinthic levels. At Nuxis, the main features of the two lowest layers (facies a and b) indicate the presence of petroplinthic horizons, while the upper layer (facies c) fits the requirements for the pisoplinthic horizon. The pisolithic levels at Guardia Pisano can be referred to a former pisoplinthite in the area.
5.2 Biological influence
In present-day soils, bacteria are mainly studied in iron deposits occurring in field drains; the presence of different kinds of bacteria mainly depends on the pH (Houot and Berthelin, 1992; Wheatley, 1988); at pH around neutrality, sheathed, budding and stalked bacteria, most often Gallionella (that exhibits the typical “twisted ribbon” morphology), Leptothrix and Sphaerotilus, are found associated with iron deposits. The most common microaerophilic bacteria involved in iron oxidation are Gallionella and Leptothrix (Emerson and Weiss, 2004). These closely associated bacteria can produce iron oxides under fluctuating environmental conditions (Schieber and Glamoclija, 2007). The activity of iron-oxidizing bacteria may also be an important factor in the weathering processes ongoing in regolith material below the rooting zone (Buss et al., 2005). In the reducing stagnant pools at the Gunma iron mine (Japan), coccoid-type bacteria induce Fe-S-(P) precipitates around the bacteria bodies (Akai et al., 1999).
The thin goethite/hematite layers of the pisoliths in both Nuxis and Guardia Pisano outcrops exhibit a nanocrystalline structure, composed of aggregates of small-ball like grains (Fig. 3), which are very similar in morphology and size to those forming the thin-banded goethite deposits at Gunma iron mine. Akai et al. (1999) demonstrated that these goethite nanocrystalline aggregates are very similar to the coatings of the coccoid-type bacteria today acting mainly in the stagnant pools of the mine. The coccoid-type bacteria iron coatings probably acted as nucleation sites for further inorganic iron mineralization giving rise to an overgrowth of the direct precipitates around bacterial bodies. Biogenic iron minerals form either as internal or external precipitates (Ehrlich, 2002; Konhauser, 1998; Préat et al., 2000). Under oxic conditions, epicellular iron hydroxide precipitation can be actively or passively promoted by acidophilic and neutrophilic bacteria. In the first instance, the metabolic activity of iron-oxidizing bacteria can induce ferric hydroxide precipitation as a secondary by-product. Alternatively, iron hydroxides can form passively through the binding of dissolved ferric species to negatively charged polymers or when soluble ferrous iron spontaneously reacts with dissolved oxygen to precipitate as ferric hydroxide on bacterial bodies acting as nucleation sites.
Furthermore, SEM analysis on the inner surfaces of the goethite/hematite coatings, mainly on the poorly microcrystalline ones, revealed the presence of filamentous bodies resembling the twisted stalks of Gallionella (Fig. 5) and the cylindrical ones of the modern Leptothrix (Fig. 6). Unbranched, septate, flattened filaments showing partitions in more than one plane (Fig. 7), which presumably show multiple sporulations in their termination, are rarely recognized. The modern non-phothosyntetic iron microorganism Crenotrix, common in dark waters, shows multiple sporulation by bipartition in more than one plane (Kolk, 1938; Völker et al., 1977). The alternation of a predominance of Leptothrix-like microaerophilic bacteria with the coccoid-type, which presumably required more stagnant waters, could indicate fluctuating pH and oxygenation conditions during their formation.
Thus, the goethite/hematite envelope formation was induced by bacterial activity, with precipitation of ferric hydroxide, which served as a precursor for the more stable iron oxides goethite and hematite, via dissolution-reprecipitation or dehydration, respectively (Fortin and Langley, 2005; Konhauser, 1998). These two competing processes (conditions favourable for the formation of goethite are unfavourable for formation of hematite and vice versa), depend, in proportion and crystallinity, also on pH. At very high pH only goethite will form, as nanocrystals (Schwertmann and Murad, 1983).
5.3 Craze planes and barite precipitation
Pisoliths often exhibit a complex craze plane system, consisting of repeatedly broken curved planes in the inner concentric layers and of irregular radiating ones. At Nuxis, the entire rock is traversed by irregular screw planes, which usually form in soils through desiccation. Voids continuing in the screw planes are common around the pisoliths, between the pisoliths and the rock. Some of the pisoliths radial planes extend to the outer surface, continuing into the rock's screw planes. The craze plane system formed mainly through soil desiccation, during burial and compaction, by detaching of adjoined layers during dehydration shrinking. In the Guardia Pisano pisoliths there are few cracks in the thick outer goethitic cortex and the craze plane complex prevails in the central nucleus, assuming a septarian-like structure (Fig. 2C).
Screw and craze planes are usually filled mainly with barite at Nuxis and calcite, and more rarely barite, at Guardia Pisano. Barite occurs widely in the Palaeozoic basement; at Nuxis two barite-mines are today disused. The Permian basement at Guardia Pisano contains ferruginous concretions composed of goethite, barite and quartz. Barite leaching takes place chiefly in reducing environments, the degree of leaching depending on biologically mediated reactions (Bolze et al., 1974; Hanor, 2000). The reprecipitation, as authigenic barite, of the barium adsorbed by the clays occurs, in the presence of sulphate ions, in oxidizing subhumid to subarid environments (Davis et al., 1990; Khalaf and El-Sayed, 1989; Stoops and Zavaleta, 1978). Nevertheless, in the soils of Bellavista (Peru), in humid tropical environment, “the barite only appears in horizons where also iron oxihydrate nodules are present” (Stoops and Zavaleta, 1978). The hypothesis that the reprecipitation of barite may be bacterially mediated is supported by precipitation of dense barite crystals in bacterial cultures of Myxococcus xanthus, an ubiquitous heterotrophic soil microorganism (Gonzáles-Muñoz et al., 2003). The prevalence of barite in the pisoliths of Nuxis can be explained not only by a supposed higher availability of barium, but also of sulphur (Table 2).
Sometimes, the barite filled craze planes are concentrated in the outer levels, sometimes in the nucleus. The highly interconnected and intersecting radial and curved planes suggest a polyphasic process, with numerous subsequent contractions due to dehydratation, during which the increased S and Ba concentration in the waters and the capillary suction favoured penetration and precipitation. Pisolith formation, their tensile contraction and barite precipitation must have been almost contemporaneous, as indicated by the plastic response to the barite crystal formation of the infilled material (Fig. 2A).
5.4 Comparison between the ferruginous pisolithic ferricretes of France and Sardinia
The close similarity in texture (concentric accretion) and composition (kaolinite and goethite prevalence) of the Upper Cretaceous–Lower Palaeocene pisolithic ferricretes cropping out in southern France (Table 2) and in southern Sardinia (Fig. 8), their dependence on well-defined climatic conditions (moist, well-drained soils in tropical, seasonally contrasted climates), the long times required for their formation and the documented bacterial mediation (the activity of aerobic bacteria like Gallionella, Leptothrix and coccoid-type Siderocapsa has already been postulated for the formation of the Charentes pisoliths by Gourdon-Platel, 1980) suggest, be it to some extent speculatively, that these pisolithic outcrops are remnants of the same pedogenetic phase. Both Sardinian and French paleocuirasses were mainly eroded when the climate drying, the lowering of the sea level and, especially in France, the first Early Tertiary tectonic movements caused the erosion of the previously formed siderolithic palaeosols, supplying continental and marine basins with large amount of detritus (Simon-Coinçon et al., 1997). The “siderolithic” discharge lasted in western Europe from the Palaeocene to the Middle Eocene (Thiry et al., 2006).
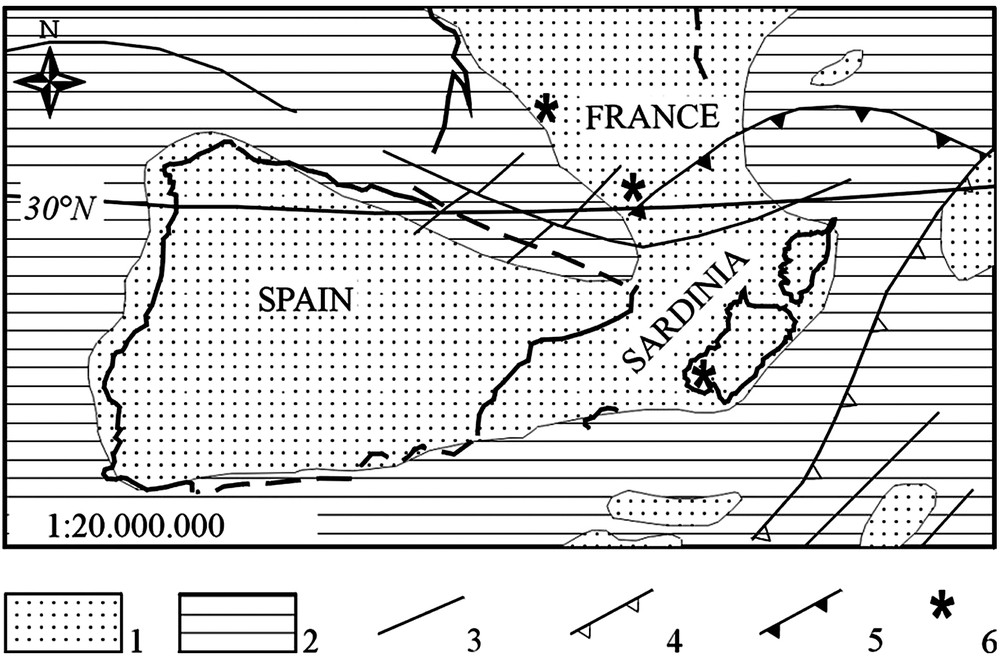
Palaeogeographic map of the Late Maastrichtian of Western Europe (after Camoin et al., 1993, modified): 1: emerged land; 2: marine areas; 3: faults; 4: oceanic subduction; 5: thrust; 6: ferruginous palaeoalterite outcrops.
Carte paléogéographique du Maastrichtien supérieur de l’Europe occidentale (d’après Camoin et al., 1993, modifiée) ; 1 : terres émergées ; 2 : aire à sédimentation marine ; 3 : faille ; 4 : subduction océanique ; 5 : charriage ; 6 : affleurements des cuirasses ferrugineuses.
Cojan and Moreau (2006) recognized subhumid conditions, with 5 arid episodes, in the terrestrial successions of the Aix-en-Provence Basin during the Late Cretaceous-Early Palaeocene. According to the Authors, these episodes, correlated with global signals, occurred in the Early Maastrichtian (episodes 1 to 3), at the end of the Cretaceous and at the beginning of the Palaeocene (episodes 4 and 5, respectively). Considering the climatic history of the Late Cretaceous–Early Palaeocene as described for the Charentes region we can tentatively place in the Late Maastrichtian, presumable after the last arid episode of the Early Maastrichtian, the warm and humid climatic phase required for the formation of plinthic palaeosols. Taking into account the long time needed for the irreversible evolution to petroplinthite and pisoplinthite (one to several million years), the arid climatic fluctuations around the K/T boundary and the increasingly dry climate during the Palaeocene could have contributed to the irreversible evolution to a petroplinthic and pisoplinthic stage of the Late Maastrichian ferruginous palaeoalterites.
6 Conclusions
The studied pisolithic ferricrete outcrops of Sardinia, composed chiefly of goethite, hematite and kaolinite, are comparable with the modern pisoplinthite, which forms mainly through the irreversible evolution of plinthite, in the warm and humid soils of the tropics under seasonally contrasting climates, probably over millions of years. The Sardinian “pisoplinthic” ferricrete can also be correlated with the pisolithic ferricretes cropping out at Senas and Charentes in southern France, as a further proxy that Sardinia belonged to the southern European margin. The Sardinian and French pisolithic ferricretes are both referable to the Late Cretaceous–Early Palaeocene. The initial “plinthic” soil probably formed already in the Late Maastrichtian. The Sardinian and French “pisoplinthic” outcrops represent the remnants of a “cuirasse” which was dismantled during the “siderolithic” discharge resulting in the erosion and redeposition in continental and marine basins, during the Palaeocene-Eocene, of the mainly kaolinitic soils of western Europe. SEM-detected traces of bacteria in the pisolithic layers prove the previously only presumed involvement of biological processes in the formation of the fossil goethitic/hematitic pisoliths, as occurs in modern humid soils. The barite filling of the crazy planes, mainly in the Nuxis pisoliths, indicates leaching of the barite-rich Hercynian basement and its reprecipitation in the tensional planes that formed in the ferruginous soil and in the pisoliths during and after their formation.
In short, the pisolithic ferricretes of Sardinia and southern France can be interpreted as the weathering product of identical warm and humid tropical climatic conditions; a tropical environment is, on the other hand, consistent with the barite leaching from the Palaeozoic substratum and with its reprecipitation into the Sardinian iron pisoliths. The close similarity in structure and composition (concentric layers and kaolinite and goethite prevalence) between Sardinian and French deposits suggests, be it to some extent speculatively, that these pisolithic outcrops are remnants of the same pedogenetic phase. Therefore, their presence provide a further evidence that Sardinia and southern France formed part of the same palaeogeographic province.
Acknowledgements
We are very grateful to François Chabaux and two anonymous reviewers for their helpful comments and suggestions that greatly helped to improve the quality of our manuscript.