1 Introduction
Several studies in tropical zones in the world enabled to better understand the formation of laterites and iron duricrusts or ferricretes (Nahon, 1986; Tardy, 1997; Tardy and Nahon, 1985). Aubert (1950) and Maignien (1958) distinguished lateritic and duricrusting phenomena. Lamotte and Rougerie (1953), Boulangé et al. (1973), Beauvais et al. (2008), and Beauvais and Chardon (2013) considered the duricrust levels of Africa like the relics of previous erosive cycles and paleoclimatic periods over the Cenozoic. Also, the humid tropical zone in Central Africa is characterized by important relics of inherited iron duricrusts or ferricretes (Beauvais and Tardy, 1991; Tardy et al., 1991). The landscape and the lateritic cover of this area also have been explained as resulting from the incision of a secondary hydrographic network and from a geochemical decay of previous ferricrete systems (Beauvais, 2009). These transformation processes were related to climatic changes implied in part by the continental drift of African plate, from the south to the north during the Cenozoic (Tardy et al., 1988). All these studies were conducted on the weathered mantle developed upon crystallophyllian and crystalline rocks.
However, if some studies were carried out on iron duricrusts developed upon sedimentary rocks in Africa and in the world (Khalid et al., 2011; Olugbenga and Olubunmi, 2012; Ramasamy et al., 2007; Zalat et al., 2012), studies are rare on iron duricrusts in the Cameroonian sedimentary basins and particularly in the Douala sub-basin. Although several studies in these basins enabled to better understand their stratigraphic and tectonic evolution (Nguene et al., 1992; SNH/UD, 2005), the studies on duricrusts from this geological context are rare.
Moreover, several biostratigraphical studies were performed in African sedimentary basins (Bankole et al., 2007; Belsky et al., 1965; Boltenhagen and Salard-Cheboldaeff, 1980; Digbehi et al., 2012; Jardine and Magloire, 1965), and particularly in Cameroonian basins (Kenfack et al., 2012; Mbesse et al., 2012; Njike Ngaha, 2004; Salard-Chebodaeff, 1977, 1990), to understand their palaeoenvironment and to suggest their relative age; but there is no study on iron duricrusts in the basins of the Gulf of Guinea that combine mineralogical, geochemical, and biostratigraphical characterizations in order to document the palaeoenvironment and the relative age of these materials.
In the present work, we report on the morphological, mineralogical and geochemical characteristics of iron duricrusts in the eastern part of the Douala sub-basin, and we study the biostratigraphy of their overlying claystones in order to reconstitute their palaeoenvironment and also to infer their relative age.
2 Study area
The study area is located at Missole I, in the eastern part of the Douala sub-basin (Cameroon, central Africa) between 3°59′–3°54′ N and 9°54′–9°58′ E (Fig. 1), in the humid equatorial climatic zone. The annual rainfall ranges between 3000 and 5000 mm, and the annual average temperature is 26 °C (Olivry, 1986). The vegetation is a dense rainforest affected by anthropic transformations (Letouzey, 1985). The geomorphology of the study area is a coastal plain with low altitudes (40–120 m). This area shows hills with flat and sharp summits, which are deeply incised by V and U shape valleys. According to the geological report of SNH/UD (2005), the relative age of Missole deposit area is Paleocene–Eocene corresponding to the N’kapa Formation.

Location and geological map of the coastal sedimentary basin in Cameroon (modified after Njike Ngaha, 1984).
3 Geological setting
The lithostratigraphy of Douala sub-basin is constituted by seven major formations related to its geodynamic and sedimentary evolution (Nguene et al., 1992; SNH/UD, 2005). (1) The syn-rift period represented by the Mundeck Formation (Aptian-Cenomanian), which lies unconformably on the Precambrian basement and consists of continental and fluvio-deltaic deposits, i.e., clays, coarse-grained sandstones, conglomerates. The post-rift sequence includes: (2) the Logbadjeck Formation (Cenomanian–Campanian), lying unconformably on the Mundeck Formation and composed of micro-conglomerates, sand, sandstone, limestone, and clay; (3) the Logbaba Formation (Maastrichtian), mainly composed of sandstone, sand and fossiliferous clay; (4) the N’kapa Formation (Paleocene–Eocene), rich in marl and clay with lenses of sand and fine to coarse-grained unconsolidated sandstone; (5) the Souellaba Formation (Oligocene) lying unconformably on N’kapa deposits and characterized by marl deposits with some interstratified lenses of channel sands; (6) the Matanda Formation (Miocene), dominated by deltaic facies interstratified with volcanoclastic layers, and (7) the Wouri Formation (Plio-Pleistocene), which consists of gravely and sandy deposits with a clayey or kaolinitic matrix.
A geological survey of the Bomkoul and Missole areas revealed different types of sediments with conglomerates, sandstones, fragments of various iron duricrusts, silts and clays (Ngon Ngon et al., 2012, 2014). Iron duricrusts were observed over various geomorphic surfaces notably on the interfluves along the Douala–Yaoundé highway and were developed upon various facies such as conglomerates, sandstones, and claystones.
4 Material and methods
The study was carried out on the Missole I outcrops from various geomorphic surfaces along the Douala–Edea highway over a distance of about 7.5 km and principally at the locality of Kendeck (3°57′–3°55′ N and 9°55′–9°56′ E) where the most representative types of iron duricrusts of the Douala sub-basin were observed. Fifteen lithofacies were described (Fig. 2, Table SM1, Supplementary data) and classified using the system of Miall (1978) and Postma (1990). A total of twenty-five iron duricrusts samples and two clayey samples were collected in the field.

Correlation log of various outcrops of Missole I (Douala sub-basin).
Various analyses were performed on the collected samples, including mineralogical, chemical, and biostratigraphic analyses.
Mineralogical surveys were carried out at the “Institut de recherches pour le développement” (IRD), France, on bulk samples of the five representative iron duricrusts and two clayey samples using a Bruker diffractometer D8 ADVANCE with a copper source (λ = 1.5489 Å) working under 40 kV and 40 mA. The exposure time for qualitative analysis was 2 h. The samples were pulverized in an agate mortar; the resulting powder was picked up on a piece of tape before being irradiated with Cu Kα radiation in the diffractometer. The resulting diffraction spectra were compared with a computerized database of common minerals, and automatic mineral-matching function was assisted by operator identification of phases consistent with the known compositions of the materials. Phase proportions were estimated by the peak-matching program without calibration to synthetic mixtures of known phase proportions. Also, sandstones were paned in order to determine the mineralogy of heavy minerals present in sand.
The chemical investigations were performed at the ALS Chemex Laboratories of Pretoria, South Africa. The chemical data were obtained from homogenized powders of twenty bulk samples of five representative iron duricrusts (conglomeratic, nodular, massive, fine in conglomerates and ferruginous sandstone) and six samples of clayey materials chemically analyzed for major elements by inductively coupled plasma atomic emission (ICP–AES) after dissolution by acid digestion with HF, HNO3, and HClO4. Trace elements were also analyzed by inductively coupled plasma mass spectrometry (ICP-MS). Elements were analyzed from powders, which were firstly digested in mixed acid (HF + HNO3 + HClO4) at ∼200 °C within pressure-tight Teflon cups, and then the dissolved solution was measured on a type PerkinElmer Elan 9000 instrument. Instrumental sensitivity and matrix effects are corrected using an indium internal standard and through standardization to matrix-matched multi-element solutions. Precision for trace elements is better than 5% (Burnham and Schweyer, 2004).
Biostratigraphical analyses were performed on the clayey samples from the Missole outcrop at the Belgium National Museum of Liège. The biostratigraphical study was based on comparison of the microsporomorphs from Missole with some data from Africa and South America (Atta-Peters and Salami, 2004; Jaramillo and Dilcher, 2001; Mbesse et al., 2012; Stead and Awad, 2005) and of “din-flagellum” with some data from Cameroon and from other areas in the world (Atta-Peters and Salami, 2004; Bankole et al., 2007; Jan du Chêne and Adideran, 1985; Mbesse et al., 2012; Oloto, 1992; Yepes, 2001). These microscope observations were done on thin layers obtained after chemical treatment of clayey powders with HCl (30%), HF (40%) and a second high-temperature HCl treatment, followed by oxidation of the organic matter by HNO3, sieved at 12 μm.
5 Results
5.1 Facies analysis
Outcrops from Missole I show three main types of sedimentary facies containing iron duricrusts and mixed up with small amount of silt, reaching 9 m of thickness (the main outcrop). From the bottom to the top, one can distinguish: sandstones with interstratified clayey silty stones, claystones, and conglomerates (Fig. 2). These sediments contain cobbles to fine-grained quartz, micas (mainly muscovite), which suggest their terrestrial source. The general color of sandstones is white, yellow or red, while claystones show a grey to dark grey color and conglomerates are yellow. Iron duricrusts are characterized by their red color, indicating oxidation and their texture depends on the type of sediments. Based on this observation, the studied sedimentary materials were grouped into fifteen (15) main facies including (Fig. 2): five iron duricrust facies (C1, C2, C3, C4, and CI), one conglomeratic facies (Gm), seven sandstones facies (S1, S2, S3, S4, S5, S6, and S7) and two claystones facies (Fm1 and Fm2). The facies survey provides insight into the depositional processes on the basis of the hydrodynamic regimes described by Miall (1978) and Postma (1990). Facies have been characterized, interpreted and classified from coarsest (C or G) to finest (F) sediments and a code (Table SM1, Supplementary data) modified after Miall (1978) and Postma (1990) has been assigned to each facies. The five up-mentioned iron duricrusts facies were differentiated based on their structure and texture, and could be considered as “palaeosoil” because they were formed under dried conditions. From the bottom to the top they include ferruginous sandstones (C1), massive conglomeratic duricrust (C2), nodular duricrust (C3), massive duricrust (C4), and laminated iron duricrust (CI). These facies and claystones facies are the main target of this study. Claystones were used for biostratigraphical study in order to reconstitute their palaeoenvironment and to determine their relative age.
5.2 Mineralogy
Our X-ray diffraction patterns indicate that iron duricrusts are essentially composed of goethite, quartz, hematite, kaolinite and smectite. Magnetite, anatase, halloysite, diaspore, chlorite, lepidocrosite, ferrihydrite, and dickite are accessory minerals (Fig. 3). On the other hand, claystones facies are composed of goethite, quartz, kaolinite, magnetite, dickite, smectite, and feldspars (Fig. 3).
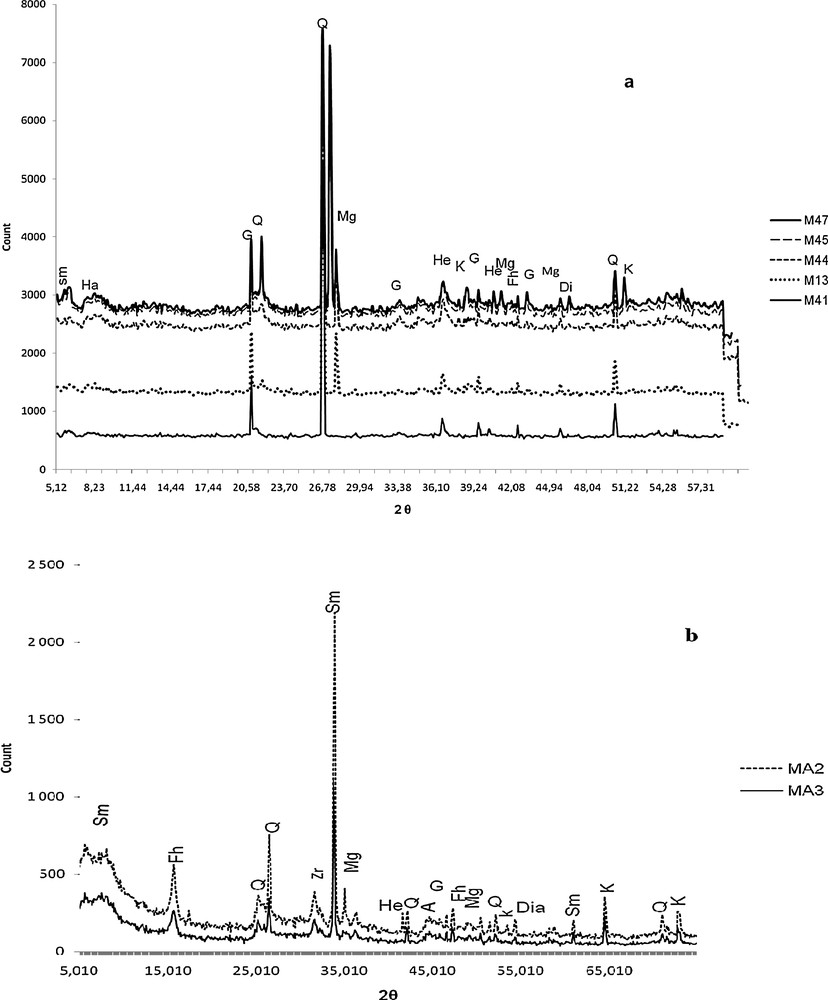
X-ray diffraction patterns of iron duricrust (a) and clayey (b) samples of Missole I. M41: conglomeratic duricrust; M13: nodular duricrust; M44: massive duricrust; M45: fine iron duricrust in conglomerates; M47: ferruginous sandstones; MA2: dark-grey heterolithic claystones/siltstones of facies Fm2; MA3: yellow-red massive claystones of facies Fm1; Q: quartz; K: kaolinite; G: goethite; He: hematite; Mag: magnetite; Hal: halloysite; Sm: smectite; Di: dickite; An: anatase; Fh: ferrihydrite; Dia: diaspore; F: Feldspar; Zr: zircon.
5.3 Geochemistry
5.3.1 Major elements geochemistry
The sampled clayey sediments and iron duricrusts are generally siliceous with small amount of alkaline earth and alkaline oxides (MgO, CaO, Na2O, K2O). On average, the clayey samples of Missole I are aluminous and relatively homogeneous, with small amount of iron (except samples MA4 with 22.9% of Fe2O3 and MA6 with 12.9% of Fe2O3) (Table SM2, Supplementary data). Differently, iron duricrusts are generally siliceous and ferruginous (Table SM3, Supplementary data). However, the ferruginous sandstones are more siliceous (66.2 to 75.7% of SiO2) and less ferruginous (< 19% of Fe2O3) than the other iron duricrusts, whereas the massive iron duricrusts are more ferruginous and less siliceous.
5.3.2 Trace elements geochemistry
Trace element concentrations of Missole I clayey materials and iron duricrusts are respectively reported in Tables SM4 and SM5, Supplementary data. These materials show the same behavior with trace elements in conglomeratic, weathered conglomeratic iron duricrusts, ferruginous sandstones and claystones. They are systematically depleted in lithophile elements (LILE) like Ba, Rb, Cs and Sr, high-field-strength elements (HFSE) like Nb, Th, Y, U and other trace elements like Ga and W (exception of some HFSE like Ta and Zr, Hf, which are enriched with these materials). However, they are enriched with transition trace elements (TTE) like Cr (exception of V in massive and nodular iron duricrusts).
Chondrite-normalized REE display chondrite-normalized patterns for the Missole I clayey materials and iron duricrusts (Fig. 4a–d) that show some fractionation of the REE group relative to chondritic meteorites. The sums of REE concentrations in iron duricrusts vary widely and the variation ranges between 45.68 to 137.15 ppm, which is lower than the average PAAS (185.3, Taylor and McLennan, 1985), whereas clayey materials have REE abundances higher than the average PAAS, between 192.81 and 790.07 ppm (Table SM5, Supplementary data). Clayey materials and iron duricrusts have high concentrations of LREE than MREE and HREE with high LREE/HREE ratios between 14.5 to 38.04, and 10.45 to 46.8, respectively. Also, these materials show similarly high (La/Yb)N ratios ranging between 5.08 to 15.25, and 3.99 to 14.17, respectively, and this is due to their high fractionation. However, all groups of iron duricrusts and clayey samples show similar chondrite-normalized patterns, parallel to sub-parallel (Fig. 4a–d). Moreover, all the samples show negative prominent Eu anomalies (Fig. 4a–d) with (Eu/Eu*)N ratios ranging between 0.53 and 0.58 for clayey materials, and 0.41 and 0.77 for iron duricrusts and no Ce anomaly, except two clayey samples (MA2 and MA5) and four iron duricrusts samples (M11, M41, M42, and M47), which have slightly or highly positive Ce anomaly (1.15 and 1.17, and 1.31, 1.31, 1.36, and 1.24 respectively).

Chondrite-normalized patterns of rare-earth elements (REE) of the Missole I conglomeratic and weathered conglomeratic duricrusts (a), nodular duricrust (b), massive duricrust and claystones (c), and fine iron duricrust in conglomerate and ferriband (d). After Taylor and McLennan (1985).
5.4 Palynomorph observations
The two clayey samples analyzed from Missole outcrop revealed that they are enriched with din-flagellum cysts and microsporomorphs. This outcrop contains well-preserved mio-spores, whereas most of the inventory made on din-flagellum cysts is more or less preserved (Plate 1). Moreover, there is a great diversity of pollen specimen whereas the din-flagellum cysts, despite their abundances are slightly diversified. Twenty specimens of pollen grains and fifteen din-flagellum cysts are highlighted (Plate 1).

Din-flagellum and microspores of the Missole I clayey materials (Douala sub-basin). 1. Cyathidites congoensis (39–40 μm). 2. Racemonocolpites facilis (35 × 34 μm). 3. Longapertites marginatus (43 × 26 μm). 4. Mauritiidites franciscoi var pachyexinatus (63 × 46 μm). 5. Mauritiidites lehmanii? (40 × 25 μm). 6, 7. Proxapertites operculatus (42 μm and 35 μm). 8. Momipites africanus? (29 × 25 μm). 9. Momipites eoafricanus? (30 × 27 μm). 10. Cricotriporites guianensis (24 × 27 μm). 11. Psilatricolpites sp. 1 (24 × 20 μm). 12. Longapertites vaneendenburgi (51 × 18 μm). 13–15. Retistephanoporites minutiporus? (48–32 μm). 16. Psilatricolpites sp. 2 (25 × 24 μm). 17. Psilatricolpites sp. 3 (25 μm). 18, 19. Psilatricolporites sp? (21 × 20 μm). 20. Retitricolpites clarensis (28 × 27 μm). 21. Psilatricolpites sp. 4 (31 × 29 μm). 22. Psilastephanocolporites scabratus (26 × 25 μm). 23, 24. Psilastephanocolporites sp. 1 (32 × 37μm and 35 × 33 μm). 25. Retitricolporites sp. (42 × 32 μm). 26. Striatopollis bellus (66 × 35 μm). 27. Cerodinium wardenense (59 × 58 μm). 28. Apectodinium homorphum (71 × 57 μm). 29. Apectodinium quinquelatum (77 × 80 μm). 30. Homotryblium tenuispinosum (79 × 72 μm). 31. Diphyes spinulum (73 × 69 μm). 32. Kallosphaeridium yorubaense (40 × 39 μm). 33. Eocladopyxis peniculata (80 × 76 μm). 34. Hystrichosphaeridium tubiferum? (44 × 39 μm). 35. Oligosphaeridium? (63 × 77 μm).
The composition of sporomorphs and din-flagellum cysts shows that palm trees (Proxapertites operculatu, P. cursus, Spinizonocolpites baculatus, S. echinatus) are the dominant pollen grains with the unknown affinity specimens like Retitricolpites clarensis, relatively Psilastephanocolporites; whereas the din-flagellum cysts are dominantly represented by gender Apectodinium (A. homomorphum, A. quinquelatum, A. hyperacanthum), Hafniasphaera septata, and relatively Kallosphaeridium.
6 Discussion
6.1 Biostratigraphy
Most of the found din-flagellum cysts characterize the period of Upper Paleocene–Lower Eocene although some of them have few precise stratigraphical extensions (Hafniasphaera septata, Spiniferites ramosus, Cordosphaeridium inodes, Cordosphaeridium cf. minimum, Cordosphaeridium fibrospinisum). However, assemblies of “din-flagellum” are constituted of specimens with stratigraphical features. Cerodinium wardenense and Eocladopyxis peniculata occur in Nigeria and Cameroon at the Upper Paleocene–Lower Eocene (Jan du Chêne and Adideran, 1985; Mbesse et al., 2012), whereas Homotryblium tenuispinosum is also known in Nigeria and Ivory Coast at the same period (Bankole et al., 2007; Digbehi et al., 2012). Moreover, Apectodinium hyperacanthum appears in Nigeria and Cameroon (Gulf of Guinea, Africa), and in the northern sea of Europe at the Upper Paleocene (Bujak and Mudge, 1994; Jan du Chêne and Adideran, 1985; Mbesse et al., 2012). The relative abundance of genus Apectodinium (Plate 1) in the world characterizes the Upper Paleocene and corresponds to the upper half part of the NP9 nanoplancton scale. Following this observation and with the absence of Lower Eocene marker such as Wetzeliella, an Upper Paleocene age and precisely Thanetian age could be suggested to the Missole I studied clayey materials.
On the order hand, all the observed terrestrial sporomorphs belong to the stratigraphical period of Cretaceous–Lower Eocene. However, the relative abundance of Retitricolpites clarensis and Proxapertites genus would characterize an Upper Paleocene age. Furthermore, Racemonocolpites facilis, Retitricolporites irregularis, Striatopollis bellus confirm the Thanetian feature of the analyzed sediments.
6.2 Geochemical and biostratigraphical pattern
Several studies showed that trace elements like V, Cu, Ni, Cr, Co, Th, U, Zn and V/Cr, Cu/Zn, Ni/Co, U/Th or Th/U ratios are used to depict conditions of the depositional environment (Akinyemi et al., 2013; Ramasamy et al., 2007). Somayajulu et al. (1994), and Madhavaraju and Ramasamy (1999) suggested that small contents of U in the sediments suggest oxidation conditions of the depositional environment. In the study area, U contents vary between 1.57 and 4.43 ppm in the iron duricrusts, and 3.39 and 5.16 ppm in the clayey materials. These contents suggest an oxidizing environment.
Furthermore, the V/Cr, U/Th or Th/U ratios are useful to characterize the depositional environment. In fact, for an anoxic environment, the U/Th ratios are higher than 1.25, and for an oxidizing environment the U/Th ratios are lower than 1.25 (Nath et al., 1997). Most iron duricrusts data and all the data obtained on clayey materials in Missole I show that U/Th ratios are lower than 1.25. The values of the studied materials suggest oxidizing conditions. Moreover, the V/Cr ratios of iron duricrusts and clayey materials are mostly lower than 2 in this study and suggest an oxidizing environment (Ramasamy et al., 2007).
On the order hand, following the biostratigraphical observations, the Apectodinium spread reveals the existence of a shallow marine environment with low salinity or a lagoon environment with dominant feature of saline waters (Bujak and Mudge, 1994; Pross and Brinkhuis, 2005). These types of environments evolve under a hot climate, 20 °C in average or more in anoxic conditions with an eutrophication of the water surface. In addition, the presences of Achomosphaera sp., Cordosphaeridium cf. inodes, and Hafniasphaera septata relatively show a coastal environment.
Moreover, the vegetation is essentially composed of palm trees taxon type. The genders Proxapertites and Spinizonocolpites (Nipa) are adapted to the mangrove biome (Germeraad et al., 1968). However, the less important presence of low-altitude humid dense evergreen forest elements such as the Ceasalpiniaceae family (Striatopollis bellus) with big trees is equally observed. These leguminous are associated with Euphorbiaceae, genders Amanoa (Retitricolporites irregularis) and Cotron (Crototricolpites densus).
The difference between the chemical data observations suggest oxidizing conditions while the biostratigraphic observations suggest anoxic conditions that are due to claystones deposits in simplistic environment such as a lagoon environment or a coastal marginal marine environment and are actually found in an oxidizing environment after their emersion.
6.3 Duricrusting phenomena
The iron duricrusts observed in the Douala sub-basin, notably at Missole I, are found in the transition of main sedimentary facies: sandstones, claystones and conglomerates and are essentially constituted of those materials. Their high Chemical Index of Alteration (CIA) suggests that they are made up of strongly weathered materials.
In fact, several studies were conducted on the formation of iron duricrusts. Nahon (1986) showed that iron duricrusts were formed in a contrasted season of humid tropical climate. Tardy and Nahon (1985) point out that duricrust phenomenon took place in sites rich in kaolinite where iron accumulates in centripetal manner. Leprun (1983) indicates that the alternations of hydration and drying have allowed reorganization and constant fixation of iron upwards, and also are the precursors of degradation. Moreover, some hypotheses were emitted on ferruginous phenomena in sedimentary environment. Arno and Alexandre (2005) suggested that this sedimentary ferruginous phenomenon resulted from diagenesis or direct precipitation of iron oxy-hydroxides. Pay (1983) supposed that ferruginous sandstones would originate from in situ precipitation, or pedogenesis or diagenesis processes. The iron of these duricrusts would derive from continental erosion (Khalid et al., 2011) and/or from solution transfers and precipitation in situ (Bocquier et al., 1984). Very recent studies (Arnold et al., 2015) highlight the usefulness of Fe fractionation isotopes in order to specify the conditions of remobilization and transfer of iron in soils.
The various iron duricrusts from the Douala sub-basin and particularly in N’kapa Formation at Missole I have diverse origins due to their features. The conglomeratic, nodular, massive duricrusts and fine laminated iron in conglomerates suggest a long period with no deposition due to the surrection of the continental margin (Mvondo, 2011) under contrasted seasonal climatic conditions. At this time, the exhumation of materials previously deposited has conducted to put soil in place (Nahon, 1986). These pedogenetic processes are characterized by the presence of iron oxides like hematite and magnetite in our study, as also reported by previous works in Bomkoul and Missole I and II (Ngon Ngon et al., 2012, 2014). They are also marked by depletion of alkaline elements, total or part of silica and a relative accumulation of iron and aluminum (Bocquier et al., 1984). Beauvais and Chardon (2013) showed that the lateritic mantle capped with ferricretes has had an important development in Africa under contrasted tropical climates during the Oligocene–Neogene.
Moreover, the Missole iron duricrusts have a post-Thanetian age according to the relative age of claystones (Thanetian) and were formed in oxidizing environment after the deposition of sedimentary materials and their late exhumation under an arid climate. The wetness following aridity promoted the moisturizing phenomena of iron oxides to iron oxy-hydroxides with the breaking up of duricrusts (Beauvais, 2009). This moisturizing phenomenon is marked by more or less abundant goethite and ferrihydrite in our study area.
However, ferruginous sandstones suppose other processes of formation. Zalat et al. (2012) suggest that the compaction and the consolidation of sediments are due to the pressure of charge and water desaturation. Pettijohn et al. (1973) defined the compaction like the itinerary from which the grains’ organization changes with the reduction of porosity. In this way, the Missole I ferruginous sandstones suppose the action of sediment charges, which should be due to the compaction origin and the diminution of pores in the water circulation zone. This compaction should be the result of water desaturation and particle reorganization. The bended morphology of ferribands should be due to the depth of sandstone bed and the weakness of sand grains like the ferribands from the Gulf of Suez in Egypt (Zalat et al., 2012).
7 Conclusion
The Missole iron duricrusts from the Douala sub-basin in Cameroon are generally siliceous and ferruginous, with small amounts of alumina (less than 7% of Al2O3), alkaline earth and alkaline oxides (MgO, CaO, Na2O, K2O) and high LOI content. In general, iron duricrusts and clayey samples are depleted in most major elements and display a high TiO2 content. In addition, they are depleted in LILE such as Ba, Rb, Cs and Sr, HFSE such as Nb, Th, Y, U and other trace elements like Ga and W, and are enriched with HFSE like Ta and Zr, Y, Hf and with TTE like Cr, with the exception of V. With their high CIA values, the Missole I iron duricrusts and clayey materials are strongly weathered. These materials have undergone a primary chemical weathering in the catchments region under humid tropical climate and secondary chemical weathering in the coastal plain region after their deposition under similar climatic conditions.
REE show similar patterns in iron duricrusts and clayey sediments with high LREE concentration and low HREE. Chondrite-normalized REE patterns show negative Eu anomalies and high fractionation between LREE and HREE. Moreover, prominent Eu anomalies with high (La/Yb)N are observed in iron duricrusts and clay sediments essentially derived from silicic or felsic parent rocks when fractionated chondrite-normalized REE patterns also indicate felsic or silicic parent rocks.
Furthermore, the V/Cr, U/Th or Th/U ratios of the studied materials suggest oxidizing conditions, whereas the biostratigraphical observations enhance the Apectodinium spreading that documents the existence of a coastal marginal marine environment with low salinity or a lagoon environment with dominant feature of saline waters. Moreover, the Missole I iron duricrusts have a post-Thanetian age according to the relative age of claystones suggested by palynomorphs analysis. In fact, the sediments analyzed during this work are not older than the Thanetian.
Acknowledgements
The authors are grateful to the staff of the “Institut de recherches pour le développement” (IRD), France, for mineralogical analysis and the staff of ALS Chemex Analytical Laboratories, South Africa for geochemical analyses.