1 Introduction and methodology
Nuclear energy produces long-lived radioactive waste. Their long-term disposal provides a major challenge for materials science. Waste material has to be stored safely for at least 105 yrs, in order to decrease significantly the radioactive dose. Many countries that use nuclear energy resort to the vitrification of the long-lived high-level waste in order to stabilize radionuclides. Due to long timescales, 105 to 106 yrs, for a significant reduction of waste radioactivity, the option for the management of these wastes is the disposal in a deep geological repository (Fig. 1). In France, the geological formation studied is the Callovian-Oxfordian claystone formation located in the northeastern sector of the Paris basin (Trouiller, 2006). The repository is designed as a redundant multi-barrier system, in order to protect the biosphere from radionuclide dispersion: the waste packages are placed in steel containers and confined by engineered barriers and clay layers (Andra, 2005).

Temporal evolution of the radioactivity of a nuclear waste after its production. A significant drop occurs after 300 yrs. This explains that nuclear wastes will be stored for this period before their disposal in a deep geological repository. The second drop occurs after 4000 yrs and corresponds to the assessed durability of steel containers. The dashed grey line represents the radioactivity of the quantity of uranium ore necessary to produce the fuel retreated in a glass package. The time after which the radioactivity is under this level is around 105 yrs.
Évolution temporelle de la radioactivité d’un déchet nucléaire après sa production. Une diminution significative apparaît après 300 ans, durée qui correspond à la période de réversibilité du stockage. La durabilité du container en acier est estimée aux alentours de 4000 ans. La ligne grise en pointillé représente la radioactivité du minerai d’uranium nécessaire pour produire le combustible retraité dans le déchet vitrifié. Le temps nécessaire pour abaisser la radioactivité en dessous de ce niveau est de l’ordre de 105 ans.
The glass being the first barrier, it is necessary to assess its alteration rates in order to quantify the release of radionuclides. This requires the prediction of the long-term behavior of glass packages under geological repository conditions (Fig. 1). Predictive models of glass alteration are based on the understanding of alteration mechanisms and are parameterized with experimental data (see for instance Frugier et al., 2008; Gin et al., 2008; Grambow, 1985; Grambow and Müller, 2001). Their long-term predictive capacity to assess the nuclear glass lifetime may be validated, by using natural or archaeological analogues (Ewing, 1979, 2001; Petit, 1992). The aim is not only to better constrain the phenomenology of glass alteration and to understand its mechanisms, but also to demonstrate that experimental short-term data are able to predict long-term alteration in complex environments (Fig. 2).
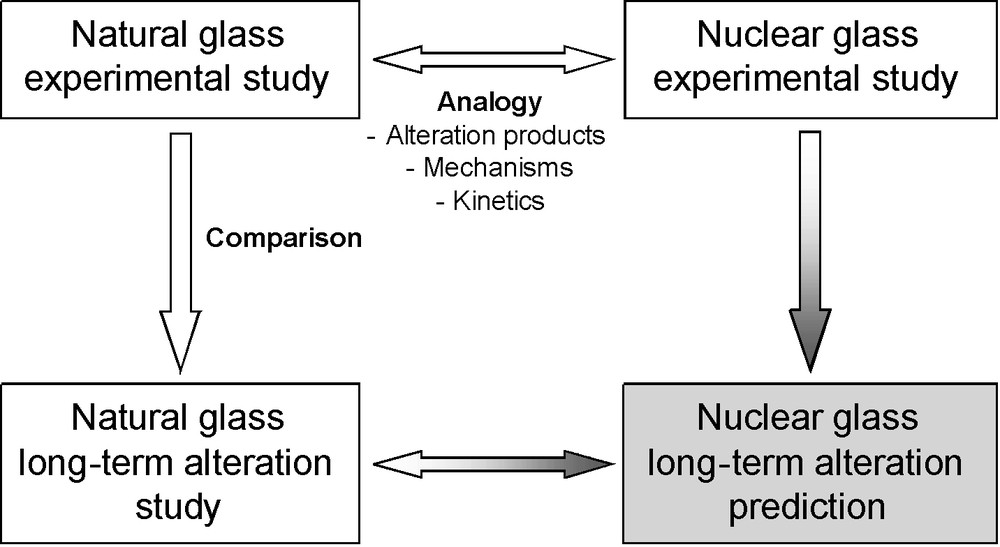
Principle of the reasoning by analogy applied to the natural or archaeological glass study. If the analogy between natural glass and nuclear glass is demonstrated, then the long-term durability observed for natural glass can be inferred for nuclear glass.
Principe du raisonnement par analogie. Si l’analogie entre les verres naturels ou archéologiques et les verres nucléaires est établie, alors la durabilité à long terme des verres naturels peut être appliquée aux verres nucléaires.
The word “analogy” is coming from the ancient Greek and originally meant proportionality in the mathematical sense (A is to B as C is to D). Reasoning by analogy consists in concluding from the relationship that links two terms (A and B) a property of the relationship that links two other terms (C and D), in a similar relation. If a model based on short-term laboratory data accounts for the phenomenology and kinetics of natural or archaeological glasses altered for long timescales, it improves the confidence in the long-term predictions of the models developed for nuclear glasses.
“Analogous” means similar and not identical. In the case of the French nuclear waste glass, archeological or basaltic glasses are good potential analogues because the alteration phenomenology and the mechanisms involved are generally common to all silicate glasses. In contact with water, silicate glasses suffer the same reactions: i) water diffusion in the glass and ion exchange (between glass modifier cations and hydrogen species) (Chave et al., 2007; Hamilton et al., 2000; McGrail et al., 2001; White and Claasen, 1980), ii) hydrolysis of the glassy network (Si-O-Si, Si-O-Al…) and condensation reactions (Bunker, 1994; Oelkers, 2001; Pelegrin et al., 2010; Valle et al., 2010;) with the formation of a “gel” layer, iii) precipitation of secondary phases (Frugier et al., 2006; Stroncik and Schmincke, 2001; Verney-Carron et al., 2010). The evolution of glass alteration kinetics is also similar and depends on the rate limiting reaction. Three rate regimes can be distinguished (Frugier et al., 2008): i) a maximal “initial rate” that corresponds to the hydrolysis of the glassy network, ii) “the rate drop” due to saturation effects in solution and to the formation of a protective gel layer by hydrolysis/condensation reactions (Cailleteau et al., 2008), iii) the “residual rate” that is very low but not nil due to the diffusion of mobile elements through the alteration layers and the secondary phase precipitation. The objective for understanding the long-term behavior of analogous glasses does not rely on an alteration environment similar to that expected in a geological repository, but rather on the interactions between the glass and its environment (steel, clay minerals, groundwater.), glass composition influencing mainly the alteration kinetics (Verney-Carron et al., 2010a and references therein).
In this article, we present several studies of natural or archaeological analogues to show how understanding the nuclear glass alteration phenomenology has led to the choice of specific analogues and has improved the confidence in long-term glass alteration modeling.
2 The understanding of alteration mechanisms
2.1 Alteration of stained glasses: influence of glass composition and weathering conditions
In the lack of natural borosilicate glasses, well-dated medieval stained glasses provide a good proxy for understanding the processes and kinetics of glass alteration (Macquet and Thomassin, 1992; Sterpenich, 1998; Sterpenich and Libourel, 2001, 2006). Manufactured from natural materials (e.g. washed siliceous sand, limestone, plant ashes, etc.) and colored by transition elements, stained glasses offer a large variety of compositions (i.e., from Na- and Si-rich to K-rich and Si-poor glasses). It is possible to assess how chemical composition controls glass durability and the behavior of minor/trace elements, under natural alteration conditions and over 102–103 yrs. Depending on their exposure (Fig. 3), stained glasses can also provide a unique way to characterize the effects of weathering conditions on glass alteration (Libourel et al., 1994): while samples from archaeological sites afford information on alteration by groundwater, stained glass windows are subject to meteoric weathering or to alteration driven by moisture, for outside or inside surfaces, respectively.
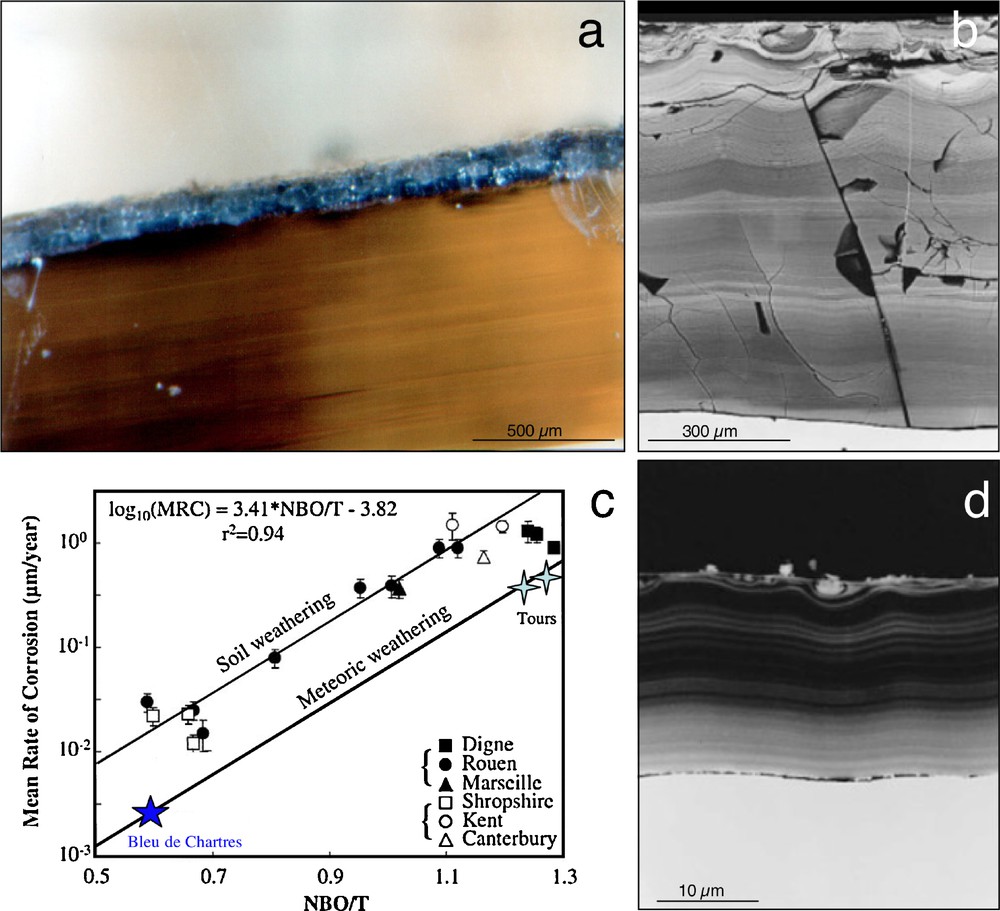
Alteration of stained glass windows. a) Outside surface of a yellow stained glass from St-Gatien cathedral (Tours, France, 14–15th century), showing a continuous thickness of alteration of 100–150 μm. b) Alteration layer of a green K-rich stained glass excavated from the archeological site of Notre-Dame-du-Bourg church (Digne, France, 13th century). Notice the laminated structure as well as the important thickness of the alteration layer (around 1 mm). c) Mean corrosion rates as a function of the bulk polymerization of the glass. See text for explanations. d) Alteration layer of Na-rich stained glass excavated from the archeological site of the Bishop's palace in Rouen (France, 9th century). The thickness of the alteration layer is an order of magnitude lower than that measured on K-rich glasses (b).
Altération des verres de vitraux médiévaux. a) Surface extérieure d’un verre jaune potassique de la Cathétdrale St-Gatien (Tours, France, xiv–xve siècle), montrant une altération continue d’une épaisseur de 100–150 μm. b) Couche d’altération d’un verre vert potassique exhumé du site archéologique de l’église de Notre-Dame du Bourg (Digne, France, xiiie siècle). Noter la lamination et l’épaisseur importante (de l’ordre du 1 mm) de cette couche d’altération. c) Taux moyen d’altération en fonction de la polymérisation globale du verre. (voir explications dans le texte). d) Couche d’altération d’un verre sodique exhumé du site archéologique du Palais episcopal de Rouen (France, ixe siècle). L’épaisseur de la couche d’altération est d’un ordre de grandeur plus faible que celle mesurée sur les verres potassiques (b).
Whatever the kind of alteration, stained glasses present traces of corrosion on their surface, including a weathered glass layer (gel) and precipitates. Optical or scanning electron microprobe observations reveal that the structure and thickness of the altered zone depend on the type of weathering (Sterpenich, 1998; Sterpenich and Libourel, 2001). For a fixed glass composition, alteration driven by moisture produces only scattered dissolution pits, whereas atmospheric weathering produces a continuous altered and micro-fractured layer up to 200 μm thick (Fig. 3a). Buried in soils, stained glasses show a more pervasive weathering with alteration of up to 1500–2000 μm (Fig. 3b) and a characteristic laminated structure. Dissolution rates for given weathering conditions depend on glass composition. For instance, under burial conditions in the archaeological site of Rouen (Normandy, France, 9th century), the mean thickness of the altered zone for soda-rich glasses is around 30 μm, while for K-rich samples it may exceed 1000 μm (Fig. 3b and d). Similarly, potassic glass windows are always darkened by alteration layers while Na-rich glasses on the same windows preserve their color and have alteration zones less than 2 μm thick, as in the blue stained glass of the Chartres cathedral (France, 13th century).
Due to the good mechanical cohesion between weathered and unweathered glass, mean corrosion rates can be estimated by dividing the mean thickness of the alteration crust by the duration of weathering. Mean corrosion rates for stained glasses in natural conditions over the last 1100 yrs, are ranged approximately 0.005–1.5 μm.yr−1 and are dependent on glass composition and on alteration conditions. Mean corrosion rates being correlated with the bulk degree of polymerization of the glass, the NBO/T parameter (NBO/T denotes bulk melt non-bridging oxygen per tetrahedral cation, Mysen, 1988) allows to evaluate the specific role of glass composition and alteration conditions (Fig. 3c). For K-rich depolymerized (silica-poor) glasses, mean corrosion rates are almost two orders of magnitude greater than for polymerized Na-rich glasses. However, for the same composition, glass dissolution rates on the outside of windows are only 6–7 times slower than for glasses buried in soils. This suggests that variations in glass composition lead to variations in glass dissolution rates an order of magnitude more pronounced than those caused by different weathering conditions. Mean elemental dissolution rates can be also estimated for weathering durations over more than 103 yrs (Sterpenich and Libourel, 2001). Alkalis (K, Na, Rb and Cs) and alkaline-earth elements (Mg, Ca, Sr and Ba) are the most leachable elements, with leaching rates ranging from 10−2 to 10−3 g.m−2.day−1, while network forming elements (Al, Fe, Zr, Ti) are less depleted ≈10−4 g.m−2.day−1, Si being intermediate. Lanthanides and actinides, with the noticeable exception of U and Eu, are almost insensitive to alteration with the lowest dissolution rates at around 10−5 g.m−2.day−1.
The alteration of medieval stained glasses is thus strongly dependent on glass composition and on the weathering conditions with highly variable elemental dissolution rates. While alteration zones may play the role of a barrier limiting the release of several elements, stained glass studies reveal that a special care has to be taken in nuclear waste management strategy for the glass formulation and the weathering condition and their modifications in the repository during very long timescales (> 105 years).
2.2 Archaeological glass blocks and the impact of the fracturing
Nuclear glass packages are fractured due to a rapid cooling of the melt in the stainless steel canister (Fig. 4; Laude et al., 1982; Minet and Godon, 2003). Due to the release of strain upon glass cooling, fractures increase the reactive surface area and influence the alteration kinetics. Because of differences in the glass surface/water volume ratio or in the transport processes between the external border and the inside part of the cracks (Fig. 4c and d), surfaces can evolve with different alteration kinetics. In the following, it is shown how fractured archeological glasses can be used to assess the long-term alteration kinetics in the cracks and address the impact of fractures on the glass alteration.

Impact of fracturing on glass alteration. Photography of an Embiez glass block (a) and a cross-section of the R7T7 nuclear waste glass block (b). Notice the importance of the fracturing in both cases. SEM microphotograph (Back-scattered electrons) of the crack network in the Embiez glass block in contact with seawater (c) and in the internal zone (d).
Effet de la fracturation sur l’altération des verres. Photographie d’un bloc de verre des Embiez (a) et coupe d’un bloc du verre R7T7 de déchet nucléaire (b). Noter l’importance de la fracturation dans les deux cas. Microphotographie au microscope électronique à balayage (électrons rétrodiffusés) du réseau de fractures du bloc de verre des Embiez dans la partie externe en contact avec l’eau de mer (c) et dans la zone interne (d).
Archaeological glass blocks (0.5–10 kg) were recovered from a shipwreck in the Mediterranean Sea near the island of Embiez (Var, France; Fig. 4a). They have been altered for 1800 yrs in seawater at a depth of 56 m and a fixed temperature of 15 °C (Fontaine and Foy, 2007; Foy et al., 2005). The glass was produced in the eastern Mediterranean region. The materials were melted in a large furnace and cooled in air, which caused the fracturing. Glass slabs were cut into blocks to be shipped to Europe, where they would have been manufactured (Fontaine and Foy, 2007; Foy et al., 2005). The composition is typically that of a Roman soda-lime glass (∼ 70 wt% SiO2, 20 wt% Na2O, 5 wt% CaO, and 2 wt% Al2O3).
The altered glass (Verney-Carron et al., 2008) reveals, in addition to a small pristine core, an external zone, in contact with seawater, where the cracks have a large alteration thickness of 400 − 500 μm (Fig. 4c), and an internal zone, where the cracks become denser but are less altered, 5–30 μm thick (Fig. 4d). All cracks are filled with alteration products that were characterized by electron microprobe and μ-X-ray diffraction. The cracks in the external zone contain Mg- and Fe-rich smectites, whereas internal cracks have a large (relative to the total thickness) hydrated glass layer at the interface with the pristine glass and only a few μm thick of Mg-rich smectites in the center of the crack (Fig. 4). This shows that the kinetics of the alteration mechanisms (ion exchange and dissolution) differs according to crack location. These differences are well explained by a coupling between alteration kinetics and element transport in solution (Verney-Carron et al., 2010a). The continuous renewal of the seawater in contact with external cracks maintained an “initial rate” for 1800 yrs and leads to thick neoformed smectite layers. In the internal cracks, the transport is assumed to be diffusive and the slow renewal of the solution favored saturation effects and low alteration rates. Moreover, crack sealing by alteration products slowed down the diffusive transport (Verney-Carron et al., 2008, 2010a).
In the present French models of R7T7 alteration rates, used in safety calculations, the increase of the reactive surface area is taken into account via two fracturing ratios (τ): τ0 for the external cracks and τr for the internal cracks that are assumed to be altered at the “residual rate” (Gin et al., 2005). The study on Embiez archaeological validates that this hypothesis remains conservative (Verney-Carron et al., 2010b).
2.3 Archeological iron making sites and primitive meteorites: insights on the alteration at the glass–metal–clay interfaces
To gain insight in the long-term behavior of nuclear waste packages at the glass–metal–clay interfaces in their geological repository conditions, we show in the following how slags from ancient iron making sites or primitive meteorites can be used as valuable proxies (Fig. 5). The stainless steel canister of nuclear waste is expected to corrode in anoxic conditions. Both analogues provide unique information about long-term corrosion under anoxic conditions of metal iron, which is rare on Earth.
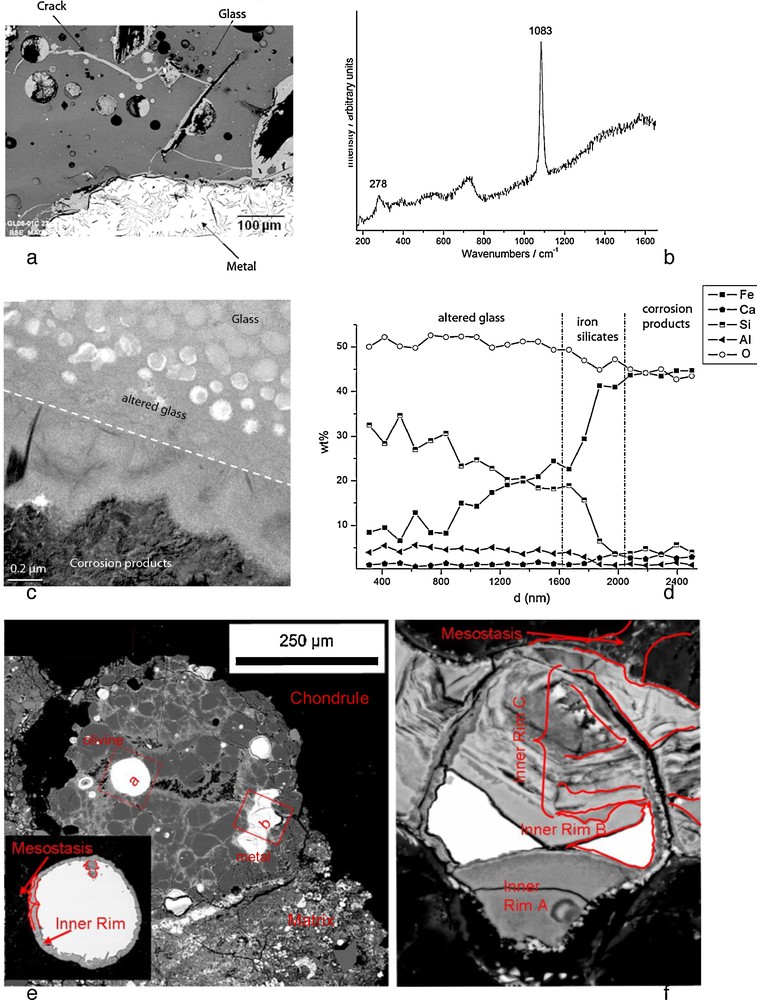
Slags from ancient iron making sites (a to d) and primitive meteorites (e and f) as analogues of the alteration of glass-metal interfaces in anoxic repository conditions. (a) SEM. Ironmaking slag sample containing metal, glass and the crack network filled with corrosion products, (b) μRaman spectra of siderite (FeCO3) from the corrosion products, (c) TEM photograph of the glass/corrosion product interface, (d) EDS-STEM chemical distribution (Kα lines) perpendicularly to the interface. e) Back scattered SEM image of magnesian porphyritic chondrule of the Al Rais CR2 chondrite. Olivines and metals grains are associated with a glassy mesostasis. The insert shows details of the metal grains labeled (a). Notice the continuous rim of corrosion products surrounding the Fe-Ni metal grain. f) Enlargement of the altered metal grain labeled (b). There are three blebs of metal left, surrounded by layers of alteration phases (inner rim).
Scories d’anciens sites de fabrication du fer (a à d) et météorites primitives (e et f) utilisées comme analogues de l’interface verre-métal dans des conditions anoxiques de stockage. a) Scories contenant du métal et du verre, fracturées par un réseau de microfractures envahies de produits de corrosion, b) Spectre μRaman de sidérite provenant des produits de corrosion, c) Image en microscopie électronique en transmission de l’interface verre/produits de corrosion, d) Profil de composition chimique (STEM-EDS) à l’interface. e) Image en électrons rétrodiffusés au microscope électronique à balayage d’un chondre porphyrique de la chondrite Al Rais CR2. Les grains d’olivine et de métal sont dispersés dans la mésostase vitreuse. L’encadré montre le détail du grain de métal (a). Noter la couche continue de produits de corrosion entourant la bille de Fe-Ni. f) Agrandissement du grain de métal (b). Noter l’existence de 3 grains de métal reliques, entourés par des couches d’altération.
Ancient slags: The question of the interaction between glass and iron is particularly important because nuclear glasses are poured in a stainless steel canister and the resulting package is expected to be surrounded by a thick carbon steel overpack (Andra, 2005). Some laboratory experiments under anoxic conditions have shown that the presence of iron and, more generally, iron corrosion products sustain glass alteration at a rate higher than the glass would undergo in the presence of site water only. This enhancement of glass alteration is due to the strong affinity of iron rich phases with respect to silicon, the main component of the glass (De Combarieu et al., 2011; Jollivet et al., 2000). It has been asserted that the formation of Fe-Si containing phase delay the formation of the protective gel layer responsible for the drop of alteration rate observed in glasses altered by water under static or low flow rate conditions (De Combarieu et al., 2011). That is why understanding and modeling interactions between glass, iron and clay is a key preliminary step in order to predict long-term behavior of nuclear glass packages. Consequently, studies of natural or archaeological analogues give precious observational insight for this issue. For example, the site of Glinet dated from the 16th century (Neff et al., 2010), has been taken as a reference site for studying glass/iron interactions. Indeed, the by-product slags produced by the blast furnace present partly glassy matrix and contain metallic particles of micrometric to millimetric sizes. The blocks present numerous cracks that were immediately saturated with water as they were buried at depths continuously under the water level. It has been verified by on-field measurements and laboratory tests that at the sample location, water was carbonated and anoxic. Moreover, the crack network connects the metallic zones of the slags to the external solution of the soil and is entirely filled by corrosion products of iron such as those found in desaerated media (Fig. 5a). Microstructural characterization of the network embedded with the corrosion products by μRaman spectroscopy, reveal the presence of siderite (FeCO3 – Fig. 5b). This siderite contains several % of calcium partially substituting the Fe atoms. This substitution was also observed on iron artefacts corroded in the same medium and could come from the groundwater but also, in the present case, from the glass matrix, that contain this element. Glasses identified in Glinet are calcium silicate glass enriched in iron and aluminum (MgO: 0.7–2.2 wt%, Al2O3: 5.1–8.8 wt%, SiO2: 61.9–76.5 wt%, K2O: 1.8–2.3 wt%, CaO: 15.6–25.1 wt%, MnO: 0.2–2.9 wt%, FeO: 1.2–9.1 wt%). This range of compositions for the ternary CaO-A2O3-SiO2 defines an area known for its immiscibility. SEM-FEG observations confirm a non-homogeneous nanostructure characterized by the presence of spheroids of several hundreds of nanometers distributed in a glass matrix. This specific structure of the glass implies different alteration kinetics for each of the phases observed by TEM (Fig. 5c). The spheroids, mainly constituted of SiO2, are less altered than the embedding matrix containing higher concentration the other elements.
At the interface between glass and iron corrosion products, different layers have been observed, linked to the variation of composition (Fig. 5c and d). EDS analyses coupled to STEM indicate that the altered glass is depleted in Ca but not in Al. Interestingly, an external festooned layer between altered glass and siderite, made of amorphous iron silicates is observed (Fig. 5c). The study of this layer can give insight into the influence of iron on the glass corrosion. Moreover, iron coming from the metal corrosion products has penetrated into the altered layers of the glass. Laboratory experiments simulating a crack have been conducted for 120 days at 50 °C on synthetised glass samples with the structure and the chemical composition of the slag glass matrix. The chemical profiles of the glass alteration layers are very comparable to the archaeological ones and are currently under study. The results are expected to help discriminating between the controlling parameters of glass alteration: transport vs chemical equilibria.
Meteorites: CR chondrites collected immediately after their fall, are primitive meteorites that have experienced aqueous alteration of metal and glass in their parent bodies. Constituted of mixtures of ferro-magnesian minerals and alumino-silicate glasses in chondrules (Fig. 5e), iron-nickel metal and sulfides blebs seated in phyllosilicate-rich matrices, CR chondrites show a range of low-temperature aqueous alteration stages, from pristine, unaltered (type 3) to completely hydrated (type 1) material (Weisberg et al., 1993). They provide an analogue for alteration and corrosion processes at the glass–metal–clay interfaces over a longer time frame than is possible in laboratory. In addition, they allow the investigation of all steps in the expected corrosion of nuclear waste, given that the physico-chemical environment of the parent body during the alteration (e.g., Zolensky et al., 1993), i.e. temperature (50–150 °C), water/rock ratio (0.4 to 1.1), low oxygen fugacity and mineralogy, is similar to that expected in a disposal facility (Andra, 2005).
Fig. 5e depicts the corrosion products after partial alteration at the metal/glass interface in a chondrule from the Al Rais CR2 chondrite. This chondrule contains several metal grains at different alteration stages. SEM images show that Fe-Ni metal grains are surrounded by several alteration layers, with intrusions of neoformed phases into metal grains (Fig. 5e and 5f). These corrosion products have a complex mineralogy, with magnetite-rich layers alternating with layers enriched in sulfides (Fig. 5f). A genetic sequence for metal/glassy mesostasis, confirmed by elemental mapping, would be metal/magnetite ± sulfide/phosphate? ± carbonate? ± sulphate?/(altered) mesostasis. The alteration layers shown in Fig. 5e and f are enriched relative to the metal in S, Si, Mg, Ca and Mn. They also have significant contents in Ni, Cr, Co, P, which are enriched in some parts compared to the metal. In the case of metal/matrix interfaces, the sequence of corrosion products is: metal/magnetite ± sulfide/magnetite/phosphate + matrix. The alteration rims become more complex with increasing alteration, with steel components moving into the surrounding environment.
In addition to document corrosion processes at metal/glass/clay interfaces, such extraterrestrial analogues provide information on the elemental mobility during alteration in anoxic environments, even if the time-frame of parent body alteration is not well constrained (104–106 yrs or more). Chemical distribution maps show that most of the “dissolved” elements, including iron, does not go very far. Even for the most altered metal grains, the phases precipitate at distances of several 100 μm. This happens in the form of sulfides, phosphates, sometimes carbonates, and Fe-oxides, which tend to form characteristic rings in the near vicinity of the corroded grains. At the metal/glass interface, element migration is even smaller even less, in the order of tens of μm.
3 The prediction of glass alteration long-term kinetics
An important objective of the studies on natural and archaeological analogues is to validate the long-term capacity of predictive alteration models (Fig. 6). These models are based on experimental data to determine the kinetics parameters. The extrapolation to longer timescales of the models helps predict the long-term behavior of nuclear glasses. Long-term simulations can be compared with the characterization (phenomenology, apparent kinetics) of natural samples altered for a long time. If the results are in agreement, this validates the predictive capacity of the natural analogue and improves the confidence in the models predicting the evolution of nuclear glasses (Ewing, 1979, 2001).

Principles of the long-term predictive capacity of the natural (or archaeological) glass alteration modeling.
Principes de la capacité predictive de la modèlisation de l’altération des verres naturels (ou archéologiques).
3.1 The Embiez archaeological glass blocks
This methodology was applied to the Embiez glass blocks, altered for 1800 yrs in seawater at a constant temperature of 15 °C (Verney-Carron et al., 2008, 2010a). The stability of these alteration conditions allows long-term modeling. Verney-Carron et al. (2010a, b) demonstrated that a model parameterized in the laboratory is able to simulate the alteration of fractured historical glass blocks. Moreover, the coupling of alteration chemistry and transport in solution explains that external cracks in direct contact with renewed seawater were altered at a high dissolution rate (400 − 500 μm), whereas internal cracks are less altered (by 1 or 2 orders of magnitude) because the low renewal of interstitial water and the sealing of the cracks by alteration products favored low alteration kinetics.
3.2 The basaltic glass
A key issue to make a reliable and robust prediction of the long-term behavior of nuclear glasses is to determine realistic values of residual rate. Basaltic glasses, with a silica content close to nuclear glasses, altered in different environments (seawater, groundwater, meteoric water) can help in this direction, with the advantage of long timescales but large uncertainties on the alteration history. This ‘residual rate’ kinetic regime is expected to be the longest one in geological disposal conditions and explains most of glass alteration (Techer et al., 2001). The mechanisms involved at this stage of reaction progress are water diffusion through the passivating gel layer and precipitation of secondary crystalline phases that consume elements from the gel (Frugier et al., 2008). It has been shown that the residual rate depends on glass and water composition, the latter being influenced by chemical reactions in the near-field. Even if residual rates are accurately measured in laboratory, natural analogues may help to determine how this rate evolves as a function of water composition, renewal rate and time. Studying basalt alteration therefore provides a new challenge for geochemists.
4 Conclusion
This paper presents an overview of the major advances offered by natural and archeological analogues in the validation of predictive models of the nuclear glass alteration in a deep geological repository. Based on terrestrial or extraterrestrial analogues, it summarizes a rational methodology to tackle the difficulty of predicting the long-term behavior of waste materials, with the expectation of reconstructing their alteration history in the past and/or forecasting their evolution in the future. This approach represents a significant advance toward assessing the uncertainties on nuclear glass alteration rates in a geological repository, giving sound arguments to reach a decision for the disposal of these materials.
Acknowledgments
G.L and the authors are much indebted to Georges Calas, Gordon Brown, and the scientific committee of the Académie des Sciences and Institut de France for their invitation at the Colloque de l’Académie des Sciences (14-15 september, 2009) and to participate to this C.R. Geoscience issue on the Environmental Mineralogy. We are grateful to Christelle Martin, Nicolas Michau, Didier Crusset and Stephan Schumacher from Andra (French National radioactive waste management Agency) for many useful discussions and suggestions during the course of this research. Part of this work was financially supported by the GL-VFA of Andra contract n° 30010180. This is CRPG contribution n° 2097.