1 Introduction
Ventifacts are large clasts (pebble- to boulder-sized) shaped by corrasion (sensu Walther, 1900): abrasion by wind-carried particles. As introduced by Evans (1911), this term was restricted to forms showing one or more facet(s) cut by sandblasting (Windkanter in the German literature), but it was extended by Bryan (1931) to include all wind-worn elements, whether grooved, carved, striated or truly faceted. Most geomorphologists also extend this term to bedrock surfaces (Greeley and Iversen, 1985; Knight, 2008), but, for reasons discussed below (Section 7), this will not be the case in this paper.
Wind-worn stones are climate-sensitive sedimentary features that provide evidence for long periods without any vegetation in terrestrial environments (Glennie, 1970; Laity and Bridges, 2009; Sharp, 1949; Várkonyi and Laity, 2012; Walther, 1900). It is quite significant that, in Permian and Triassic red-beds, ventifacts (in situ or slightly reworked) and palaeosols of semi-arid types are mutually exclusive nearly everywhere (Bourquin et al., 2011). Therefore, they can be used with more confidence than aeolian dunes deposits to characterize desert conditions, under hot or cold palaeoclimates. In fact, aeolian dunes can occur under semi-arid and even humid climates, and from near the Equator to the Arctic Circle. It is also noteworthy that ventifacts that are reworked, but still recognizable, are not very far from their place of origin, as most of their characteristics disappear relatively quickly during transportation by water (Cailleux, 1942).
Presently, deserts cover about one-fifth of the Earth's land surface: 50% are stony deserts (regs), but only about 20% are covered by sand (Laity, 2008; Mountney, 2006). This justifies the large number of studies on Quaternary ventifacts, their origin and signification, that have been carried out since the mid-19th century (Knight, 2008): see the bibliography compiled by Bryan (1931) and by Gill et al. (2011). On the other hand, it is somewhat surprising that, although there is already quite extensive data on ventifacts from Mars (Bridges et al., 2004; Greeley and Iversen, 1985; Laity and Bridges, 2009), so little work has been devoted to ventifacts provided by the geological record, which have often been mentioned, but rarely described.
2 Context of this study
This paper draws on studies that were initiated about 40 years ago (Durand, 1972) by the discovery, in North-East France, southeast of the Vosges Massif, of an outcrop showing an almost undisturbed part of an extensive alluvial palaeoreg overlying the migmatitic Variscan basement (Fontenoy-le-Château: 47° 58′ 36.7″ N – 6° 12′ 36.8″ E). Alluvial palaeoreg means that clasts exposed are not residual (in situ) material but of alluvial (stream transported) origin (Laity, 2008, p. 162). That reg is one of the witnesses of the subtropical desert conditions that prevailed during a part of the Lower Triassic in most regions of Europe (Bourquin et al., 2007).
Close examination of each coarse clast available (from pebbles to small cobbles) showed that hardly more than 20% of them had kept their original (fluvial) rounded shape. The others bore features unknown on typical water-worn gravel of same lithology (quartzite and quartz), and therefore attributed to wind actions. It should be noted that, unlike most palaeo-periglacial Pleistocene ventifacts, the studied specimens were not found at the ground surface, but were extracted from Triassic sandstones; thus any recent change in their morphology, of natural or human origin, can be ruled out. Subsequently, their study led to the identification of many new sites located mainly in the Lower Triassic (Bourquin et al., 2007, fig. 1), but also in the Permian, Upper Triassic, and Pleistocene, from various countries in Europe and North Africa.
The experience thus acquired suggests that many clasts presented in the recent literature as ventifacts (Rodríguez-López et al., 2010; Soria et al., 2011) have nothing in common with true wind-worn stones, and conversely, that typical ventifacts often go unnoticed. These misinterpretations can be explained by the use of only a few oversimplified criteria, such as ‘polish’ and ‘dreikanter shape’, as well as by the scatter and relative scarcity of illustrations to which refer.
Accordingly, the aim of this synoptic presentation is to contribute towards the recognition of ventifacts in the sedimentary record, based on a critical examination of the diagnostic features commonly used to distinguish them, and on new studies permitting to suggest additional criteria.
Firstly, the sedimentary contexts favourable to finding ventifacts are evaluated. Then, the distinctive characters that could be seen on each one are treated from surface features, medium-scale features (different types of pit especially), and general shape, i.e. in order of increasing alteration of the original appearance. In addition, wind-worn non-mobile substrates are discussed.
3 Sedimentary settings
In modern environmental, most ventifacts are found in desert pavements (synonyms of regs, Cooke et al., 1993), which are typically monolayer (only one-clast thick). Some of these are formed by deflation: lifting and removal of fines particles and sand by the wind (Cooke, 1970; Fig. 1). At first, the deflation lag is more or less discontinuous, but once the clasts become closely packed in the horizontal plane, they protect the sediment below from further erosion.
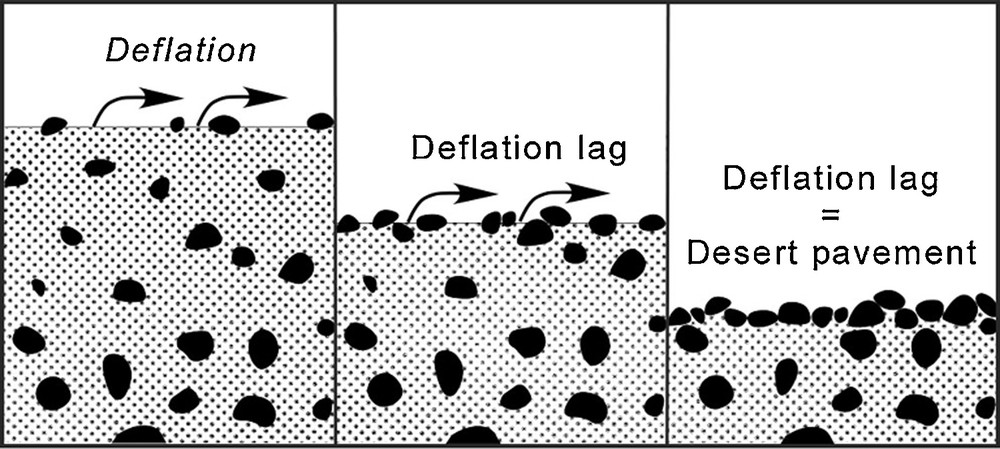
Sketch showing the genesis of a desert pavement by deflation.
On geological outcrops, thin gravel beds are frequently supposed to correspond to these deflation lags and thus are thought to be the best place to find ventifacts. The reality is generally disappointing because monolayer lags may also be formed usually in more dynamic subaquatic environments (Fig. 2). The most basic sedimentological structure characterizing gravel river beds is a coarse surface layer (called either armour or pavement), often not much more than one or two grains in thickness (Bray and Church, 1980; Charlton, 2008, fig. 7.4; Richards and Clifford, 1991; Fig. 2A). Along the coasts, structures of similar appearance are formed by wave action (Zecchin et al., 2011, fig. 9D; Fig. 2B) or even by tsunamis (Goff et al., 2012, fig. 1).
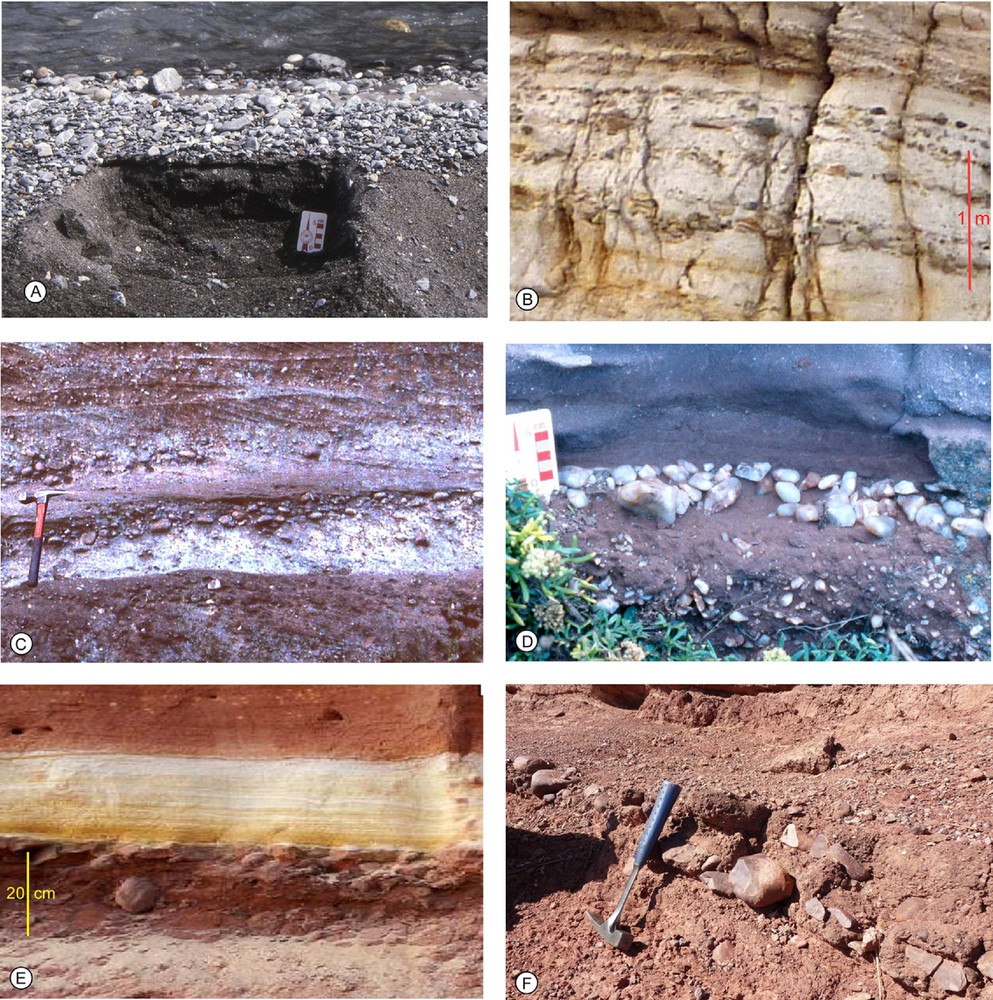
Different types of pebble lags. A. Fluvial lag on the side and top of a longitudinal bar. Bed of the Var River, SE France. B. Coastal marine lags deposited in the swash zone. Pleistocene, Port Ghalib, Egypt. C. Pebble layer, without ventifacts, at the top of a braided bar. Lower Triassic, Vosges Massif, NE France. D. Local palaeoreg resulting from sandblasting on a fluvial lag type A. Lower Triassic, Sardinia, Italy. E. Extensive Triassic palaeoreg at the top of Budleigh–Salterton Pebble Beds, overlain by yellow wind-deposited sands of the basal Otter Sandstones, Devon, UK. F. Widespread palaeoreg induced by a long hiatus in sedimentation. Lower Triassic, Argana basin, Morocco. In B and D, the scale card is 5 cm high. Masquer
Different types of pebble lags. A. Fluvial lag on the side and top of a longitudinal bar. Bed of the Var River, SE France. B. Coastal marine lags deposited in the swash zone. Pleistocene, Port Ghalib, Egypt. C. Pebble layer, without ventifacts, at ... Lire la suite
In contrast to monolayers, well-defined gravel layers several clasts thick (‘desert pavements’ of Rodríguez-López et al., 2010, fig. 10) cannot be formed by deflation, but such structures are common in coastal environments, either marine or lacustrine (Bourgeois and Leithold, 1984; Clifton, 1973, 2003; Zecchin et al., 2011, fig. 10). These ‘selection pavements’ (Bluck, 2011) are formed under wave hydrodynamics.
During the Early Triassic, in European and North African basins, the climate was, for a while, warm and hyper-arid (Péron et al., 2005), and sedimentary successions display fluvio-aeolian deposits including palaeoreg surfaces (Bourquin et al., 2007, 2009; Durand, 1972, 1979; Linol et al., 2009). Gravel pavements such as those seen in Fig. 2C are very common, but they never show any particular concentration of ventifacts, although the latter are occasionally present everywhere, in reworked state. Actually, undisturbed palaeoregs (Fig. 2D–F) are very rare; they can be buried by bimodal supermature sandstones (Folk, 1968), also the product of the desert floor, as at the Fontenoy site. Usually, ventifacts occur in the geological record in various reworked states, sometimes scattered and sometimes more or less gathered in ‘nests’. In most cases, they are found within fluvial sedimentary units totally devoid of palaeosols, where overbank deposits are clearly depleted in clay, but rich in aeolian quartz sand. As far as we know, the ‘reg type’ (McFadden et al., 1987), so common in modern deserts (Laity, 2008), was reported in only one case (Wright et al., 1991).
On the other hand, it is also noteworthy that, in some cases, a prolonged climate change could lead to the incorporation of pre-formed ventifacts in soil profiles of semi-arid type, where they are usually somewhat damaged by dissolution and/or fracturing in situ (Durand and Meyer, 1982).
4 Surface features
Very usually ventifacts are said to exhibit a polished aspect, and this criterion is often used as a clue for ventifaction on stones yielded by the geological record, but its usefulness deserves discussion.
4.1 The problem of ‘polish’
This question will be discussed separately for recent ventifacts and those found in ancient sedimentary series, where additional processes have to be accounted for.
Beyond the fact that it seems that the term ‘polish’ is applied to significantly different aspects, depending on the authors (Bather, 1900, p. 410), it should be pointed out that it is commonly known that artificial sandblasting is used to manufacturing frosted glass and not to polish anything (see physical experiences in Whitney and Dietrich, 1973). Since a real ‘windpolish’ was actually reported many times on rocks from both hot as well as cold deserts, it can be concluded that, even if polish forms more easily on the very smooth surfaces of ventifacts, its genesis definitely postdates the period of shaping by corrasion (“polished-appearing surfaces on ventifacts tend to lose their gloss when subjected to sand abrasion”, Whitney and Dietrich, 1973). Its origin can be reasonably attributed to the abrasive action of wind-driven suspensions of dust and/or ice particles (Christiansen, 2004; Schlyter, 1994; Whitney and Dietrich, 1973).
On the other hand, there were numerous cases of confusion with different types of very thin coatings (Dorn, 1998, 2009) overlying former corrasion surfaces, and which could be easily removed by sandblasting: desert glaze (Fisk, 1971) and rock varnish (Oberlander, 1994), more frequent, and often called ‘desert varnish’ albeit incorrectly because it also occurs in other settings. In any case, regardless of the nature and origin of the ‘polish’ noticed on Quaternary ventifacts, it has little chance to remain intact in the geological record owing to overprinting by diagenetic phenomena.
On ancient ventifacts, and even fluvial pebbles (Fig. 3), embedded in a clayey matrix, a true mechanical polish can occur after burial, probably related to tectonic deformations (Clifton, 1965; Judson and Barks, 1961), but also perhaps to differential compaction (Leonard et al., 1982). In many tectonic situations, preferential shear stress in a fine-grained matrix leads to the displacement and rotation of pebbles and cobbles instead of fracturing, producing a tectonic polish and microstriations. We can see an outstanding example in the Riba de Santiuste Triassic conglomerates (Guadalajara, Spain), where in some beds, fluvial cobbles show both solution pits at their contacts and a well-polished surface all around, whereas a few tens of meters away crops out a layer rich in ventifacts that show no special polish.

Fluvial cobble from a sandy-clay channel-fill, basal Miocene, SE France. The curved faces (F) showing radial fissures (rf) (Bourke et al., 2007) are characteristic of the breakdown of very fine-grained homogeneous rock. Preservation of these delicate features is evidence that aeolian abrasion (i.e. corrasion) did not occur. Note the diagenetic polish, best developed on the faces than on the well-rounded edges (E). C. Remnant of a cortex around the block of flint in the source rock.
4.2 Clast polarity
Despite the fact that, as seen above, no peculiar surface texture is useful per se to identify ventifacts found in the geological record, comparison of surface features on different sides of a single clast proved very helpful. Whitney (1978) had already noticed on palaeo-periglacial ventifacts that their bottoms were quite distinctive from all other surfaces. The great majority of ventifacts we studied, from any provenance, exhibit a polarity resulting from the contrast between a smooth upper side and a more or less irregular lower side, which allows us to easily recognize even non-faceted forms of ventifacts (Fig. 4A). In some cases, the underside of such clast (termed ‘polarized’ clast herein) retains the same aspect as that of the original clast: a rough fracture surface (Fig. 4A) or moderately smoothed surface common on fluvial cobbles, but in many other cases, it bears erosion traces (Fig. 4B) that can have very particular forms (Section 5.3).

Ventifacts showing a clear polarity, Lower Triassic. A. Quartz from Alpes-Maritimes, SE France. B. Quartzite from the Vosges Massif, NE France.
It must also be recognized that there are bipolar ventifacts, with a similar morphology on both sides (Fig. 7B), which implies an overturning of the object during ventifaction or between two distinct periods separated by a very short phase of aquatic reworking.
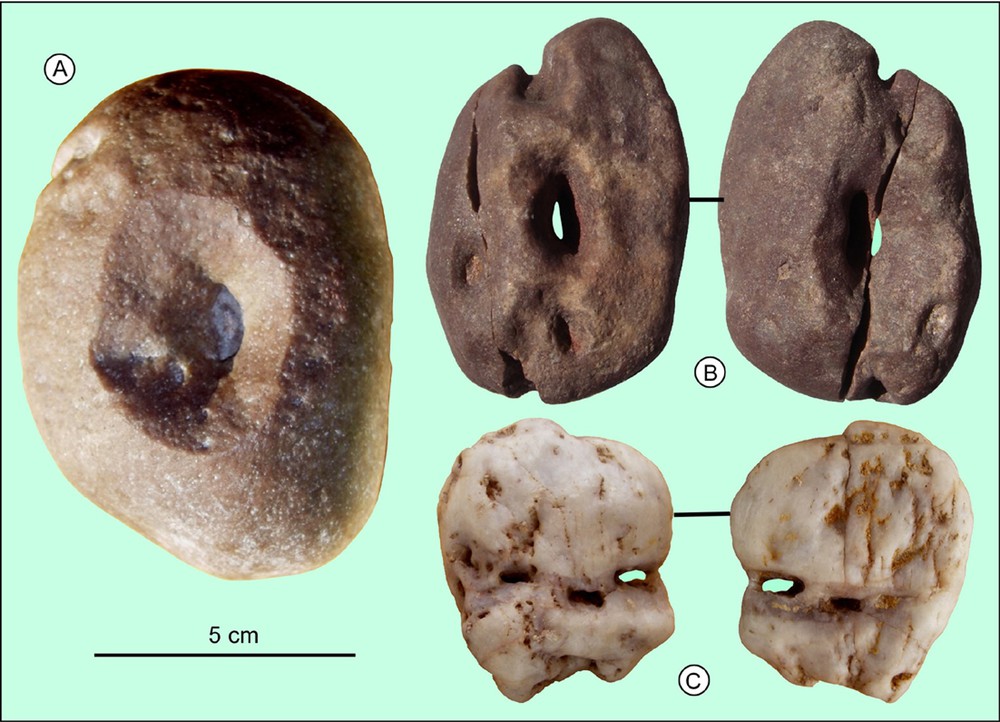
Vortex pits. A. Coarse-grained quartzite ventifact with a central vortex pit 15 mm deep. B. Fine-grained quartzite, bipolar, perforated ventifact. C. Quartz ventifact with lateral perforation. Specimens from different localities in the Lower Triassic from the Vosges, NE France.
5 Mesoscale features
Here is ranked among the mesoscale features any macroscopic unevenness affecting the faces or facets of a pebble or cobble. They include classically: pits, flutes and grooves (Sharp, 1949). Although not enough studied so far, they are very useful in discriminating between ventifacts and water-worn stones, even after a significant aquatic reworking. This is because mesoscale features are very different depending on the mode of wear of large clasts in water (attrition) or air (corrasion).
In water, gravel in transport wears out almost exclusively by rubbing against the bedrock and striking one another; sand grains are generally inefficient (Kuenen, 1956). Thus, the sharp edges of the clast become rounded very quickly, while the large concavities are less impacted (Fig. 3) and narrow re-entrants keep their pristine appearance (Section 6, Fig. 16A).
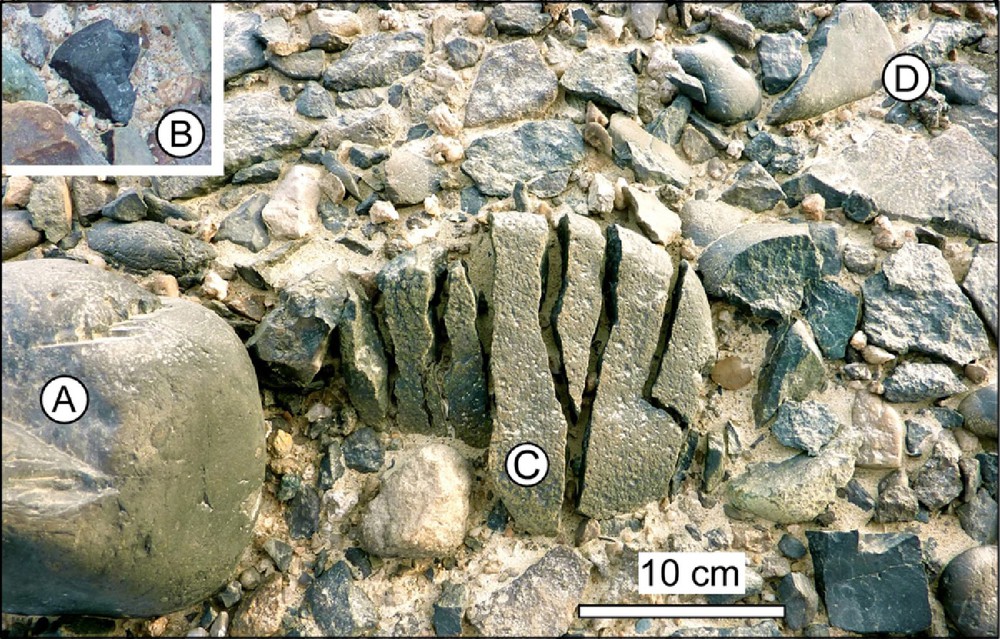
Close-up view of a present desert pavement, located at the top of the section partially shown in Fig. 2B; Pleistocene, Port Ghalib, Egypt. A. Rounded cobble with raw re-entrant surfaces; this is evidence that corrasion did not act on this reg. B. Dreikanter-like clast resulting from pure fracturing. C. Quartzite cobble affected by vertical cracks caused by directional insolation. D. Broken-round clast susceptible to being shaped as the most common type of einkanter encountered in the French Triassic (Fig. 17: 12–13).
In air, it is generally believed that corrasion is only produced by the direct impact of sand grains in saltation. The first consequence is that the faces of clasts with petrographic heterogeneities do not wear evenly, as the softer part is preferentially removed. This clue is generally the best sign of ventifaction on rock fragments that were never or scarcely transported (Fig. 5A–B), although this may also easily appear on pebbles that were already well rounded (Fig. 5C–D). Moreover, because sand grains are also dragged into vortices (Fisher, 1996; Whitney, 1978, 1983; Whitney and Dietrich, 1973) and can rebound on the wall of cavities (Várkonyi and Laity, 2012), corrasion is able to reach and deepen the most depressed areas at the surface of any pebble or cobble.
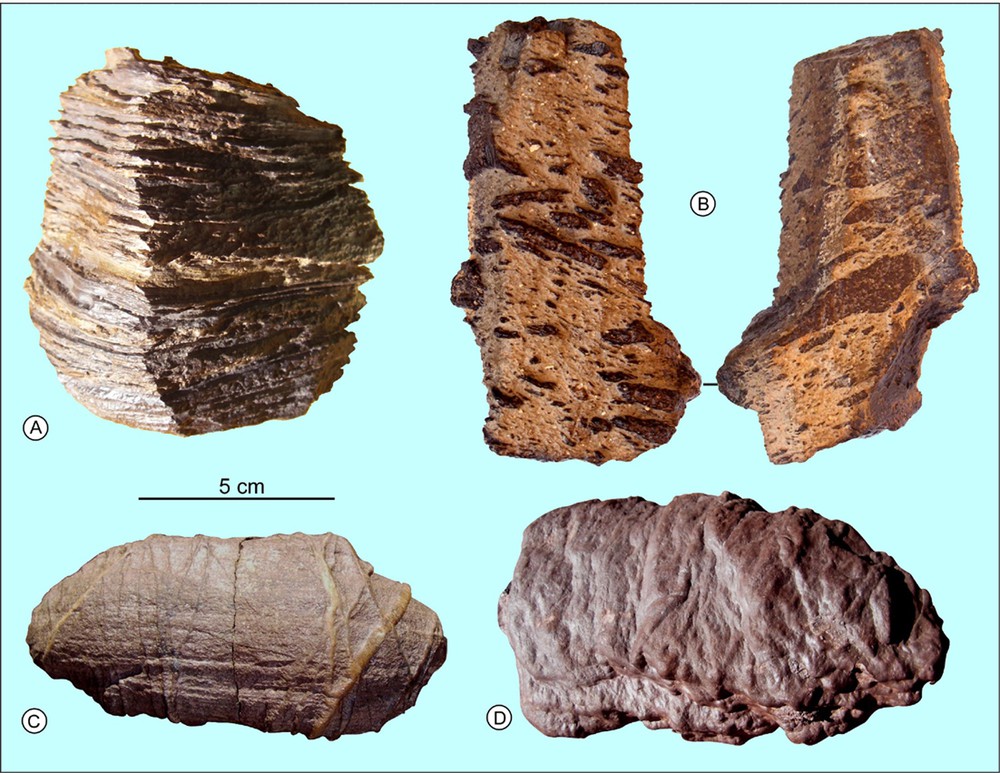
Highlighting of petrographical inhomogeneities of clasts by corrasion (differential etching). Upper part: ventifacts from modern regs, Mauritania. A. Ferruginous quartzite. B. Pyroclastite. Lower part: ventifacts from the Lower Triassic alluvial palaeoreg of Fontenoy-le-Château (Vosges, France). C. Quartzite with quartz veins. D. Quartzite with bioturbated structure.
5.1 Classical features
It is common to distinguish three basic types of mesoscale features on ventifact surfaces, between which transition forms exist: pits, flutes and grooves (Greeley and Iversen, 1985; Knight, 2008; Laity and Bridges, 2009; Sharp, 1949; Várkonyi and Laity, 2012; Whitney and Dietrich, 1973; Fig. 6). As originally described, the pits are irregularly shaped depressions, more or less circular, “from the size of a pencil point to an inch in diameter and depth” (Sharp, 1949). Flutes are scoop-shaped in plan and U-shaped in cross-section, and grooves are longer, and in principle, open at both ends.
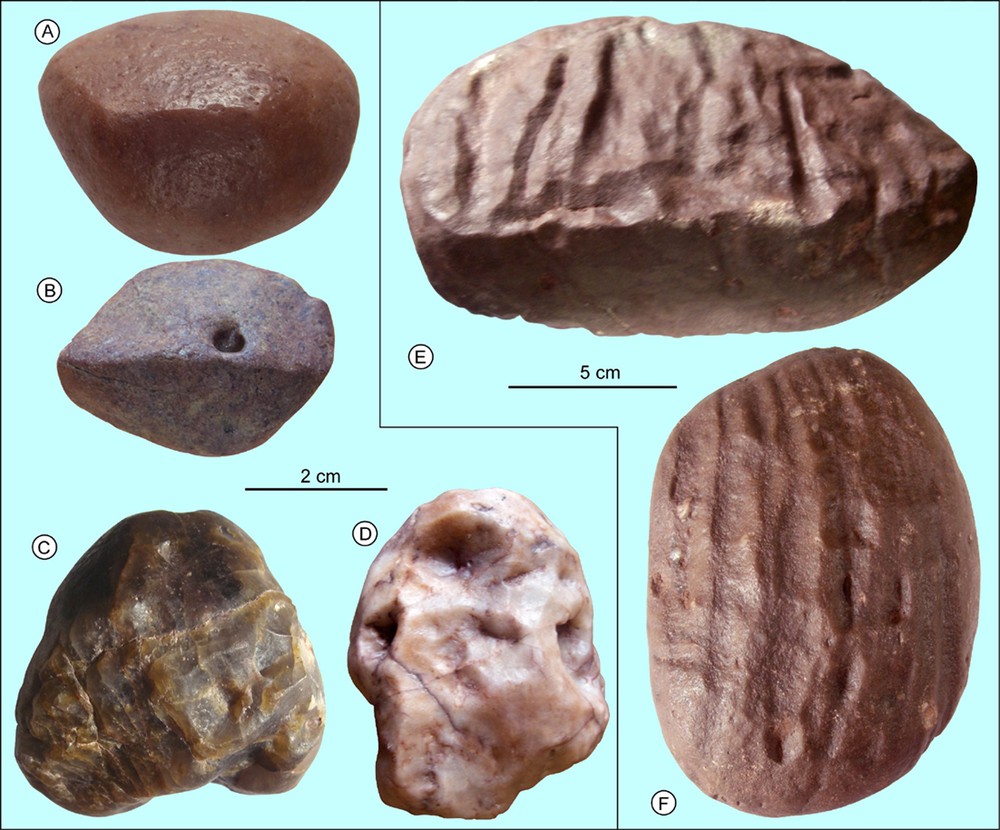
Examples of classical mesoscale features on ventifacts. A. Quartzite with surface pitted by preferential removal of feldspars. Triassic, Vosges. B. Quartzite with an isolated pit of unknown origin. Pleistocene, Almeria, Spain. C. Quartz where a few coalescent pits tend to take a polygonal shape. Triassic, SE France. D. Quartz with large but shallow pits. Lower Triassic, Vosges. E. Quartzite cobble with a long aeolian ridge, grooves with a different direction on one face, and three pits on the other. Lower Triassic, Vosges. F. Quartzite with transitional forms from pits to grooves elongated following internal lamination. Lower Triassic, Vosges. Masquer
Examples of classical mesoscale features on ventifacts. A. Quartzite with surface pitted by preferential removal of feldspars. Triassic, Vosges. B. Quartzite with an isolated pit of unknown origin. Pleistocene, Almeria, Spain. C. Quartz where a few coalescent pits tend to take a polygonal ... Lire la suite
On all the ventifacts studied, these features are very rare. As noticed by Sharp (1949), their nature and density depend primarily upon lithologic characteristics, and rocks such as quartz and fine-grained quartzites have especially smooth faces. On the other hand, they are preferentially found on larger specimens (Maxson, 1940), and always seem to mark a locus of weakness. In particular, grooves follow very discrete pre-existing joints and thus can show very different directions on the same ventifact (Fig. 6E).
5.2 Vortex pits
The origin of the different pits is not always clear. However, Whitney (1978) experimentally showed that some rounded pits could be formed under the action of a vortex with an axis more or less perpendicular to a surface exposed to the wind. Although studied at the SEM scale (Whitney, 1979), and contrary to the claim of Viles and Bourke (2007a), these vortex pits were also recorded at the macroscopic scale (McCauley et al., 1980; Whitney, 1978). A central boss on the bottom, if preserved, could be a major recognition criterion (Whitney, 1979, fig. 2; Fig. 7A).
It seems that vortex location is controlled by the outline shape of the gravel, because if the latter was overturned, the formation of a new pit can occur at the corresponding locus of the counterpart surface, and on exception, it can totally pierce the stone that becomes a perforated ventifact (Whitney, 1978, fig. 2B; Fig. 7B–C).
In some cases, corrasion (or even a previous phase of fluvial attrition) has reached a pre-existing cavity (e.g. a geodic cavity common within quartz clasts). Then the opening takes a typical funnel shape (Fig. 8A–C) that can be also attributed to a vortex associated with the centrifugal ejection of sand grains having penetrated into the hole. It is also noteworthy that the wall of the cavity, with well-formed crystals, is also smoothed (Fig. 8D–E), whereas during fluvial transport, the shiny facets of such crystals are well preserved.
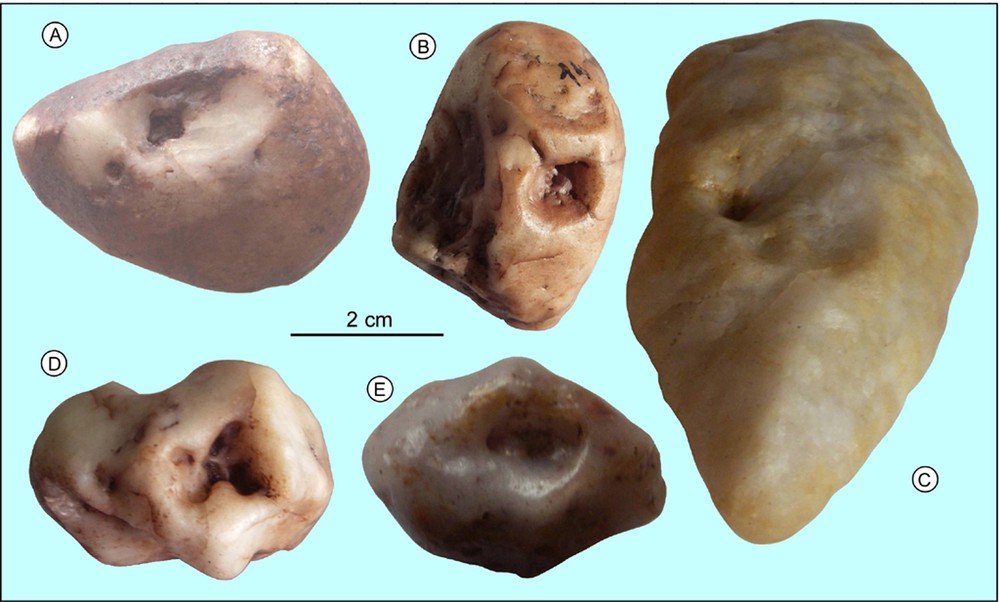
Aspect of geodic cavities on quartz ventifacts. A–C. Funnel-shaped openings. D and E. Cavities with a smoothed wall. Specimens from different localities in the Lower Triassic from the Vosges.
5.3 Rubbing pits
Approximately 2% of the quartzite pebbles and cobbles from the Fontenoy palaeoreg exhibit circular hard-edged pits, most commonly isolated and between 5 to 15 mm in diameter, similar to the prints left by a ball on a block of plasticine (Fig. 9). A morphometric study showed that their shape is exactly that of a spherical cap. These pits, which never exist on quartz clasts, can be found in almost all sites with Triassic ventifacts. Unlike the vortex pits, they are located on the underside of polarized ventifacts as well as on other stones showing no trace of corrasion, which should therefore be more or less embedded during pit formation.

Examples of ventifacts with rubbing pits. Lower Triassic of the Vosges Massif, NE France.
In terms of their shape, and given that they only occur on quartzite, these pits resemble those characterizing the ‘pitted pebbles’ described by Kuenen (1942), produced by pressure solution under tectonic stress (Cortés et al., 2002; Klein, 1963; Ruano and Galindo-Zaldívar, 2004), and renamed ‘cratered pebbles’ by Ernstson et al. (2001). Actually, they differ by the nature of their filling, which consists of sandstone matrix and not a single quartz pebble. Moreover, the sharp boundary and the absence of pale halos (iron leaching), associated cracks and remnants of a possible ‘printing’ pebble, show that the clasts carrying them are not ‘impressed pebbles’ that have been reworked.
As far as we know, the pits described above have never yet been reported, albeit an example appears very likely in Whitney and Dietrich (1973, fig. 2A). Similar features, although larger and formed in subaqueous setting, were described by Schoeller (1952) under the name of ‘cupules de rodage’. They are due to the oscillation, under the effect of an ascending water flow, of hard-rock pebbles trapped in intergranular spaces of a boulder-filling, at the bottom of a karst chimney (Fig. 10A).

Explanatory sketches for the genesis of the two types of rubbing pits. Not to scale. A. In aquatic setting. B. In aeolian setting.
A similar mechanism may be invoked for the formation of the aeolian pits discussed above (Fig. 10B), and for which the name of ‘rubbing pits’ is proposed herein. In both cases, there is, at first, settling of coarse heterogranular sediment, by simple free-fall in the case described by Schoeller, and by streamflow in the case of the Triassic regs. Then, intervenes a flow that is unable to shift the largest clasts, but can sweep away the smallest clasts and move the trapped intermediate-sized clasts. Each rubbing pit could thus be produced by a small quartz pebble bouncing around the intergranular cavity between the quartzite phenoclasts, in the same way as a pea within the chamber of a whistle. A basic experiment that we realized with pulses of compressed air and a small spheroid quartzite-pebble was able to replicate these features on balls in plaster.
It is suggested that the process inferred for the formation of rubbing pits is at the origin of, or participates in, a particular fashioning of the underside of some ventifacts. The morphology of these faces is seldom discussed in the literature, although “a large proportion of ventifacts show evidence of wind erosion on all surfaces, including bottoms” (Whitney and Dietrich, 1973). Apart from the possible presence of rubbing pits, strictly located there, the lower face may be almost entirely converted into an even supporting plane (Kuenen, 1928) with a ridge running in a curved line right round it (Fig. 11A–B). In some cases, this surface is gently corrugated (Fig. 11B) or shows elongate and shallow pits or flutes (Whitney and Dietrich, 1973, fig. 2F; Fig. 11J); grooves may even appear rarely (Fig. 11I). Although uncommon, one of the most characteristic features of the underside of ventifacts is an irregular somewhat deep carving, which can result in a markedly concave shape (Whitney, 1978, undated, figs. 29 and 31; Whitney and Dietrich, 1973, fig. 1F; Fig. 11C–G). At least in some cases, this could be due to the work of sand-laden turbulences initially centred on rubbing pits whose original stone would have escaped (Fig. 11H). By contrast, Lindsay (1973) attributed this morphology, observed under present ventifacts in Antarctica, to salt weathering.
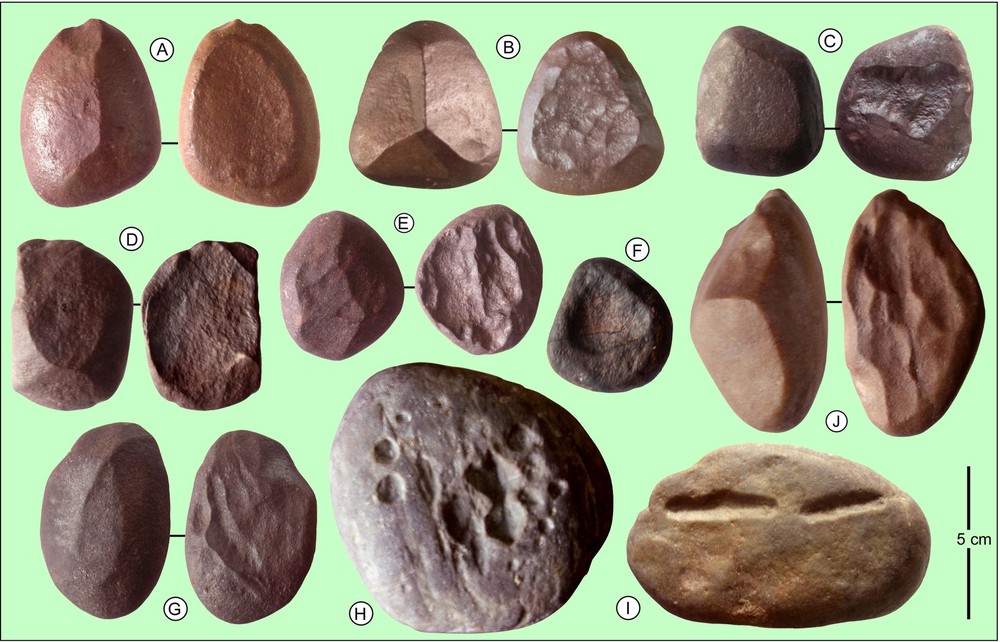
Various aspects of the underside of quartzite ventifacts. A and B. Specimens with a more or less regular supporting plane. C–G. Different types of carved surfaces, up to the formation of a concave face (D and F). On the underside of C, note the preservation of a sub-central pillar, probably corresponding to a point of contact with an underlying pebble. H. Development of corrasion starting from rubbing pits. I. Grooves following a pre-existing tectonic joint. J. Elongate and shallow coalescent pits (flutes).
5.4 Impact features
Many ventifacts carry traces of percussion (Fig. 12), but this has very rarely been reported (Bourcart, 1928, fig. III1a; Lindsay, 1973). On ventifacts found near the modern ground surface, these traces seem to have often been regarded as artefacts resulting mainly of tillage practices.

Impact features on Lower Triassic ventifacts. A. Quartz ventifact with tiny incipient cones located only on one side (here left) of the main ridge. B–J. Quartzite ventifacts with percussion facets. Arrow tips show the impact points. I. Flake corresponding to a fragment of ventifact. J. Ventifact formed from the core left by the detachment of small flakes.
The most subtle features are tiny cracks appearing as crescent or circular-shaped surface markings (Fig. 12A), from 1 to 5 mm in diameter, that are only visible on quartz and are known as ring cracks, shatter marks, or incipient ‘hertzian’ cones (Bourke et al., 2007), which result from clast-to-clast collisions. These traces were considered to be an index of marine abrasion by Cailleux and Tricart (1963), although they have been repeatedly identified in fluvial settings (Bourke et al., 2007; Conybeare and Crook, 1968; Klein, 1963; Reineck and Singh, 1980). The aeolian scars differ from their fluvial counterparts by their smaller sizes, and mainly in their preferential location on the upper face of a clast, and even sometimes on only one side of the main ridge (Fig. 12A).
The percussion-fracture facets, which are more or less conchoidal in shape, are more apparent, especially on quartzite ventifacts, and most often occur on the acute edges of clasts (Fig. 11B–J). Some, with radial fissures, have still kept a rough appearance (Fig. 11B) while others are smoothed out (Fig. 11G); occasionally up to four distinct phases of percussion can be distinguished on the same clast according to the degree of smoothing. Several small pebbles can be interpreted as flakes or chips separated by percussion (Fig. 11I) and others as residual cores (Fig. 11J). All these features (Section 6.2) are unlikely to have formed during an aquatic reworking because given the absence of boulders and large cobbles in this deposit, shocks between clasts would have been too buffered in water to produce the detachment of flakes (Bourke et al., 2007).
5.5 Vermiculations
Loose stones with a peculiar vermiculated surface, covered with tiny ‘rills’ (Fig. 13), can be found on the ground of many modern desert areas. Travellers usually attribute this aspect to wind abrasion, according to an opinion stated by Whitney and Dietrich (1973) among others. Nevertheless, these gravels are not ventifacts. Their origin was already well established as early as the 1940s (Cailleux, 1942; King, 1936; Maxson, 1940); more recently, Viles and Bourke (2007b) ranked their specific carving among ‘microsolutional features’ and showed examples formed on pre-shaped ventifacts (their figure W9).
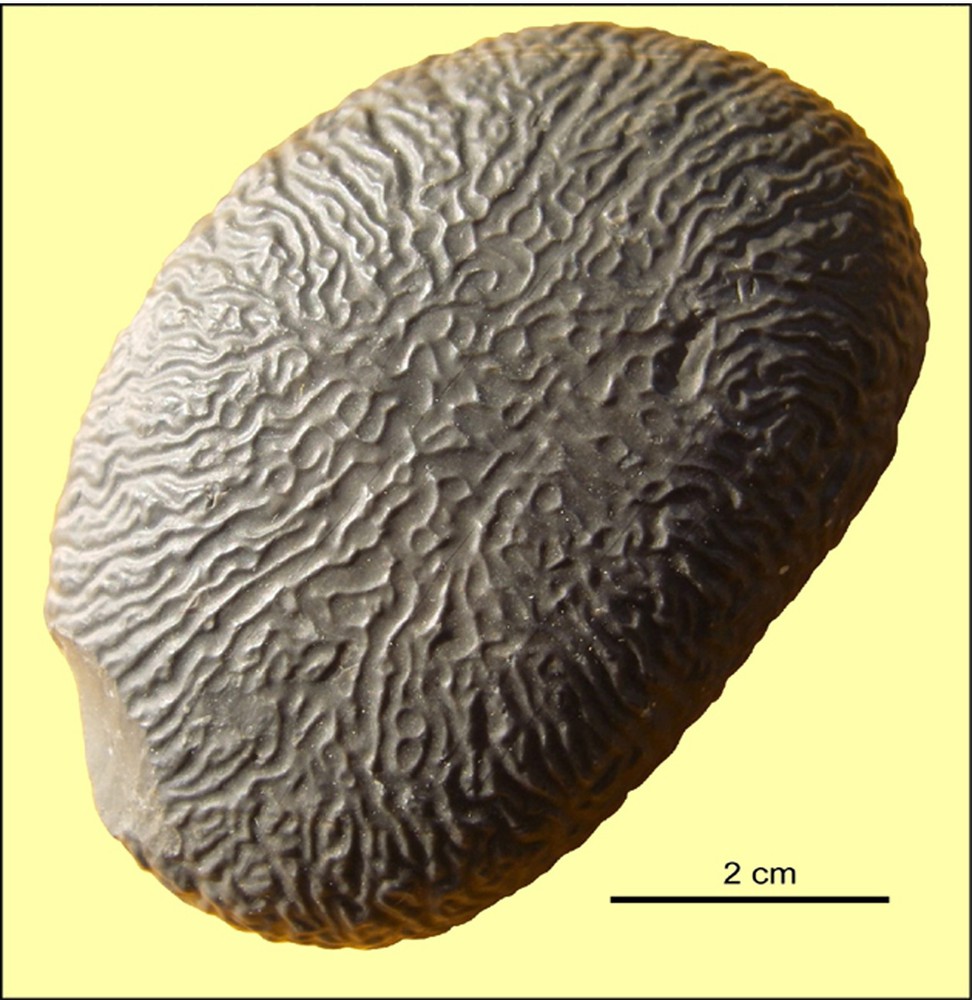
Small vermiculated limestone cobble or ‘rillstone’. Huqf Desert, Sultanate of Oman.
These stones are non-porous limestone (or dolomite) pebbles corroded by dew droplets that frequently form at night in many deserts, especially coastal-type ones such as the Atacama and the Namib. At sites sheltered from the wind, dew flows solely by gravity, causing rills with radial arrangements. On pebbles exposed to a moderate wind, relatively constant in direction, the wind-driven drops create more or less parallel grooves. In areas of vortices or changing winds, the drawings are often more or less labyrinthiform. Where the wind is stronger, dew evaporates too quickly to corrode and ventifaction can occur, but only if sand is available.
This fashioning can also form in landscapes with a discontinuous pseudo-steppe vegetation cover. In siliciclastic sedimentary successions, it is not surprising to never find such ‘rillstones’, but their occurrence in calcareous fanglomerates is not to exclude.
6 General shape
The sizes of ventifacts can vary to a considerable extent. They appear to be limited only by the size of the original clasts. In Triassic red-beds, the biggest of these may be clearly wind-worn (Fig. 14A), and in periglacial environments, where boulders are common, ventifacts can be several metres long. In some places, small ventifacts may be encountered (Fig. 14B); miniature ventifacts, less than 2 mm across, have even been reported (Higgins, 1956; Thompson and Worsley, 1967). Based on the data of the previous author and field observations in northwestern Sardinia, it appears that the scarce Lower Triassic examples of tiny ventifacts could have been shaped while they were immovable, stuck in a dried mud puddle.
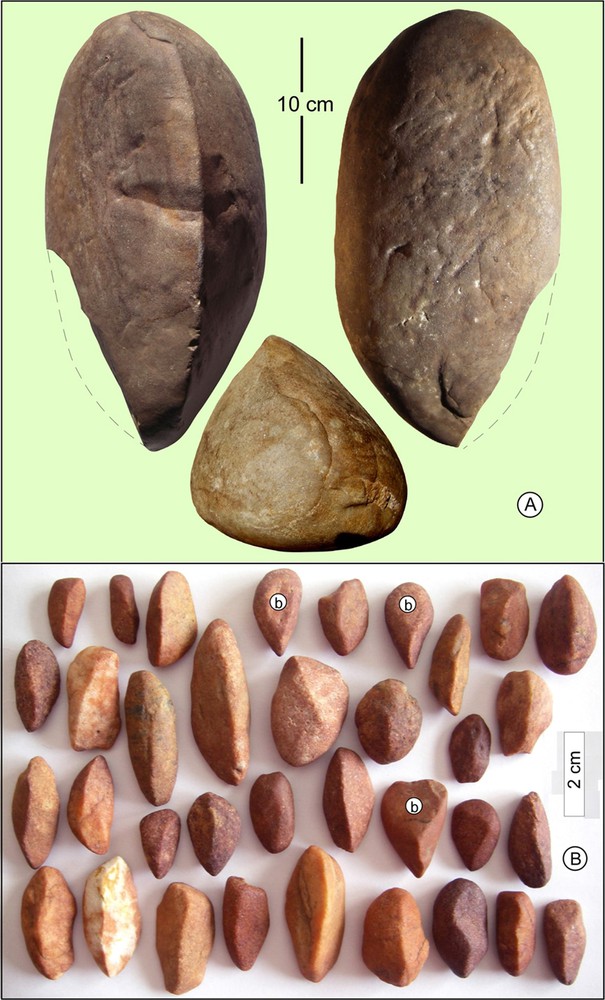
Size range of ventifacts. A. The biggest ventifact (quartzite einkanter) found in the Triassic of NE France. Although it is clearly reworked, its polarity, the ‘roof’-shaped cross-section, and a groove along a joint are still recognizable. B. ‘Miniature’ ventifacts (quartzites) from the Pleistocene of Almeria (Spain). Note the three babouche-shaped ventifacts (b).
The general outline, in plan view, of ventifacts may show very angular and/or extremely irregular shapes that are unable to withstand transportation by streamflow (Bourcart, 1928, fig. VI.1; Maxson, 1940, fig. 11; Sugden, 1964, fig. 1; Fig. 15) and, when preserved, provide a good criterion for autochthony if transport by solifluction can be excluded. However, in the case of carbonate clasts, one must be very cautious, because very similar forms can be produced by simple chemical etching.
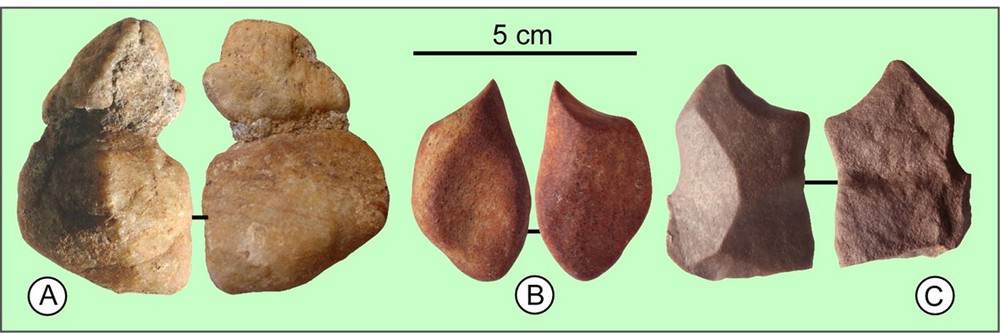
Examples of ventifact shapes that could not withstand a long transportation in streamflow. A. Slightly reworked quartzite from the Lower Triassic of Lombardy, Italy. B. Quartzite from the Pleistocene of Almeria, Spain. C. Quartzite from the Lower Triassic of Vosges, NE France; a part was broken on the outcrop.
6.1 Faceted ventifacts
In some instances, general shape can hardly be used to distinguish a ventifact from a faceted clast of another type. This occurs especially if one is faced with a stone never transported, or even a first-cycle sedimentary element, that usually shows a polyhedral form, with faces that are sometimes flat (tectonic joints of the mother rock) and sometimes slightly concave or convex (resulting from thermal or mechanical shocks). In these cases, it is only if the heterogeneities have clearly been emphasized by corrasion (Fig. 5A–B) that a ventifact can be identified. Conversely, the lack of corrasion may be demonstrated by the rough aspect of dihedral or trihedral re-entrants (Fig. 16A).
All polyhedric clasts show trihedral corners, which is why they are so often described as ‘dreikanters’ and are therefore interpreted as ventifacts (Viles and Bourke, 2007a, figs A1, A4, A5). Some of their faces commonly correspond to joint planes, mainly tectonic in origin. In contrast, facets carved into ventifacts by aeolian abrasion are rarely flat. As noticed by Higgins (1956), whereas in a vertical section they are more or less straight in profile, most are slightly to strongly curved in the plan view, so that the ridges between them are typically arcuate (Fig. 17: 1).
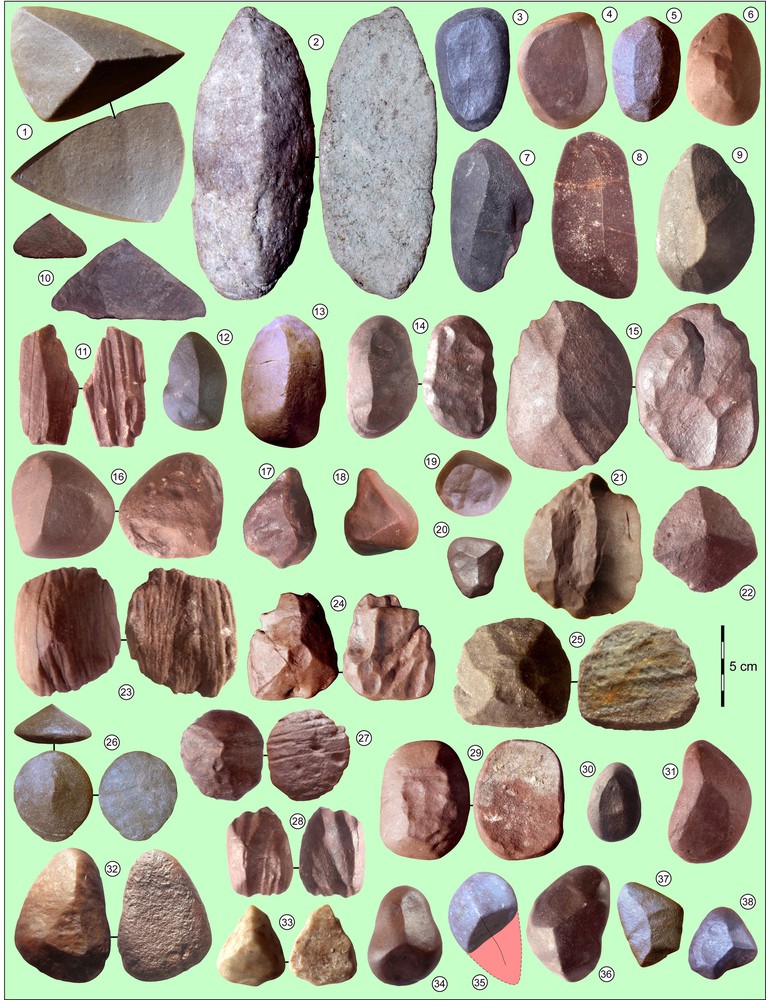
Typology of faceted ventifacts from the Lower Triassic of the Vosges Massif, NE France (unless otherwise). The selected specimens area made of quartzite (unless otherwise) because the ridges are hard to see without retouching on photographs of quartz ventifacts; for them, drawing is better (Durand, 2006, fig. 4; Durand, 2008, fig. 14). 1: polyhedral periglacial ventifact of unknown provenance. University of Rennes collection. 2: reworked triquetrous ventifact made of coarse-grained quartzite. 3 and 4: ventifacts with a ridge following the general outline. 5: ‘worn eraser’-type ventifact. 6: flat-topped ventifact. 7–9: einkanters. 10: transverse sections of einkanters from Fontenoy. 11: einkanter with a keel that is clearly independent from the internal structure. 12 and 13: the most classical type of einkanter type in the Triassic of NE France. 14: einkanter with supporting plane. 15: einkanter with large flutes on the underside. 16: globular einkanter. 17 and 18: einkanters with a sinuous ridge. Cañizar Formation, Spain. 19: globular einkanter with only one facet and an arcuate ridge. 20: ventifact with five ridges. 21: ventifact with two parallel ridges. 22–24: ventifacts with four ridges. 25: ventifact with multiple ridges (Walther's Vielkanter). 26 and 27: conical ventifacts. 28: multifaceted bipolar ventifact. 29: ventifact with a composite upper face and concave lower face. 30 and 31: two stages of evolution towards the ‘babouche’ type. 32: flat-topped ‘pre-dreikanter’ made of quartz. 33 and 34: quartz and quartzite dreikanters, the shaping of which was controlled by the original contour of the clast. 35–38: dreikanters resulting from the break on an einkanter. Masquer
Typology of faceted ventifacts from the Lower Triassic of the Vosges Massif, NE France (unless otherwise). The selected specimens area made of quartzite (unless otherwise) because the ridges are hard to see without retouching on photographs of quartz ventifacts; for ... Lire la suite
The action of corrasion is much easier to recognize on clasts that were already rounded during transport in a river or in the sea. What is typical in this case is the occurrence of specific ridges clearly limiting newly-formed facets (Kuenen, 1928; Fig. 17). Following King (1936) we restrict the term ‘facet’ to surfaces cut by corrasion, that of ‘face’ being applied for the original surfaces of the clast. Ridges characteristics of aeolian wear are regular and clean, albeit blunt (Cailleux, 1942). Some are more or less sinuous and generally fade towards the base of the clast (Whitney and Dietrich, 1973, fig. 4; Fig. 17: 7–9, 12–14 and 34), whereas others tend to follow the general outline (Fig. 17: 3–5). The number of main ridges depends partly on the initial shape of the clast (Heim, 1888; King, 1936; Kuenen, 1928, 1960; Fig. 17: 20–27), and partly on the wind regime (Schoewe, 1932; Whitney, 1983). King (1949) concluded that, with angular material, the original shape exercises considerable control over the final form, whereas with originally rounded materials, the wind direction controls the orientation of the facets.
The simplest shape of faceted ventifact is that of an einkanter (Walther, 1911; Figs. 14 and 17: 7–19). It has only one ridge, often referred to as ‘keel’, generally more or less parallel to the longest dimension. The einkanter is also the most common type of faceted ventifacts (De Geer, 1886; Fisher, 1996; Gillies et al., 2009; Schoewe, 1932). Counting on deflation pavements in Poland indicated an average of 70% of ventifacts with two facets versus only 20% of ventifacts with three facets (Antczak-Górka, 1999).
These pebbles may show a single wind-worn facet (Fig. 17: 17–19), but often bear two opposite ones, and when they have an overall elongate shape, may bear three or even more. In the first case, they have a triangular cross-section, which earned them the epithet ‘triquetrous’, and may have either one or two acute ends (Figs. 14B and 17: 2). Wade (1910) erroneously applied the name dreikanter to this last morphology, described by him as a ‘brazil-nut’ shape, and this confusion still persists today occasionally (Flores et al., 2011). More recently, Whitney (1983, figs. 9 and 10) restricted the use of ‘triquetrous’ to double-pointed forms, and distinguished as ‘brazil-nut’ those with a single point; the latter tends to be bulkier at one end (windward) and to have four or more faces instead of the three that characterize the triquetrous forms. A special shape, named the babouche form herein, derived from the brazil-nut form, can be distinguished (Whitney, undated, fig. 36; Fig. 14B, b), with a keel located at the acute end and an enlarged, somewhat sharp orthogonal basal ridge at the other end.
It was shown that the triquetrous shape is normally derived from a flat-topped precursor (Schoewe, 1932; Whitney, 1983, fig. 10; undated, fig. 39). Schoewe (1932) concluded from his experiments that this type represents the most complete ventifact end-form, all others being considered as partially developed. However, Berg (1931) noted, on natural specimens, that there could be numerous longitudinal edges and therefore, he thought that the end-member would be more like a spindle shape.
At some localities, forms related to the triquetrous shape are dominant (Fig. 14B). This has been reported in modern environment settings, from periglacial deposits (King, 1937) as well as hot deserts (Holmes, 1978, fig. 23.9; Wade, 1910, fig. 2). Conversely, they are scarce in the studied Triassic deposits; it seems to depend above all on the initial shape of the clasts.
During the Triassic, well-rounded fluvial cobbles that were more or less ellipsoidal in shape seem to have been cut into something resembling slices, perhaps by physical weathering processes related to directional insolation (Eppes et al., 2010; McFadden et al., 2005; Fig. 16). The resulting vertical cracks isolated clasts with plane surfaces suitable to ensure the stability required for the formation of new facets. As commonly observed during the course of the shaping of ventifacts, facets tend to recede more rapidly in their upper part than in their lower (Greeley et al., 2002); thus clasts from the medial part of the original cobble tend to take first a shape of ‘worn eraser’ (Mary, 1964; Fig. 17: 4–5). Along with the decrease of the surface of the apical facet, the latter tends to become more or less concave (Fig. 17: 24), probably as a result of the vortex invoked in Section 5.2. The pieces from the ends, with a profile like that of half an egg, were special precursors for the formation of the most common ventifacts in these deposits (Fig. 17: 7–9 and 12–14). Those in the form of a spherical cap would be the origin of conical ventifacts (Fig. 17: 26–27); their shape, always independent of the internal structure, is particularly characteristic of wear by sand-laden wind.
Special attention must be paid to the use of the term dreikanter. Although (or because?) widely applied, it has become ill-defined. Some confusion was introduced very early by Bather (1900) who proposed the dreikanter as the type of faceted pebbles; moreover, although he illustrated dreikanters that meet the definition of German geologists (figs. 1 and 2), he qualified them as ‘triquetrous’ in the text. Originally, Berendt (1885) proposed the term to refer to pyramidal stones showing ‘drei Kante’ (Kante = ridge) on one side. A dreikanter must therefore be defined by the presence of three ridges converging to one apex point (Fig. 17: 33–34), and not the presence of three facets, as is often indicated especially in the English-language literature, or the presence of three corners (Barnes-Svarney and Svarney, 2004).
As stated above, dreikanter-shaped true ventifacts are not very common in aeolian settings. They may have developed from a clast that was already triangular in outline (Fig. 17: 33–34), and in these cases, the first stages were often those of a flat-topped pebble (Fig. 17: 32). Nonetheless, in many others, they result from a particular set of circumstances: e.g. if an einkanter breaks (Fig. 17: 35–38). But it should be emphasized herein that if the ‘classic’ dreikanter shape is not the most characteristic shape of a ventifact, this is above all because this shape can result from mechanical processes, predating a fluvial transport, that have nothing to do with aeolian wear (Jones, 1953). They could originally be chipped or broken fragments from the corners of rhombohedral or sub-cubical joint blocks (Fig. 18) during their rapid descent on talus slopes upstream in the valley.
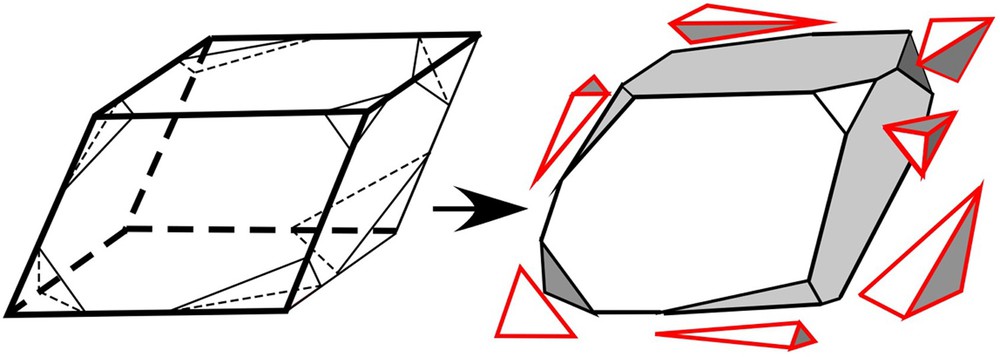
Genesis of dreikanter-like clasts by the cutting of tetrahedroid corners from raw blocks.
Redrawn from Jones, 1953.
6.2 Spheroid ventifacts
The identification of percussion marks on ventifacts (Impact features, Section 5.4) involves a search for the objects that were responsible for them. Since it seemed that there were more clasts with a high degree of roundness among the small pebbles, especially quartzite, on some outcrops rich in ventifacts, a morphometric study was undertaken to examine this.
All the pebbles of the Fontenoy palaeoreg were systematically collected until were gathered 100 quartzites and 100 quartz of the five length-classes, at intervals of 1 cm, between 6.5 and 1.5 cm. The three axes of each were measured; these data were plotted on a Zingg's diagram (Zingg, 1935), where each clast is represented by a dot, and were processed in two ways. The first consisted of drawing a density diagram for each size class, to determine the centroid of each population. The second way, inspired from Schleiger (1964), was used to present the results in more summarized fashion; this was accomplished by transferring every point onto a single diagram and then demarcating the areas of dominance of one size class over the others (Fig. 19).
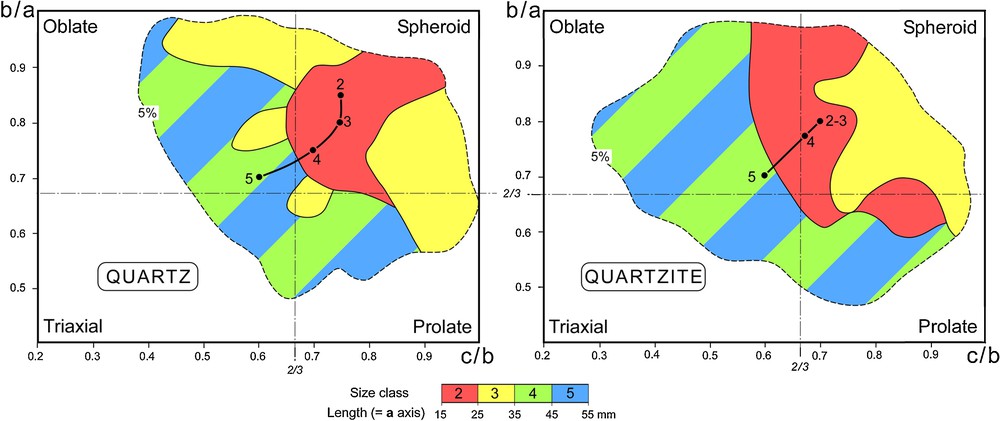
Morphometry of pebbles from the palaeoreg of Fontenoy-le-Château (Lower Triassic, NE France). Clasts dimensions: a: long axis, b: intermediate axis, c: short axis. 1–4: centroids of the four size classes.
For quartz pebbles, sphericity increases in an almost regular manner from the larger to the smaller sizes, while for quartzites, the most spherical shapes belong to the 25–35 mm class. The overall decrease in sphericity noted in the 15–25 mm class is related to the presence of numerous chips produced by collision in the depositional environment. Thus, the small quartzite balls found in association with faceted pebbles can be considered as spheroid ventifacts shaped by rolling and colliding with larger objects (Várkonyi and Domokos, 2011), a view already advanced by Mary (1964) and Karlov (1969). Their size is similar to that of the biggest clasts that can be transported in saltation by modern severe storms in cold or hot desert areas (McKenna Neuman, 1990; Sakamoto-Arnold, 1981).
7 Wind-worn non-mobile substrates
Sand-laden winds carve not only on loose stones, but also, of course, on other substrates as bedrocks, boulders, and anchored gravels; the resulting geomorphic features, described many times, are rather similar to those displayed by the upper side of classic ventifacts. Otherwise, for a long time, some researchers have reported instances of facets and other “ventifact-like” morphologies generated by the action of relatively coarse material transported by water, in streams or on the beach (Frankel, 1955; Kuenen, 1947; Lomas, 1907, p. 193; Taljaard, 1939). Kuenen (1947) coined the term ‘aquafact’ to describe these water-faceted objects. In our present state of knowledge, it does not seem that strictly geomorphic criteria exist for distinguishing them from the previous ones.
The problem is complicated by the fact that, even in some current cases, the origin of such forms is questionable. ‘Anchored ventifacts’, ‘bedrock-cut ventifacts’, and ‘ventifacted boulders’ have been described in temperate coastal areas, including the present-day intertidal zone (King, 1949; Knight, 2002, 2005; Knight and Burningham, 2001, 2003). Nevertheless, it should be noted that, in a similar environmental setting, facing the town of Etel (southern Brittany, France), the currently shaped rocks are undoubtedly aquafacts (Fig. 20). On ‘Men Du’ rocks, right at the narrow opening (only ~170 m at low tide) of a wide branching ria (22 km2), where tidal currents can exceed 5 m s−1, it is easy to watch, at low tide, the peculiar faceting process in action (Fig. 20A).

Aquafact morphologies at Etel, southern Brittany, France. A. Bedrock-cut aquafacts with the rising tide (current from right to left). B. Aquafacts in the lower intertidal zone. Sand and granules on the bottom move at each tide. C. Upper intertidal zone. Note the barnacles on the left facets, indicating a lasting tidal-current inequality. D. Aquafacted boulder with three keels. E. Giant water-faceted ‘dreikanter’ in the upper intertidal zone. In D and E, the scale card is 5 cm high.
In the geological record (see example in Watts, 1903), it is even more problematic than in present-day environmental settings to know whether the facets are of aquatic or aeolian origin; thus only a purely descriptive term should be used to refer to them: e.g. faceted rocks.
8 Conclusion
With this study, we hope to help recognition of wind-worn pebbles and cobbles yielded by geological series, through a combination of diagnostic criteria. In situ occurrence of such ventifacts is a valuable evidence of desert palaeoenvironmental conditions, although palaeoclimatic inferences cannot be put forward without other information provided by the sedimentary context. Moreover, from our knowledge, the well-preserved widespread palaeoregs seem to mark major stratigraphic discontinuities above a geomorphic surface remained a long time without vegetation as a result of a very dry climate, hot or cold. It must be emphasized that, partly due to the importance of diagenesis, no surface texture (as ‘polish’) is useful per se to identify confidently ventifacts found in the geological record. In contrast, a polarity in the appearances of wear is a major diagnostic criterion. Mesoscale features are also very important to the recognition of ventifacts. Hollows whose walls have been smoothed make it possible to identify them, even after a rather long fluvial reworking that would have rounded seriously the ridges. Rubbing pits and impact features provide also significant clues. Among wind-worn pebbles and cobbles, the most common shape (einkanter) displays only one ridge, and dreikanter-shaped true ventifacts are not very common. Thus, when in a layer, there are a high percentage of tetrahedroid forms, a non-aeolian origin should be suspected for them. For facets carved on unmovable substrates, only a sedimentological study of the associated deposits would allow us to know whether they are of aquatic or aeolian origin.
Further studies are still needed, especially in an attempt to discriminate ventifacts from hot deserts and those from periglacial environments, what is not possible in the present state of knowledge.
Acknowledgments
We thank the two reviewers, Jean-François Ghienne and Krisztina Sebe, for their constructive comments. Sara Mullin is acknowledged for post-editing the English version of the manuscript. This paper has been formatted according to the preceding guidelines of C. R. Geoscience.