1 Introduction
The need to understand how species respond to environmental variation has become critical as environmental changes have demonstrable ecological effects in many natural systems (Parmesan, 2006; Walther et al., 2002). Determining how individuals base key life-history decisions on environmental cues is important to predict how environmental changes will influence them. Phenotypic plasticity, defined as the ability of a single genotype to modify its phenotype under heterogeneous environmental conditions (Houston and McNamara, 1992), is fundamental to a plant's ability to cope with environmental change at various temporal and spatial scales.
Stomata regulate the permeability of leaves of terrestrial plants to gases (gas phase conductance), so that the leaves can absorb CO2 for photosynthesis without losing excessive water vapour (Raven, 2002). There is at present sufficient evidence to suggest that perennial terrestrial plants regulate their gas exchange (and thus CO2 uptake and water loss) by varying the opening of the stomatal pores (Young et al., 2006) and by changing the stomatal frequency on leaves (Gagen et al., 2011; Hetherington and Woodward, 2003). Thus, to optimize their resource use and evade costs of having excess stomata, plants could be expected to adjust stomatal frequency (Roth-Nebelsick, 2007). Amongst several environmental factors, atmospheric CO2 has often been observed to have a strong influence in the regulation of stomatal frequency (Chen et al., 2001; Hetherington and Woodward, 2003; Wagner et al., 1996; Woodward, 1987). However, most scholars agree with the view that responses are species-specific (Marchi et al., 2004; Tognetti et al., 2000) and include cases in which species do not respond at all or respond to increasing atmospheric CO2 by increasing, rather than decreasing, stomatal frequency (Hetherington and Woodward, 2003).
Although strong plastic (developmental) responses have been observed in stomatal frequency (stomatal density and stomatal index [SI]) (Royer, 2001), there is clear evidence of genetic control of the CO2 response (Jordan, 2011). Thus, in Arabidopsis thaliana, the HIC gene codes for CO2 responses in both stomatal density and index (Gray et al., 2000). The latter evidence strongly suggests that stomatal frequencies are determined (at least to some extent) by the genotype and implies that plants can regulate their stomatal frequency by phenotypic plasticity, allowing them to adapt to changing environmental conditions. For example, it is often observed that plants have higher stomatal density when growing at lower CO2 concentrations (Hetherington and Woodward, 2003; Woodward, 1987) or at higher elevation (where the partial pressure of CO2 is presumably lower) (McElwain, 2004). The negative relationship between CO2 concentration (or partial pressure) and stomatal frequency has often been used in palaeoecological investigations to reconstruct temporal changes of atmospheric CO2 concentrations using (sub)fossil leaf cuticles collected from sedimentary archives (Beerling, 1999; Beerling and Chaloner, 1993; McElwain and Chaloner, 1995; McElwain et al., 2002; Steinthorsdottir et al., 2013). However, such reconstructions are based on the assumption that environmental changes at smaller scales (i.e., among individuals or within individuals of the same population) are negligible compared to the stomatal-frequency changes caused by varying CO2 concentrations (Finsinger and Wagner-Cremer, 2009). Although estimates of the stomatal-frequency variation at intra-population and intra-individual level might be necessary to fully account for uncertainties in paleo-CO2 reconstructions, the analysis of modern leaves and of these sources of variation is rarely conducted (Chen et al., 2001).
Here we aimed to compare stomatal frequency within the Leonardoxa africana complex (Fabaceae–Caesalpinioideae) at different hierarchical levels: among sub-species, among populations belonging to the same sub-species, among individuals of the same population, and within individuals. We were particularly interested in investigating at which hierarchical level the greatest changes in the SI were observed and in illustrating the role of environmental variables as potential predictors for cuticle-morphology characteristics (stomatal frequency and stomatal pore size). Our investigation focuses principally on variations of SI because this variable allows a more appropriate comparison among leaves, since the SI is less biased by external factors than is stomatal density (Beerling, 1999).
2 Material and methods
Leonardoxa africana (Baill.) Aubréville (Fabaceae: Caesalpinioideae) is a species complex native to Atlantic central Africa (Fig. 1) that comprises four distinct, mostly allopatric subspecies (L. a. africana, L. a. letouzeyi, L. a. gracilicaulis, and L. a. rumpiensis). These have been described and identified based on morphological characters, particularly those related to the plants’ interactions with ants (McKey, 2000).

Location of the studied populations and contemporary distributions of Leonardoxa africana subspecies.
Emplacement des populations étudiées et distribution actuelle des sous-espèces de Leonardoxa africana.
Adapted from McKey, 2000.
These four taxa of small- to medium-sized understory trees are located in lower Guinea forests from extreme south-eastern Nigeria to Gabon (Fig. 1). Leonardoxa africana ssp. gracilicaulis McKey is a non-myrmecophyte involved in loose associations with opportunistic ants attracted to foliar nectaries that is most common on hills (McKey, 2000). Leonardoxa africana ssp. rumpiensis McKey has hollow internodes, inhabited by a variety of opportunistic ants (Chenuil and McKey, 1996; McKey, 2000). It appears to be very localized in submontane forests in the Rumpi Hills. Leonardoxa africana ssp. letouzeyi McKey is found in lowland forests in extreme western Cameroon and south-eastern Nigeria. Although its juvenile individuals can shelter several species of arboricolous ants in their internodes, mature trees of L. a. letouzeyi are associated specifically with a single ant species, Aphomomyrmex afer Emery (Gaume and McKey, 1998; McKey, 1991, 2000). Subspecies rumpiensis and letouzeyi may be considered as morphologically and ecologically ‘transitional’ myrmecophytes (Brouat and McKey, 2000; Fiala et al., 1999). The fourth sub-species of the complex, L. africana ssp. africana, is morphologically the most specialized myrmecophyte. It occurs in lowland coastal forests in southern Cameroon. Leonardoxa a. africana is associated, as early as the seedling stage, with one specific mutualist ant species, Petalomyrmex phylax Snelling (McKey, 1984, 2000). All four subspecies form pinnately compound leaves composed of two to four leaflet pairs.
2.1 Sampling design, leaf collection and cuticle analysis
Mature leaves of the four Leonardoxa subspecies were collected and dried at room temperature. All leaves were labelled with the subspecies name, the population, and the individual to which they belonged. We analysed two sets of leaves of Leonardoxa to investigate variation of cuticle-morphology characteristics at different hierarchical levels. The larger set comprised at least two leaves (sometimes three) for each individual growing in the sampled populations (Table 1). In the smaller set (hereafter named the ‘leaflet-comparison dataset’), all leaflets from one compound leaf were analysed for four individuals per species (Table 2). For the leaflet-comparison dataset (which included leaves of L. a. africana and L. a. gracilicaulis with three leaflet pairs), leaflet position within the leaves (proximal, middle, distal leaflet) was also noted.
Moyennes et écart-types des paramètres de morphologie cuticulaire mesurées sur des feuilles provenant de différentes populations des sous-espèces du complexe L. africana.
L. a. gracilicaulis | L. a. letouzeyi | L. a. africana | L. a. rumpiensis | ||||||
Population | Boundé (84 m) | Ebogo (669 m) | Mt Kala (931 m) | Iboti (1009 m) | Nguti (229 m) | Korup (351 m) | Boundé (84 m) | Ebodjé (86 m) | Dikome (1148 m) |
SD (# mm−2) | 243.3 ± 63.4 | 298.3 ± 108.3 | 239.6 ± 75.3 | 236 ± 61.7 | 233.9 ± 82.4 | 288.5 ± 102.4 | 229.5 ± 63.2 | 259.2 ± 72.4 | 354.4 ± 97.2 |
ED (# mm−2) | 3446.6 ± 437.6 | 3339.2 ± 404.0 | 3233.8 ± 483.2 | 3533.3 ± 471.4 | 3492.7 ± 696.0 | 3071.5 ± 445.4 | 3200.9 ± 392.3 | 3116.0 ± 423.8 | 3531.8 ± 455.6 |
SI (%) | 6.7 ± 1.9 | 8.3 ± 3.0 | 6.9 ± 2.2 | 6.3 ± 1.6 | 6.3 ± 1.9 | 8.6 ± 2.9 | 6.7 ± 1.8 | 7.7 ± 2.1 | 9.3 ± 3.1 |
PL (μm) | 13.5 ± 1.2 | 13.2 ± 1.2 | 14.3 ± 1.4 | 13.5 ± 1.3 | 13.6 ± 1.1 | 13.4 ± 1.3 | 13.2 ± 1.0 | 12.0 ± 1.2 | 13.8 ± 1.3 |
PW (μm) | 7.4 ± 0.1 | 7.7 ± 1.27 | 8.9 ± 1.2 | 7.3 ± 1.4 | 7.4 ± 0.9 | 8.3 ± 1.1 | 7.5 ± 1.0 | 6.7 ± 1.0 | 7.6 ± 1.0 |
# individuals | 10 | 10 | 10 | 8 | 10 | 10 | 10 | 10 | 8 |
# leaves sampled | 25 | 25 | 22 | 16 | 23 | 25 | 21 | 21 | 18 |
Moyennes et écart-types des paramètres de morphologie cuticulaire mesurées sur des feuilles provenant de différentes populations des sous-espèces du complexe L. africana.
L. a. africana | L. a. gracilicaulis | ||||||||
Population | Boundé (84 m) | Ebodjé (86 m) | Boundé (84 m) | ||||||
Leaflet position | Distal | Middle | Proximal | Distal | Middle | Proximal | Distal | Middle | Proximal |
SD (# mm−2) | 284 ± 47.7 | 312 ± 46.0 | 312 ± 22.8 | 293 ± 76.3 | 292 ± 55.4 | 285 ± 65.2 | 348.0 ± 71.8 | 272.0 ± 17.9 | 328.8 ± 86.2 |
ED (# mm−2) | 3064 ± 188.3 | 2864.0 ± 28.5 | 3140 ± 104.9 | 3224 ± 325.8 | 3173.3 ± 241.6 | 3570 ± 705.8 | 3421.6 ± 411.2 | 3520.0 ± 232.8 | 3564 ± 354.8 |
SI (%) | 8.49 ± 1.40 | 9.82 ± 1.38 | 9.04 ± 0.69 | 8.36 ± 2.08 | 8.49 ± 1.82 | 7.63 ± 2.14 | 9.25 ± 1.78 | 7.19 ± 0.63 | 8.42 ± 1.95 |
PL (μm) | 12.4 ± 1.0 | 11.9 ± 0.5 | 12.4 ± 0.8 | 12.7 ± 0.9 | 12.6 ± 0.8 | 12.6 ± 1.0 | 13.6 ± 1.3 | 13.0 ± 0.9 | 13.1 ± 1.2 |
PW (μm) | 6.6 ± 0.6 | 7.1 ± 0.7 | 6.8 ± 0.4 | 7.1 ± 0.8 | 6.9 ± 0.8 | 7.3 ± 0.7 | 7.7 ± 0.8 | 7.8 ± 0.8 | 7.5 ± 0.9 |
For cuticle analysis, a fragment of 0.5 cm2 was cut with a knife, bleached in a 5% solution of NaHClO2 at ∼60 °C for 5–6 h and then washed in distilled water. The bleaching caused the separation of the abaxial and adaxial leaf epidermis, allowing the stomata-bearing abaxial epidermis to be easily mounted on microscopic slides with glycerine jelly (Fig. 2). Cuticle analysis was performed using an Olympus BX 60 transmitted-light microscope (Hamburg, Germany) at ×500 enlargements connected to a Leica DFC 300 FX R2 video camera (Münster, Germany). The Leica Application Suite v3.7 image-analysis software allowed measurement, on count fields of 0.05 mm2, of stomatal density (SD) and epidermal-cell density (ED). Stomatal frequency (SI) was derived using the formula SI = (SD/[ED + SD] × 100) after Salisbury (1927). For each leaf, up to seven count fields were analysed and in addition 20 stomata were measured for pore length (PL) and pore width (PW), following Finsinger and Wagner-Cremer (2009). Two analysts performed the measurements of cuticle morphology, with the larger set being analysed by TDS (Dos Santos, 2011) and the smaller leaflet-comparison dataset analysed by WF.
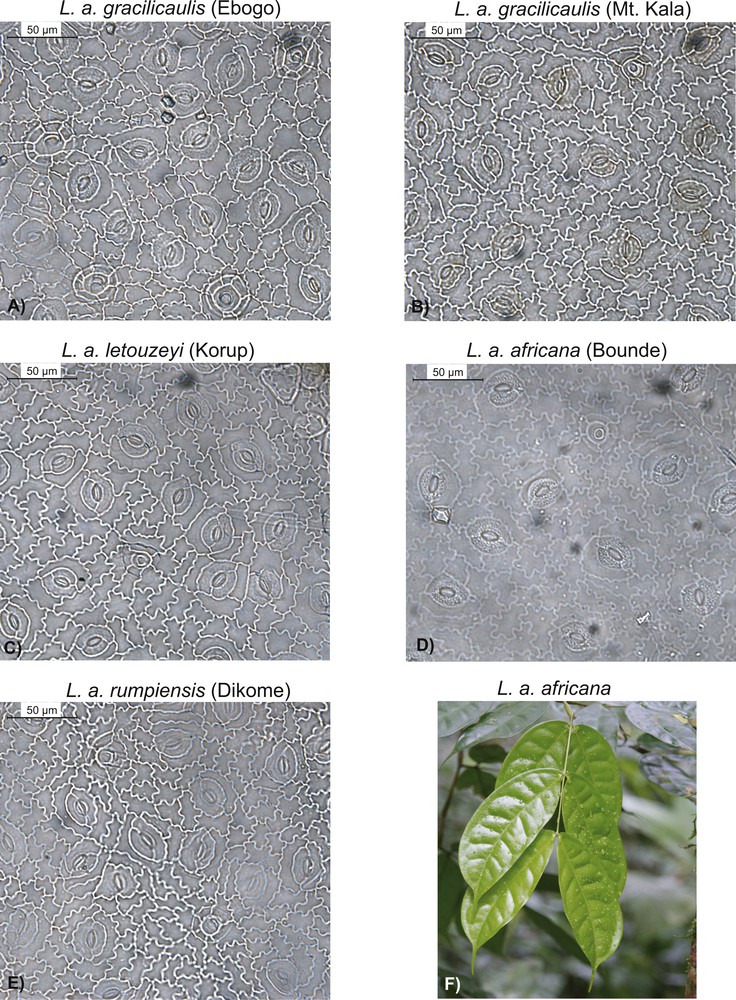
Images of leaf cuticles of the four subspecies of the Leonardoxa complex analysed in this study. A, B. Leonardoxa africana subsp. gracilicaulis from Ebogo (A) having higher SI and SD than leaf from Mt. Kala (B). C. Leonardoxa africana subsp. letouzeyi. D. Leonardoxa africana subsp. africana. E. Leonardoxa africana subsp. rumpiensis. F. Image of compound leaf of L. a. africana (color available online).
Image des cuticules de feuille des quatre sous-espèces de Leonardoxa analysées dans cette étude. A, B. Leonardoxa africana subsp. gracilicaulis provenant d’Ebogo (A) et de Mt. Kala (B). C. Leonardoxa africana subsp. letouzeyi. D. Leonardoxa africana subsp. africana. E. Leonardoxa africana subsp. rumpiensis. F. Image de feuille de L. a. africana.
2.2 Environmental variables
For each population, 20 environmental variables (Appendix S1) were extracted with the Diva-GIS software from the WorldClim database (Hijmans et al., 2005) that summarizes climate indices over a period of 50 years (AD 1950–2000). The dataset has a spatial resolution of 30 arc s (∼1 km) and allows obtaining accurate estimates of temperature and precipitation and derived climate indices (seasonality of temperature and precipitation, isothermality) in mountainous regions with large climatic gradients over small horizontal scales.
2.3 Numerical analyses
The dataset represents a hierarchical sampling wherein factors are nested within each category (populations in subspecies, individuals in populations, etc.). To investigate at which hierarchical level most of the variation in SI originates, we analysed the dataset using a variance-components analysis (Crawley, 2005, p. 638; Sims et al., 2006). We fitted to the L. africana SI dataset a linear mixed-effect model that included each of the categorical factors as a random effect plus a random error term (ɛ). The linear mixed model was fitted to these data using restricted maximum likelihood (REML) as implemented in the nlme v3.1–107 library (Pinheiro et al., 2013) in R v2.14 (R Core Development Team, 2012).
The relationships between climate variables are illustrated with a standardized principal component analysis (PCA; based on a correlation matrix with focusing on inter-subspecies correlations and standardizing subspecies scores by standard deviations, with centering and standardization by subspecies). This choice is particularly appropriate if the variables are measured in different units, e.g. when the data are actually environmental variables (Leps and Šmilauer, 2003). The relationship between stomata data (SI, PL and PW) and climate variables was illustrated with PCA (based on a covariance matrix with focusing on inter-subspecies correlations, subspecies scores not divided by standard deviation, centering by subspecies) and climate data added passively. All ordinations were calculated in Canoco 4.5 (Ter Braak and Šmilauer, 2003).
3 Results
Means and standard deviations of SD, ED, PL, and PW are listed in Tables 1 and 2 by subspecies, population, and leaflet position within compound leaves. In the nine populations of the four subspecies (Table 1), SD ranged between 229 and 354 stomata mm−2. All populations had means lower than 300 stomata mm−2 except that of L. a. rumpiensis at Dikome. Mean values of ED ranged between ∼3000 and ∼3500 cells mm−2 without notable extreme values. Similarly to mean SD, mean SI values were relatively homogeneous for most of the populations (with mean SI values ranging from 6 to 7%) but three populations had higher values: L. a. gracilicaulis at Ebogo (8.3%), L. a. africana at Ebodjé (7.7%), and L. a. rumpiensis at Dikome (9.3%). The variability of SI values, as estimated based on the standard deviations, accounts for 20–30% of the mean SI for all populations.
Mean values of S.D., ED, and SI measured on different leaflets of compound leaves from L. a. africana and L. a. gracilicaulis from Boundé (Table 2) differed from those listed in Table 1, likely indicating an observer effect. For SI, leaflet position was not a statistically significant factor for L. a. africana (ANOVA, p = 0.298), but was significant for L. a. gracilicaulis (ANOVA, p = 0.0489) (Fig. 3).

Boxplot illustrating the similarity between SI values in different leaflets (D: distal, M: median, P: proximal) of L. a. africana (LA from populations Boundé and Ebodjé) and L. a. gracilicaulis (LG from Boundé).
Boxplot illustrant la similarité entre les valeurs de SI sur différentes folioles (D : distal, M : médian, P : proximal) de L. a. africana (LA provenant des populations Boundé et Ebodjé) et L. a. gracilicaulis (LG provenant de Boundé).
SI and SD were strongly positively correlated in both datasets (r = 0.86–0.88; p < 0.001), indicating that variation in SI and SD was mainly due to differences in stomatal initiation rather than in the lateral expansion of epidermal cells.
The estimated variance components for SI were sometimes higher than the residual variance (Table 3). Variance among leaflets was always smaller than the intra-site (among-individual) variance (∼10% vs ∼40–50% of the variance, respectively), reflecting the marked difference of SI among individuals in comparison to the differences among leaflets of the same individual. Intra-site variance was mostly in the order of magnitude of residual variance except for L. a. gracilicaulis (51% vs 36% of the variance). Also in the full dataset (where among-leaflet variance was not taken into account), the intra-site variance was in most cases larger than variance at other hierarchical levels.
Pourcentage de variance expliquée par niveau hiérarchique pour l’indice stomatique (SI).
Inter-taxon | Inter-site | Intra-site | Intra-individual | Inter-leaflet | Residual % variance | |
Leaflet dataset | ||||||
All | 8.0 × 10−7 | 8.1 × 10−9 | 45.6 | / | 12.0 | 42.4 |
L. a. africana | / | 1.4 × 10−6 | 47.9 | / | 10.2 | 41.9 |
L. a. gracilicaulis | / | / | 51.1 | / | 12.9 | 36.0 |
Full dataset | ||||||
All | 6.2 × 10−6 | 12.1 | 40.6 | 18.9 | / | 28.3 |
L. a. letouzeyi | 30.2 | 41.5 | 7.4 | / | 20.9 | |
L. a. africana | 8.3 | 17.0 | 39.4 | / | 35.3 | |
L. a. gracilicaulis | / | 9.0 | 39.6 | 19.4 | / | 31.9 |
L. a. rumpiensis | / | / | 55.6 | 22.4 | / | 22.0 |
The first two Principal Component Analysis (PCA) axes of the climate data (Fig. 4a) for each of the nine populations explained ∼84% of the variance in the climate data (46 and 38%, respectively). The climate variables with the highest loading on the first PCA axis were altitude and mean annual temperature, whereas seasonality of temperature and precipitation, and isothermality had higher loadings on the second PCA axis.
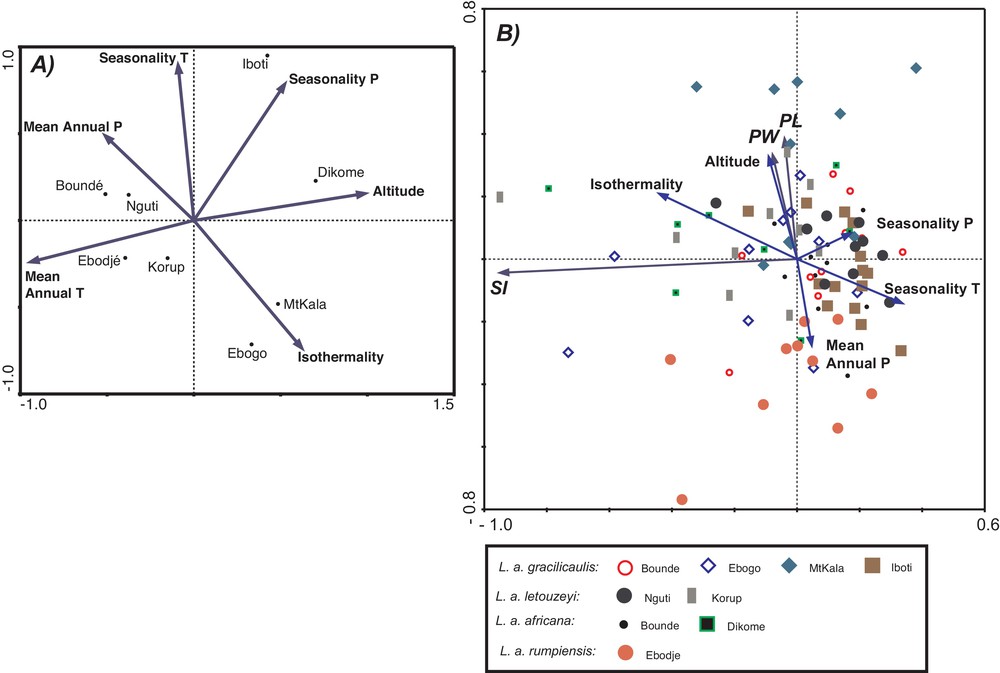
A. PCA illustrating main patterns of variation of climate indices at the sites where the nine studied populations of the L. africana complex occur. B. PCA illustrating the relationships between cuticle-morphology variables (SI, PL, and PW) in individuals of L. africana and climate indices (isothermality, altitude, seasonality of T and P, and mean annual P). Climate variables were added passively in this PCA (color available online).
A. ACP illustrant les principales tendances de variation des indices climatiques selon les sites où se trouvent les neuf populations étudiées du complexe L. africana. B. ACP illustrant les relations entre les variables de morphologie cuticulaire (SI, PL, PW) chez les individus de L. africana et les indices climatiques (isothermalité, altitude, saisonalité de la température et des précipitations, et précipitation annuelle moyenne). Les variables climatiques ont été additionnées passivement dans cet ACP.
The PCA of cuticle morphology parameters (Fig. 4b) placed SI with highest loading on the first axis (which explained ∼75% of the variance in the stomata data), whereas PL and PW were strongly correlated with each other and with the second PCA axis. Although the overall absence of distinct clusters of populations in the PCA biplot illustrates readily the high intra-population variability of stomatal morphology and frequency, some patterns seem to emerge. Populations having leaves with high SI values (Dikome [L. a. rumpiensis], Korup [L. a. letouzeyi], and Ebogo [L. a. gracilicaulis]) are mostly present towards the left part of the biplot. Also, whereas leaves from Ebodjé (characterised by low PL and PW values) are mostly located in the lower part of the biplot, those from Mt Kala are located in the upper part of the biplot. When added passively to the PCA with the stomata data, climate variables more strongly correlated with the first PCA axis are those related to the seasonality of temperature and precipitation, and isothermality.
4 Discussion
Regional environmental conditions only account for a relatively small part of the intra-taxon variability, as shown by higher variance of SI at the intra-site level than variance at higher and lower hierarchical levels (intra-individual, inter-taxon, and inter-site). Inter-site and intra-site variances of SI were smaller than intra-individual variance only for L. a. africana, of which the two populations studied are located at similar altitude and experience similar climatic conditions (Fig. 4a). This suggests that in most subspecies leaves within individuals were less different than leaves among individuals, and that increasing the number of replicate leaves per individual would have little impact on the precision of the variance-component estimates (Solomon, 2005). Inter-site variance was highest (although it did not exceed intra-site variance) for L. a. letouzeyi, whose two studied populations also experience similar climatic conditions (Fig. 4a) but are located at different altitudes, with leaves from the higher-altitude population having higher SI values (6.3 ± 1.9% vs 8.6 ± 2.9%).
Several herbarium studies have demonstrated SI-CO2 relationships in ferns, Gingkoaceae, conifers and angiosperms (Beerling et al., 1998; Kouwenberg et al., 2003; Wagner et al., 2007). However, stomatal-frequency datasets based on studies of herbarium specimens may invariably introduce significant variation into any SI-altitude or SI-CO2 relationship, owing to variation in the environmental conditions experienced by the sampled individuals, e.g., in habitats and locations of the individual trees sampled, or in light environments associated with different positions of leaves within the canopy (Kürschner, 1997; Smith et al., 2010; Woodward and Bazzaz, 1988).
Although the CO2 mixing ratio in air remains constant over altitudinal gradients, the CO2 partial pressure (
Part of the intra-site variability is certainly related to variation among leaflet positions. In fact, our analysis of leaflets from pinnately compound leaves of L. a. gracilicaulis indicated statistically significant differences in SI among leaflet positions (Fig. 2). Although we did not attempt to estimate the intra-leaf (or the intra-leaflet) variance with multiple readings on each leaf (leaflet) lamina, as Poole et al. (2000) did in their extensive study of Alnus glutinosa leaves, our results are consistent with the results of those studies in showing that intra-leaf variability can add significant noise to stomatal frequency-environment relationships.
On the other hand, stomatal frequencies can also be influenced at intra-site and intra-individual levels by other factors, including the timing of leaf maturation, developmental stage of leaves (Marchi et al., 2008), length of shoots, and position in the canopy (Chen et al., 2001). Although the sampling strategy adopted to collect the Leonardoxa leaves did not take into account most of these factors, it is likely that the high variance at intra-site level reflects phenotypic plasticity within each of the L. africana subspecies, presumably in response to highly local variation in micro-environmental conditions. In fact, L. africana trees often grow in the shaded understorey, although they sometimes also grow in less shaded riverside settings (McKey, 2000), and are therefore exposed to varying degrees of light intensity and microclimate conditions, which make them liable to experience different light regimes. It will be essential to estimate the influence of these micro-environmental factors if stomatal responses to environmental changes are further studied in this genus.
Inter-site variance seems to be related to regional environmental (climatic) conditions, as suggested by the PCA (Fig. 4). In L. a. gracilicaulis, individuals growing in a strongly seasonal climate (Iboti, Fig. 4a) were characterized by the lowest stomatal frequencies, whereas populations growing in the least seasonal and most isothermal climate (Ebogo) had the highest stomatal frequencies (Table 1). The high SI values in L. a. rumpiensis, the highest observed in this study, could also be related to environmental conditions, as this subspecies occurs at high altitude and under low seasonality, in a climate characteristic of tropical cloud forests. Long leaf lifespan has been proposed as a factor that may reduce the selective pressure to regulate stomatal initiation (and thus stomatal density and index) (Haworth et al., 2011) based on conditions experienced during the leaf-formation season to optimise gas exchange in response to major inter-seasonal and inter-annual environmental changes such as variation in water availability. In comparison, plants with shorter leaf lifespans (such as deciduous species) may be better adapted to optimise for the conditions of the growing season and for year-to-year fluctuations of environmental variables (Miller-Rushing et al., 2009; Wagner et al., 1996). Because Leonardoxa trees possess leaves with a relatively long lifespan (>3 years) (McKey, 1984), our results may suggest that Leonardoxa tend to regulate their stomatal frequencies with respect to the least favourable climate conditions at the growing site (hydric stress, temperature). In addition to highlighting the extent of plastic responses, our study also shows that the two different subspecies growing at the same site (L. a. gracilicaulis and L. a. africana at Boundé), exhibit similar stomatal frequency values, although they are genetically well separated (Léotard et al., 2008). This may reflect a high degree of adaptation of the two subspecies to the environmental conditions at Boundé.
5 Conclusions
Our analysis of stomatal parameters at different hierarchical levels in four Leonardoxa subspecies focussed on the SI to investigate its variation among subspecies, among populations of the same subspecies, among individuals of the same population and among leaves of the same individual. Only in few cases did we detect clear relationships between stomatal frequency and altitude that could reflect the well-known negative SI–CO2 relationship. Although variance of SI at the intra-site level always exceeded the variance at the inter-site level, perhaps owing to highly variable micro-environmental conditions within sites, we could highlight the potential influence of environmental factors (e.g. seasonality of precipitation) on the SI at the inter-site level. The long life span of Leonardoxa leaves may be a key factor that causes these plants to optimise for year-to-year fluctuations of the environment with respect to the least favourable climate conditions at the growing site (hydric stress, temperature). What emerges, therefore, is that in modern Leonardoxa trees the stomatal frequency could reflect great phenotypic plasticity presumably in response to local variation in micro-environmental conditions (e.g., position in the canopy, exposure).
Acknowledgements
We thank the Ministry of Scientific Research and Innovation of the Republic of Cameroon for permission to carry out this study. We thank Xavier Garde and the IRD in Yaoundé for providing logistic help in the field, and the residents of the villages near our study sites for their hospitality. We also thank Geo Coppens (CEFE) for help with using the WorldClim database. This study was funded by two grants from the French Agence Nationale de la Recherche to DM: one from the “Biodiversity” programme (IFORA project) and one from the “Sixth Extinction” programme (C3A project). We are grateful to Giovanna Battipaglia for comments on an earlier version of this manuscript and to two reviewers, Margret Steinthorsdottir and Roberto Tognetti, for thoughtful and constructive comments.