1 Introduction
Eurasia is commonly considered as a single rigid plate in paleo-reconstructions and computations of plate circuits allowing the computation of Euler rotation parameters between various components of the global plate system. However, a by-now large amount of Cenozoic paleomagnetic measurements from widely distributed localities over Asia generally report well-documented and ascertained primary magnetizations that exhibit anomalous paleomagnetic inclinations. In effect, they are often significantly shallower than those expected from the reference Meso-Cenozoic parts of the Apparent Polar Wandering Path (APWP) for Eurasia (e.g., Besse and Courtillot, 2002; Torsvik et al., 2008). Importantly, these synthetic reference APWP's rely on the hypothesis that Eurasia, from its western end in Europe to its eastern end in Siberia and East Asia, was a single, rigid plate.
The reference APWP of a given plate is built by first computing a synthetic APWP on a fixed continent after transferring worldwide paleomagnetic data onto it, using plate circuits as defined by ocean floor isochrons and resultant Euler rotation parameters (e.g., Müller et al., 1997, 2008). This fixed continent is Africa for the APWP's of Besse and Courtillot (2002) and Torsvik et al. (2008). Then a synthetic APWP is computed for a given plate by re-transferring the calculated master APWP onto it. All this construction relies on the hard hypothesis of rigid lithospheric plates, as postulated by the Plate Tectonics paradigm. This allows assuming the consistency of rotation parameters between every pair of plates, and to check the overall consistency of the plate circuit over the whole Earth system.
In this global system, the huge Eurasia plate has always been considered as a single entity since at least the Mesozoic. This hypothesis is reasonably verified for the plate circuits between Atlantic-bordering continents, but such a consistency has never been checked on the northeastern edge of this system, between North America and Siberia through the Arctic Ocean. Therefore, the fact that measured Cenozoic paleomagnetic inclinations are systematically shallower than predicted ones for a widely distributed amount of sampled localities in Asia has led the community to identify the so-called “Asian Tertiary inclination anomaly”.
Beyond discussions about age uncertainties of the studied formations, which could lead to such a situation (e.g., Cogné et al., 1999), this Asian Cenozoic inclination anomaly has received three interpretations:
- • a widespread effect of diagenesis and compaction having “flattened” paleomagnetic vectors recorded in sediments;
- • the persistence of non-dipolar Gauss coefficients, in particular the persistence of an axial octupolar component g30 in the mathematical expression of the geomagnetic field during some tens of millions of years;
- • a tectonic differential motion between the western and eastern parts of the Eurasian plate from the Mesozoic to the Present, still retaining the hypothesis of a dipolar magnetic field.
Based on a recent analysis of the Asian Meso-Cenozoic paleomagnetic database (Cogné et al., 2013), we examine each one of these three hypotheses and propose that a tectonic cause is actually the main contributor to this Cenozoic inclination anomaly.
2 The inclination anomaly
After a recent comprehensive review of the paleomagnetic database (Cogné et al., 2013), we propose a Cenozoic (e.g. 50–0 Ma) map of the paleolatitude anomaly over Asia. Indeed, the inferred paleolatitudes of rock formations are intimately linked to their recorded paleomagnetic inclinations. Based on 182 paleomagnetic studies of 50–0 Ma rock formations, we have computed the paleolatitude anomaly Δλ = λobs − λexp at each locality, as the difference between the observed paleolatitude (λobs) and the paleolatitude (λexp) expected from the reference European APWP of Besse and Courtillot (2002), under the hypothesis of a dipolar magnetic field (Fig. 1a). Negative values (hot colors on Fig. 1) occur at sites located further south than expected from the reference APWP, i.e. where observed paleomagnetic inclinations are shallower than predicted. Conversely, positive Δλ’s (cold colors) denote paleomagnetic inclinations that are steeper than predicted at the corresponding localities.

Maps of the 0–50 Ma Cenozoic paleolatitude anomaly in Asia computed as Δλ = λobs − λexp, where λobs are the actual paleolatitudes determined from paleomagnetic studies from 182 localities (see the review of Cogné et al., 2013), and λexp are the expected paleolatitudes from various reference magnetic field models at each locality; a negative Δλ reflects an anomalously shallow observed paleomagnetic inclination with respect to the predicted one; a: Δλ computed using the European Apparent Polar Wandering Path of Besse and Courtillot (2002; BC02) in the hypothesis of a dipolar magnetic field and a rigid Eurasian plate; b: Δλ computed using the BC02 European Apparent Polar Wandering Path acknowledging a maximum 15% of Cenozoic octupolar contribution to the magnetic field actually recorded by paleomagnetic data (see text); c: Δλ computed using the East Asian Apparent Polar Wandering Path of Cogné et al. (2013) in the hypothesis of a dipolar magnetic field and tectonic differential motions between Europe and East Asia.
From Fig. 1a, it is obvious that Cenozoic Δλ’s are largely negative over the Asian part of Eurasia, reaching very high values (∼−15°/−18°) in Tibet and central Asia, moreover exhibiting unconceivable values of ∼−8°/−10° over eastern Asia. The high values of the paleolatitude anomaly in Tibet and central Asia are most probably due to India collision and indentation into Eurasia (e.g., Chen et al., 1993; Cogné et al., 2013) and will not be further discussed here. Most importantly, however, the differences between observed and expected paleolatitude values in eastern Asia should be interpreted as the signature of a large relative north–south movement between eastern Asian regions and Eurasia since or during the Cenozoic, e.g. ∼1000 km of intracontinental shortening between East Asia and Siberia. This would be the natural interpretation following both hypotheses of a dipolar magnetic field and a rigid Eurasian continental plate. However, such Cenozoic to post-Cenozoic north–south relative movements between East Asian terranes and Siberia were never identified, neither in field studies nor in global tectonics models. Thus, this map (Fig. 1a), based on paleomagnetic results on the one hand, and on hypotheses both of a dipolar magnetic field model and of a rigid Eurasia plate on the other hand, must be considered as anomalous.
Three mechanisms have been advocated to explain this anomaly. These three mechanisms are discussed below.
3 Is the Cenozoic inclination anomaly in Asia due to diagenetic processes?
For Cenozoic and/or Mesozoic sediments in Asia, sedimentary processes such as compaction, and/or particle imbrication in river water flows have often been advocated to be responsible for the shallowing of paleomagnetic inclination with respect to the ambient field vector (e.g., Bazhenov and Mikolaichuk, 2002; Dupont-Nivet et al., 2002; Gilder et al., 1996, 2001, 2003, 2006; Tan et al., 2003, 2010; Tauxe and Kent, 2004; Yan et al., 2006, among others). As a matter of fact, a large number of paleomagnetic studies in central and eastern Asia are from sedimentary formations, mainly redbeds, where such processes may be suspected. We challenge this explanation. Based on our previous observations (Cogné et al., 1999) and our recent review of the Asian paleomagnetic database (Cogné et al., 2013), we stress the following points.
3.1 Latitudinal dependence of inclination shallowing in sediments
We advocated a first point several years ago (Cogné et al., 1999). Should a compaction induced inclination flattening mechanism in sediments follow a simple law, it would depend not only on the flattening amount in sediments, but also on the initial inclination of the magnetic field. Therefore, the anomaly should be related to the paleolatitudes at which sediments were formed. A very simple law of the form:
With a volume loss in sandstones of ∼0.2–0.4 (Baldwin and Butler, 1985; Lemée and Guéguen, 1996) and an a coefficient of 1.3 (Arason and Levi, 1990a,b), values of f = 0.3 to f = 0.5 were judged as reasonable bounds of a compaction induced ΔI in continental Meso-Cenozoic sediments from central Asia. The resulting curves of ΔI as a function of latitude in Asia (e.g., Cogné et al., 1999; Fig. 2a) are in disagreement with Cretaceous experimental values obtained in localities spread from Tibet to Central Asia localities (e.g., from south to north; points #1 to 8 and yellow qualitative patch on Fig. 2a). As a matter of fact, in the southernmost localities, ΔI is the largest where it should have been the smallest, whereas it is the contrary in the northern sites. Thus, our experimental data of Mesozoic sedimentary localities from Tibet to central Asia fail to demonstrate this latitudinal dependence of the inclination anomaly, at least in these examples.
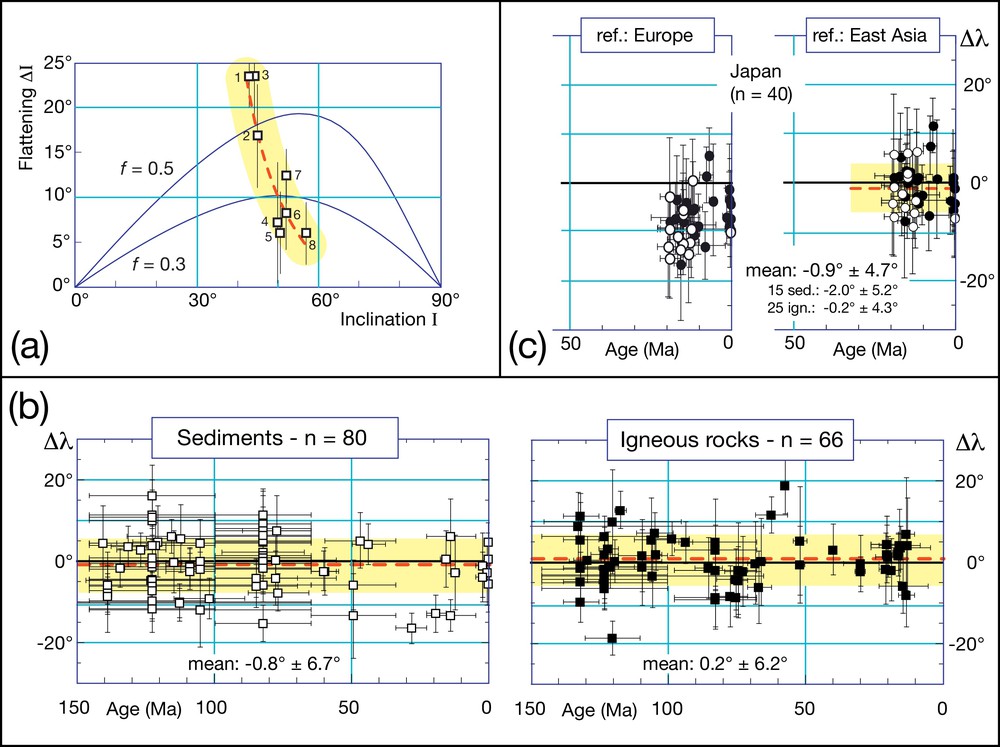
(Color online); a: inclination flattening (ΔI) as a function of initial inclination (I) in sediments; curves for flattening factors f = 0.3 and f = 0.5, after Cogné et al. (1999); square symbols with error bars are Cretaceous data from (1) South Lhasa, (2) North Lhasa, (3) Qiangtang, (4) Kunlun, (5) Tadzhik basin, (6) Xining–Lanzhou, (7) Tarim and (8) Junggar (in Cogné et al., 1999, after Halim et al., 1998); the yellow patch with a red dotted curve qualitatively underlines the discrepancy between experimental results and the modeled curves of inclination shallowing; b: plots of the paleolatitude differences Δλ, using the East Asia Apparent Polar Wandering Path of Cogné et al. (2013), as a function of geological age from the Present to 150 Ma, for sites located in eastern Asia (North and South China blocks, Korea peninsula, Amuria terrane and Siberia); a negative Δλ reflects an anomalously shallow paleomagnetic inclination at the locality; c: same as in (b) but for Japan dataset only; left plot: Δλ using the BC02 European Apparent Polar Wandering Path; right plot: using the East Asian Apparent Polar Wandering Path of Cogné et al. (2013); open (closed) symbols in (b) and (c): data from sedimentary (igneous) rocks.
3.2 Sediments vs. effusive rocks inclinations
A second line of evidence further arguing against sedimentary processes emerges from the recent analysis of the paleomagnetic database (Cogné et al., 2013). A significant number of paleomagnetic studies in Asia come from igneous rocks, such as basalts, in which no significant compaction or sedimentary effects on magnetic recording are usually suspected. The inclination anomaly also exists in these studies, no more but no less than in sedimentary localities of eastern Asia. In effect, the Cenozoic inclination anomaly has been obtained in basalts from Mongolia (Hankard et al., 2007). Furthermore, this anomaly is demonstrated in all stable blocks of eastern Asia, including the North and South China Blocks (NCB and SCB), Korea and Amuria terranes, and in the Siberian craton (e.g., see the review by Cogné et al., 2013), regardless of the igneous or sedimentary nature of sampled localities.
In order to check this observation, we have computed a new APWP solely based on paleomagnetic data from East Asia (e.g., North and South China blocks, Amuria terrane, Korean Peninsula, and Siberian Craton), still based on the dipolar field hypothesis. We have then re-computed the Δλ differences between measured and modeled paleolatitudes at each locality (Fig. 2b), using this new East Asia APWP (e.g., Cogné et al., 2013). The anomaly disappears in these eastern Asia terranes (Fig. 2b). The residual Δλ differences are statistically identical for data from sediments (−0.8° ± 6.7°, n = 80; Fig. 2b, left) and from igneous rocks (0.2° ± 6.2°, n = 66; Fig. 2b, right). Moreover, these values are not significantly different from zero.
This observation also applies to data from the Japanese islands only, which were not included in the computation of the East Asia APWP. In effect, both sedimentary and effusive paleomagnetic results from Japan demonstrate an “inclination anomaly” since 20 Myr (Fig. 2c, left). We notice that the anomaly is of the same order of magnitude for sedimentary and igneous rock formations. This is again confirmed when computing the residual Δλ anomaly using the new East Asia APWP (Fig. 2c, right): the residual discrepancy between predicted and observed paleolatitudes averages −2.0° ± 5.2° for sediments and −0.2° ± 4.3° for igneous rocks. Here again, these values are not statistically different either from each other or from zero. Therefore, this Japanese example also demonstrates that, within uncertainties, no statistically significant bias in magnetic field recording can be evidenced in sedimentary beds with respect to effusive rocks.
3.3 Inclination flattening in sediments is a minor phenomenon
In our analyses and compilations, these studies from East Asia show that residual Δλ values for sediments are always lower than for igneous rocks. This may be interpreted as the sign of a slightly shallower inclination recorded in sediments, in the order of ∼1–2° in terms of paleolatitudes. However, this is still well under the 2σ error limits of ∼5° on these statistics; thus it carries no robust statistical significance.
Altogether, this comparison of paleomagnetic results from sedimentary vs. igneous rocks, based on the analysis of the paleomagnetic database from Asia, leads us to conclude that sedimentary processes during diagenesis and/or deposition are not the first-order mechanism responsible for the widespread Cenozoic inclination anomaly in Asia (Fig. 1a), though in some peculiar cases, with some peculiar lithologies, such a mechanism can actually be advocated.
4 A tectonic cause or a non-dipolar geometry of the magnetic field?
Once the hypothesis of a diagenetic mechanism in sediments discarded, two explanations remain:
- • an anomaly in the global geomagnetic field, with the persistence of a significant non-dipolar component over several tens of millions of years;
- • a (as yet ill-understood) tectonic scheme of Eurasia plate deformation in the Cenozoic.
We now discuss these two hypotheses.
4.1 Non-dipolar geometry of the magnetic field
An anomaly of the Tertiary dipolar geomagnetic field in Asia was first proposed by Westphal (1993) from an analysis of Eocene paleomagnetic data from Iberia to Afghanistan. Based on information available at that time, the author proposed that a transitory dipole, inclined with respect to the Earth rotation axis, could have accounted for a ∼18° latitude anomaly in Eurasia during the Eocene. Shortly after, Chauvin et al. (1996) proposed a map of the Tertiary inclination anomaly over Asia with a common mechanism for all studied formations. Disputing the hypothesis of an inclined dipole, they proposed that the persistence of long-lasting non-dipolar magnetic field components could actually account for the overall Eurasian anomaly. In particular, they suggested that the contribution of an axial octupolar component G3 = g30/g10, as high as ∼10%–15%, could account for the ∼10°–14° anomaly of inclination at 35°N latitude. However, the authors pointed out that such high values of non-dipolar components should be considered as abnormal over several tens of millions of years. Si and Van der Voo (2001) pursued the same idea and, through different tests over Asian data, reached the same conclusion that a long-lasting G3 = g30/g10 contribution on the order of ∼10%–15% could explain a large part of the Cenozoic inclination anomaly. These authors, however, still did not provide explanations for such long-lasting persistence of a non-dipolar magnetic field component in the Cenozoic at the global scale.
We illustrate this hypothesis with the paleolatitude anomaly map of Fig. 1b. We have constructed this map using the reference European APWP of Besse and Courtillot (2002), and introducing a non-dipolar G3 = g30/g10 contribution in the computation of paleolatitude differences Δλ (Livermore et al., 1983; Merrill et al., 1998). Since the paleolatitude anomaly appears to have a maximum at ∼50–40 Ma, progressively diminishing through time (e.g., 20 Ma) then vanishing at ∼10 Ma (e.g., Hankard et al., 2007), we have modeled a 15% contribution of G3 for 30–50 Ma-old data, 7.5% for 10–30 Ma-old data, and 0% for 0–10 Ma-old data. The map on Fig. 1b suggests that a long-lasting octupolar contribution to the global magnetic field over the last 50 Myr can indeed account for the anomaly over all of eastern Asia. In effect, except for the Tibetan and Central Asian terranes, the East Asian regions are colored light yellow to light green, i.e. has a zero (± 5°) paleolatitude anomaly (Fig. 1b).
Although this non-dipolar model could account for the inclination anomaly, the idea of a long-lasting g30 term in the geomagnetic field is still far from having been proven on independent lines of evidence. In particular, we emphasize that Courtillot and Besse (2004) proposed that this octupolar term was, on the average, not in excess of a statistically insignificant value of 3 ± 8% of the dipolar field during the last 200 Myr. Still based on the dipolar hypothesis, we therefore consider that the European APWP is not the proper reference.
4.2 A new reference APWP for easternmost Eurasia
We propose to interpret the paleolatitude anomaly of eastern Asia as due to the inappropriateness of the European reference APWP in predicting the paleolatitude of the eastern end of the Eurasian plate in Cenozoic times. We have produced an independent APWP of eastern Asia from 130 Ma to the Present (Cogné et al., 2013; Fig. 3a), based on paleomagnetic results from the North and South China blocks, the Korean peninsula, Amuria terrane and Siberia (NCB, SCB, KOR, AMU and SIB, Fig. 3c), assumed to be the reasonably stable components of this highly mobile system.
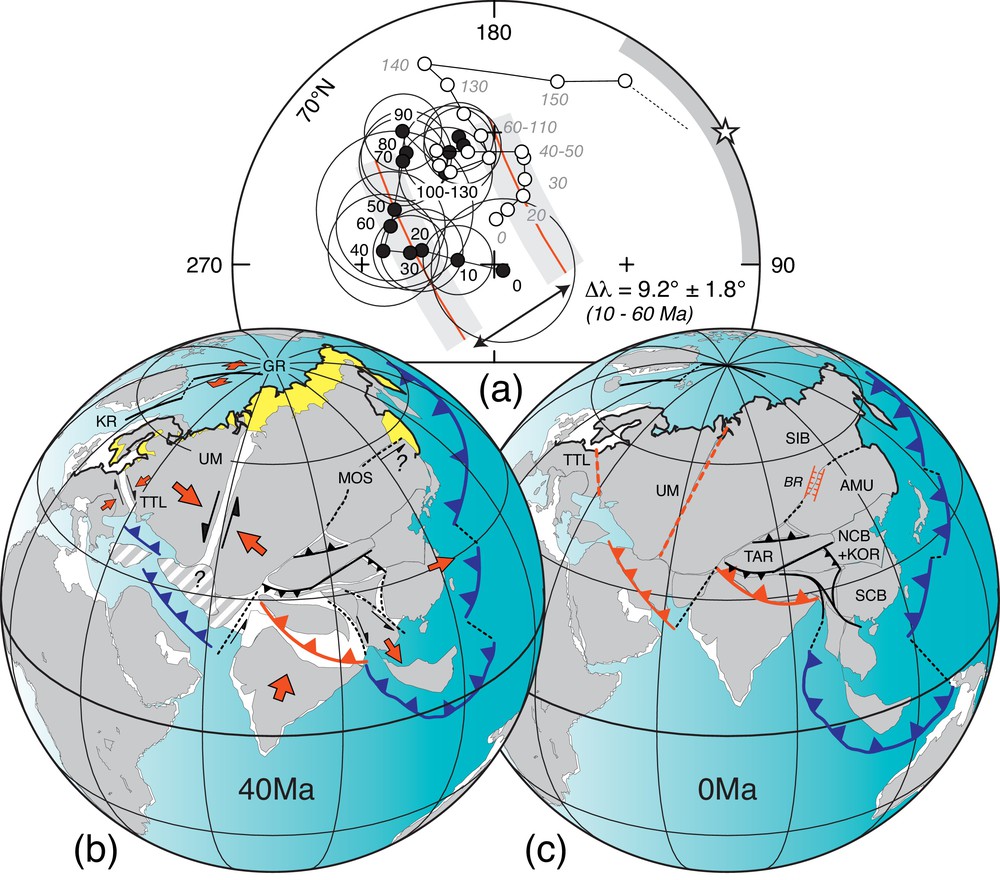
(Color online); a: equal-area projection, limited to the 70°N latitude, of the new East Asian Apparent Polar Wandering Path (black dots) of Cogné et al. (2013) compared to the Europe Apparent Polar Wandering Path (white dots) of Besse and Courtillot (2002); bold red lines with grey areas: small-circle fits over the 10–60 Ma poles of both paths, centered on a common reference point arbitrarily located within the eastern Asian terranes at {45°N, 120°E}; white star: longitude of this small-circles center; grey sector: spreading of sites longitudes used in the computation of the East Asian Apparent Polar Wandering Path; b: reconstruction at 40 Ma; c: present-day configuration; orthographic projections centered on {30°N, 75°E}; yellow area with a bold black outline in (b): paleoposition of Eurasia as predicted by the BC02 European Apparent Polar Wandering Path for a rigid Eurasian plate, to be compared to the grey contours and background of the East Eurasian paleoposition as predicted by the East Asian Apparent Polar Wandering Path; red and black arrows: expected and observed relative tectonic movements (see text); abbreviations in (b): GR: Gakkel ridge; KR: Knippovitch ridge (Arctic toponymy after Jakobsson et al., 2008); MOS: Mongol–Okhotsk suture; TTL: Tornquist–Tesseyre line; UM: Ural Mountains; abbreviations in (c): AMU: Amuria terrane; BR: Baykal rift; KOR: Korean peninsula; NCB: North China block; SCB: South China block; SIB: Siberia craton; TAR: Tarim block.
This APWP (closed symbols on Fig. 3a) is obviously different from the European APWP of Besse and Courtillot (2002; open symbols on Fig. 3a). More precisely, this difference resides in a far-sided location of eastern Asia Cenozoic paleopoles with respect to the poles of the European reference APWP, as seen from Asian site locations (grey sector on the right side of Fig. 3a). On the average, this difference amounts to 9.2° ± 1.8° for the 10 Ma–60 Ma period, quite similar to the ∼10° paleolatitude anomaly observed over eastern Asia in the map of Fig. 1a. As a consequence, computing the Δλ paleolatitude using the East Asian APWP as a reference, while still assuming a dipolar magnetic field hypothesis, resolves the paleolatitude anomaly of the eastern regions of Asia (Fig. 1c). In effect, as was the case for the map computed under the non-dipolar hypothesis (Fig. 1b), all are covered with light yellow/green colors (Fig. 1c), depicting a zero (± 5°) paleolatitude anomaly.
Large anomalies with hot colors (orange to red, Fig. 1c) remain in Tibet and central Asia, and cold colors (green to blue) over the Indochina peninsula, with a total magnitude between them of ca. ± 15° (Fig. 1c). These anomalies can confidently be attributed to:
- • the ongoing indentation of India into Asia, resulting in intracontinental shortening between Tibet, Central Asia and Siberia;
- • the southeastward extrusion of Indochina Peninsula.
This, however, is not the focus of the present paper and will no longer be discussed here.
Therefore, under the hypothesis of a dipolar geomagnetic field holding through geological times, a specific APWP for East Asia (Fig. 3a) can actually account for the main features of the Cenozoic paleolatitude anomaly over East Asia. Thus, we turn to the discussion of a potential tectonic decoupling between western and eastern Eurasia during at least the Cenozoic.
4.3 Tectonic hypothesis
Cenozoic differential motions between Europe and Siberia/East Asia would result in relative movements that would explain the differences between the reference APWPs of Fig. 3a. On Fig. 3 (b and c), we propose a scenario in which eastern Eurasia (e.g., Siberia and East Asia) follows the new East Asia APWP, locating it ∼10° further south than predicted from both the European APWP Cenozoic poles and the rigid Eurasia plate hypothesis. The bold contour with yellow background in these figures (Fig. 3b) underlines the discrepancy of Eurasian paleolatitudes predicted by the European APWP, to be compared to the paleolatitudes computed following the East Asian APWP (contours with grey background), assumed to be the stable parts of the Asian mobile system in the Cenozoic. Indeed, the inescapable consequence of this hypothesis is that Eurasia can no longer be considered to have been a single rigid plate since at least the beginning of the Cenozoic.
A non-rigid Eurasian plate was put forward by Cogné et al. (1999), but, at that time, no precise tectonic constraints were available as to the locus and ages of tectonic movements accounting for Cenozoic relative movements. In the frame of this hypothesis, relative north–south movements between Europe and Asia could have been concentrated along two discrete zones, the Tornquist–Tesseyre line (TTL) and the Ural Mountains (UM). However, field evidence of Cenozoic deformation along the Tornquist–Tesseyre zone or the Urals Mountains was quite scarce at that time. Since then, however, several studies have documented Cenozoic tectonics within both TTL and UM structures. They can be summarized as follows.
Kopp (1999, 2005, 2007) and Bergerat et al. (2007) identified an Eocene NE–SW shortening across the TTL, which, accounting for the relative azimuth of the TTL, results in dextral strike-slip faulting in this area. The movements we predict in this area (large red and thin black arrows over TTL on Fig. 3 b) are coherent with these field observations.
Geological and structural studies in the southern (Kopp, 2005; Verzhbitskii and Kopp, 2004, 2005) and northern (Kopp, 2007) Urals documented a Cenozoic east–west shortening between the central Kazakhstan block and the East European craton (Puchkov, 1997; Verzhbitskii and Kopp, 2005). Considering the Ural orientation, a sinistral strike-slip component is predicted by our model (e.g., large red arrows and black left-lateral shear arrows over UM on Fig. 3b), as actually described by geological field studies (Kopp, 2005, 2007; Puchkov, 1997).
Although the situation in central Asia is complicated by the Miocene Arabian collision in the south (e.g., Verzhbitskii and Kopp, 2004, hatched grey areas on Fig. 3b), the present-day topography of the Ural Mountains also suggests that it results from a Cenozoic east transpression with a left-lateral shear component.
A last point remains to be clarified: the amounts of continental shortening and respective lateral movements implied by this tectonic model. No precise evaluations, based on either field measurements or paleomagnetic estimates, are yet available. The inferred mid-Eocene to present-day shortening across the Ural is likely more important than across the Tornquist zone, as suggests the difference in relief. Our reconstructions (e.g., Fig. 3b) suggest that intracontinental displacements of a few tens of kilometers (up to ∼200 km?) in shortening and transcurrent movements are localized in those discrete zones, but it might be possible that diffuse continental deformations also exist over Eurasia, from East Europe to Siberia.
We emphasize that such moderate intracontinental movements could account for the large inclination or paleolatitude anomaly observed in the remote parts of eastern Eurasia, implying a more than 1000 km latitude discrepancy. This can be regarded as a huge lever-arm effect and one can understand why it has long remained undetected. Only more precise tectonic and paleomagnetic studies in the middle-eastern Eurasian regions, but also a careful check of the kinematic consistency between East Eurasia and North America in the Arctic, will help fully solving the plate circuit over these northern regions and produce the required amounts of intracontinental shortening across Eurasia.
5 Conclusions
The anomaly of Tertiary paleolatitudes (or paleomagnetic inclinations) over Asia has long been a puzzling problem for paleomagnetists as well as for tectonists, and overall for the whole community of Earth scientists in their understanding of plate tectonics of the largest continental plate on Earth, Eurasia. Based on the analysis of the whole Asian paleomagnetic database, and in line with previous studies and discussions, we acknowledge that three main causes explain the anomaly:
- • a shallowing of rock paleomagnetic records (namely paleofield inclinations) due to diagenetic processes;
- • the persistence of non-dipolar components in the magnetic field throughout the Cenozoic;
- • global (plate) tectonic movements between the eastern and western ends of Eurasia since the Mesozoic.
We favor the latter mechanism: the anomaly is due to a tectonic differential motion between Europe and Asia since the Cretaceous. This conclusion matches the assumed persistence of a dipolar geomagnetic field at the geological time scale during the Tertiary and recent field observations throughout central Europe, along the Tornquist–Tesseyre Line and the Ural mountains. Finally, we emphasize that our interpretation places Siberia ∼1000 km further south in the Cenozoic than previously expected from the reference European APWP in the hypothesis of a rigid Eurasia plate. Consequently, the amounts of intracontinental shortening across central Asia and the amounts of southeastward extrusion of Indochina peninsula under the effect of the India plate collision, and its ongoing penetration into Asia, should be reappraised.
Acknowledgements
This article has been kindly reviewed by J.-P. Burg and K. Schulmann. This is contribution 3451 of IPGP.