1 Introduction
Kimberlites, alkali basalts and lamprophyres are important conveyors of gems, especially for diamond and corundum from the asthenosphere and/or lower lithosphere to the upper crust (Giuliani et al., 2014; Shirey et al., 2013). These magmas carry xenoliths of mantle harzburgite, lherzolite and websterite, and in some localities eclogite, but also xenoliths of the lower crust such as felsic and mafic granulites. Diamond has never been found either as inherited crystals in alkali basalts or as inclusions in corundum. On the contrary, small sized crystals (< 3 mm) of ruby and sapphire have been described in xenoliths and diamond carried by kimberlites (Hutchinson et al., 2004).
In the diamond-bearing Mbuji-Mayi kimberlites from the Democratic Republic of Congo (DRC), centimetre-sized gem-quality ruby and pink sapphire megacrysts occur along with diamond and other minerals (Demaiffe et al., 1991; Pivin et al., 2011). In the sapphire-bearing Changle alkali basalts from the People's Republic of China (PRC), two to three centimetre-sized dark blue or yellow to brown gem-quality sapphires occur with diopside, anorthoclase, magnetite, zircon and garnet megacrysts (Guo et al., 1996; Song and Hu, 2009). Oxygen isotope compositions of corundum from alkali basalt have been extensively used to specify their geologic and geographic origins (Giuliani et al., 2014). Rubies originate from mafic rocks from the subcontinental lithosphere and sapphires have either magmatic (i.e. syenitic melt) or metamorphic (i.e. granulites) origin (Sutherland et al., 2009). In the present work, measurements of oxygen isotope ratios (18O/16O) in the lattice oxygen of ruby and/or sapphire, combined with trace-element analysis, from the Mbuyi Maji kimberlite and the basaltic gemfield in the Changle area provide clues to trace their origin in the deep Earth. The presence of megacrysts of corundum in kimberlite opens the debate about their origin. The unique way to answer this question is to compare their mineralogic and isotopic features with those of the corundums conveyed by alkali basalts. The comparison of these data together with new oxygen isotope ratios on corundum hosted by mafic rocks worldwide permits to propose a genetic scenario for the kimberlitic and basaltic eruptions from the lithospheric mantle up to the surface, and to give a clue for the origin of the gems.
2 Geological setting
The Mbuji-Mayi diamond-bearing kimberlites located in the East Kasai province (Demaiffe et al., 1991) intruded at the Upper Cretaceous the Archean Congo–Kasai craton (Fig. 1a). Two groups of pipes—a northern one and a southern one–crosscut the 2.8–2.4-Ga Archean Dibaya migmatitic complex, the 1.3–0.95-Ga Middle to Late Proterozoic sedimentary Mbuji-Mayi Supergroup, and the Lower Cretaceous Lualaba sedimentary series. The kimberlites contain megacrysts of garnet and diopside (Pivin et al., 2009), zircon and baddeleyite, and also Mg-rich ilmenite, Nb- or Cr-rich rutile, corundum, kyanite, Mg-rich chlorite, and rutile-silicate intergrowths (Pivin, 2012). The 0.5- to 1-cm-sized corundum megacrysts are either red and deep pink gem rubies, or cloudy pinkish to greyish sapphires (Fig. 1b and c). The rubies contain inclusions of Cr-bearing spinel (with Cr2O3 up to 1.5 wt%), chromite and rutile. The Cr-bearing sapphires host Mn-rich ilmenite, margarite and rutile inclusions. These different mineral species do not belong to a single paragenesis, but they are included in the kimberlite magma or are the fragments of xenoliths. The nodules are mostly eclogite, and rare peridotite and pyroxenite, or exceptional Cr-rich kyanite-bearing clinopyroxenite xenoliths (Pivin et al., 2011). These nodules do not contain corundum. Mbuji-Mayi kimberlites have Sr and Nd isotope signatures that are typical of Group-1 kimberlites (Weis and Demaiffe, 1985). The chemical composition and REE-patterns of garnet and clinopyroxene indicate that these minerals are the metasomatic product of mantle peridotites affected by fluid-bearing kimberlite (Pivin et al., 2009). Recent Hf–Nd systematics on garnet and clinopyroxene, zircon and baddeleyite suggested that garnet recrystallized from an “old” garnet-bearing peridotite during the interaction with the proto-kimberlite magma, while the three minerals crystallized during metasomatic interaction by the percolation of the proto-kimberlite fluid through these peridotites (Pivin, 2012).

(Color online.) The Mbuji-Mayi diamond kimberlites: (a): Central and South Africa diamond localities in relation to Archean cratons. Location of the Mbuyi-Mayi kimberlitic province (Democratic Republic of Congo) within the Congo–Kasai craton of Central Africa. Image of polished section of ruby (b) and pink to greyish sapphire (c) from the Mbuji-Mayi diamond kimberlite.
The Fangshan alkali basalts outcrop from Changle to Linqu (Fig. 2a) and cover about 1000 km2, adjacent to the Tan-Lu fault in the Shangdong peninsula, in eastern China (Guo et al., 1996). The volcanic rocks are Lower Miocene alkali basalt and tholeiite. The alkali basalts contain xenoliths of spinel lherzolite, wehrlite and pyroxenite. The sapphire megacrysts are located in the Fangshan Hill in four ∼ 1-m-thick horizontal layers of alkali basalt associated with peridotite xenoliths, and clinopyroxene, anorthoclase, magnetite, zircon, and garnet megacrysts (Song and Hu, 2009; Song et al., 2008). The sapphires are dark blue or yellow to brown (Fig. 2b) and most of them form hexagonal prisms broken along basal {0001} planes. The pinacoid section shows the hexagonal core and fine-oscillatory zone (Fig. 2c). Solid inclusions include zircon, columbite, oligoclase, carbonates and sulphates (Guo et al., 1996; Song and Hu, 2009). Microthermometry and Raman analysis on fluid/melt inclusions have characterized polyphase-fluid inclusions formed by H2O–CO2, Al2O3, Fe(Nb–Ta–Ti) oxides and minor carbonates and sulphates (Song et al., 2008). These inclusions homogenized at 1100 to 1300 °C.
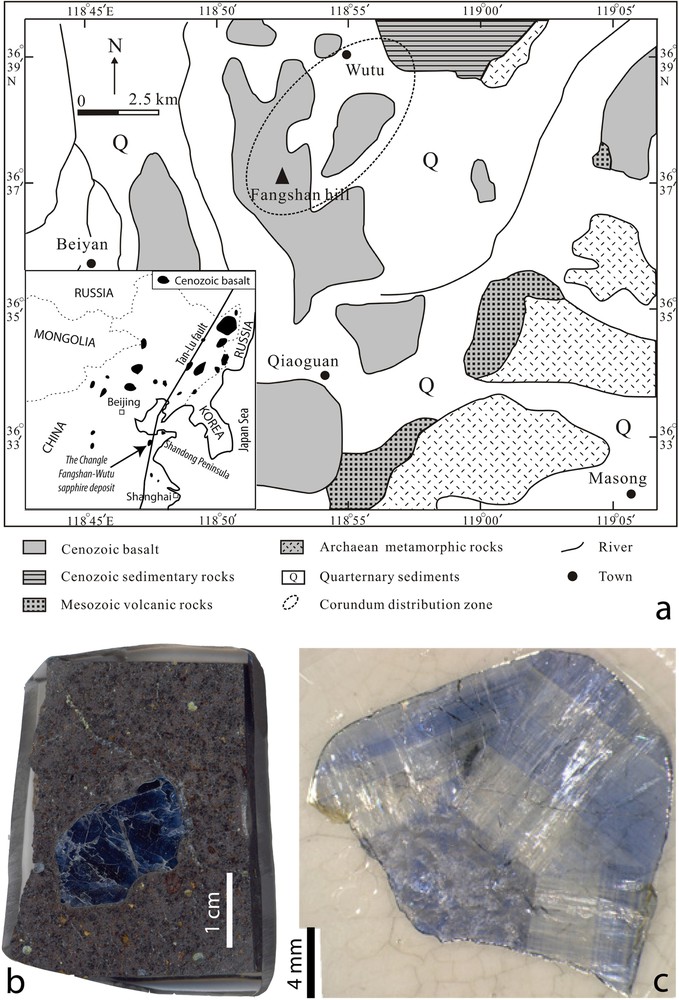
(Color online.) The Changle corundum alkali basalts in the People's Republic of China: a: map of distribution of the Changle-Linqu Caenozoic alkali basalts with the location of the sapphire-bearing Fangshan hill lava flows; b: image of sapphire megacryst included in the alkali basalt. (Photograph: F. Fontan); c: image of the pinacoid section of a sapphire with the hexagonal core showing fine-oscillatory zoning. (Photograph: G. Giuliani).
3 Samples and analytical techniques
Thirteen megacrysts of ruby and sapphire were collected from the Mbuji-Mayi kimberlite in the DRC, and nine megacrysts of blue and yellowish to brown sapphire from the Fangshan alkali basalt in the Changle province of PRC. In addition, six samples of ruby or pink sapphire associated with mafic rocks from France, Morocco and Madagascar were analysed in order to complete the worldwide chemical and oxygen isotope database on corundum and to discuss their origin in the deep crust.
The electron-probe microanalyses (EPMA) were obtained on a Cameca SX100 at the University of Lorraine with the operating conditions given in Rakotosamizanany et al. (2014).
Oxygen isotope analyses of corundum were performed at SUERC using a modification of the laser-fluorination technique described by Giuliani et al. (2005). Measurements of NBS 28 quartz standard gave a mean value of δ18O = 9.6 ± 0.1‰ (N = 6) over the course of this project, identical to the recommended value. Duplicate analyses of sapphire samples allow the estimation of the uncertainty for such material around 0.2 ‰ (1σ).
4 Results
4.1 EPMA analyses
The analyses on corundum megacrysts from Mbuji-Mayi and Changle are reported in Table 1. The Mbuji-Mayi rubies (N = 3) have very high contents in Cr2O3 (0.71–4.59 wt%) and relatively high FeO (0.22–0.66 wt%). Vanadium is relatively limited (986–1867 ppm) as well as Ti (< 13–68 ppm). The genetic indicator elements defined by Peucat et al. (2007), MgO (31–100 ppm) and Ga2O3 (50–193 ppm) range to moderate levels, with a low Ga/Mg ratio (0.6 to 2.6). The Fe/Cr ratio is below unity (0.12–0.45). The pinkish to greyish Cr-bearing sapphires (N = 10) are slightly richer in FeO (0.38–0.75 wt%) than rubies and they always contain some Cr2O3 (0.08–0.58 wt%). Vanadium is between 318 and 1128 ppm, and Ti is poorer in Cr-bearing sapphires than in rubies. The Ga/Mg ratio (1.95–3.95) is in the range of, or above, the Ga/Mg ratio of ruby. The Fe/Cr ratio is above unity (1.1–10.4).
Representative electron microprobe analyses of sapphire and ruby megacrysts from the Mbuji-Mayi kimberlite, and blue and yellow to brown sapphire megacrysts from the Changle alkali basalt.
Country | Ruby and sapphire megacrysts in the Mbuji-Mayi kimberlite - Democratic Republic of Congo | ||||||||||||
Sample | CO1 | CO2 | CO3 | COa1 | COa4 | COb1 | COb4 | COe1 | COe4 | COe5 | COe7 | COe8 | COfl1 |
Type | Ruby | Cr-bearing sapphire | |||||||||||
Colour | Flashy pink | Fuchsia | Red | Pinkish | Pinkish | Greyish to pinkish | Greyish to pinkish | Pinkish | Pinkish | Pinkish | Pinkish | Pinkish | Greyish to deep grey |
Number of analyses | 8 | 4 | 6 | 4 | 13 | 8 | 5 | 2 | 4 | 7 | 3 | 4 | 6 |
Al2O3 (wt%) | 99.39 ± 0.30 | 94.45 ± 0.48 | 97.30 ± 0.46 | 99.80 ± 0.15 | 99.00 ± 0.37 | 99.79 ± 0.29 | 99.79 ± 0.29 | 98.68 ± 0.10 | 98.46 ± 0 | 98.27 ± 0.37 | 98.69 ± 0.9 | 99.3 ± 0.4 | 99.27 ± 0.14 |
MgO (ppm) | 94 ± 52 | 105 ± 31 | 100 ± 28 | 100 ± 36 | 55 ± 37 | 81 ± 32 | 52 ± 50 | 55 ± 7 | 75 ± 19 | 51 ± 38 | 67 ± 32 | 60 ± 39 | 58 ± 40 |
TiO2 (ppm) | 45 ± 38 | 113 ± 36 | < dl | 110 ± 85 | 54 ± 36 | 64 ± 45 | < dl | < dl | < dl | < dl | < dl | 31 ± 19 | < dl |
V2O3 (ppm) | 273 ± 38 | 280 ± 53 | 140 ± 32 | 623 ± 46 | 477 ± 34 | 318 ± 56 | 518 ± 46 | 760 | 350 ± 42 | 746 ± 127 | 520 ± 106 | 471 ± 133 | 1128 ± 55 |
Cr2O3 (wt%) | 0.71 ± 0.28 | 4.59 ± 0.81 | 1.70 ± 0.09 | 0.08 ± 0.03 | 0.41 ± 0.08 | 0.08 ± 0.05 | 0.33 ± 0.03 | 0.44 ± 0.05 | 0.19 ± 0.1 | 0.58 ± 0.08 | 0.37 ± 0.1 | 0.28 ± 0.1 | 0.22 ± 0.08 |
FeO (wt%) | 0.22 ± 0.07 | 0.47 ± 0.03 | 0.66 ± 0.04 | 0.53 ± 0.01 | 0.71 ± 0.03 | 0.75 ± 0.03 | 0.76 ± 0.07 | 0.56 ± 0.04 | 0.49 | 0.72 ± 0.1 | 0.38 ± 0.1 | 0.57 ± 0 | 0.71 ± 0.04 |
Ga2O3 (ppm) | 193 ± 58 | 50 ± 29 | 75 ± 44 | 153 ± 26 | 151 ± 47 | 170 ± 33 | 110 ± 58 | 135 ± 21 | 148 ± 48 | 159 ± 39 | 117 ± 31 | 175 ± 30 | 163 ± 36 |
Total | 100.28 ± 0.32 | 99.56 ± 0.58 | 99.69 ± 0.39 | 100.50 ± 0.18 | 100.15 ± 0.37 | 100.68 ± 0.30 | 99.86 ± 0.29 | 99.78 ± 0.01 | 100.20 ± 0.4 | 99.63 ± 0.41 | 99.51 ± 0.9 | 100.2 ± 0.3 | 100.4 ± 0.20 |
Fe2O3a | 0.25 | 0.53 | 0.73 | 0.59 | 0.78 | 0.83 | 0.84 | 0.63 | 0.54 | 0.79 | 0.42 | 0.63 | 0.78 |
Fe/Cr | 0.35 | 0.12 | 0.43 | 7.38 | 1.90 | 10.38 | 2.55 | 1.43 | 2.84 | 1.36 | 1.14 | 2.25 | 3.55 |
Cr/V | 26 | 1.64 | 121 | 1.2 | 8.6 | 2.6 | 6.5 | 5.8 | 5.5 | 7.7 | 7.0 | 5.9 | 2 |
Ga/Mg | 2.6 | 0.6 | 0.95 | 1.95 | 3.5 | 2.65 | 2.7 | 3.1 | 2.5 | 3.95 | 2.2 | 3.7 | 3.6 |
Country | Sapphire megacrysts in the Changle Alkali Basalt - China | ||||||||||||
Sample | F3C1 | F3C2 | F3C6a | F3C6b | F3C16 | F3C17 | F3C18 | F3C32 | |||||
Colour | Deep blue | Colourless to yellowish | Blue | Deep blue | Light blue | Light blue | Yellow to brown | Brownish | |||||
Number of analyses | 11 | 12 | 20 | 18 | 12 | 13 | 20 | 18 | |||||
Al2O3 (wt%) | 98.90 ± 0.8 | 98.76 ± 0.50 | 99.38 ± 0.82 | 99.26 ± 0.44 | 98.44 ± 0.52 | 98.91 ± 0.36 | 99.44 ± 0.35 | 99.39 ± 0.45 | |||||
MgO (ppm) | 73 ± 65 | < dl | 90 ± 40 | 79 ± 35 | 75 ± 62 | 62 ± 51 | 47±34 | 40 ± 26 | |||||
TiO2 (ppm) | 255 ± 202 | < dl | 169 ± 92 | 154 ± 65 | 200 ± 95 | 238 ± 145 | < dl | < dl | |||||
V2O3 (ppm) | 145 ± 52 | < dl | 133 ± 33 | 136 ± 21 | 117 ± 39 | 123 ± 60 | 67 ± 32 | < dl | |||||
Cr2O3 (ppm) | 91 ± 30 | < dl | 72 ± 50 | 92 ± 48 | < dl | < dl | < dl | < dl | |||||
FeO (wt%) | 1.18 ± 0.07 | 1.40 ± 0.11 | 1.15 ± 0.09 | 1.18 ± 0.07 | 1.16 ± 0.04 | 1.16 ± 0.09 | 1.25 ± 0.11 | 1.42 ± 0.09 | |||||
Ga2O3 (ppm) | 400 ± 45 | 358 ± 52 | 404 ± 62 | 406 ± 63 | 367 ± 89 | 400 ± 58 | 417 ± 58 | 332 ± 48 | |||||
Total | 100.11 ± 1 | 100.21 ± 0.41 | 100.52 ± 0.84 | 100.52 ± 0.42 | 99.68 ± 0.53 | 100.15 ± 0.36 | 100.76 ± 0.36 | 0.41 ± 0.01 | |||||
Fe2O3a (wt%) | 1.30 | 1.55 | 1.28 | 1.31 | 1.29 | 1.29 | 1.39 | 1.58 | |||||
Fe (ppm) | 9161 | 10869 | 8928 | 9161 | 9005 | 9005 | 9704 | 11024 | |||||
Ga/Mg | 7.0 | – | 5.7 | 6.9 | 5.9 | 8.2 | 11.3 | 10.6 |
a Calculated from FeO content (wt%).
The blue (N = 5) and yellowish to brownish (N = 4) sapphires from Changle have high concentrations of FeO, respectively in the ranges 1.15 to 1.18 wt%, and 1.25 to 1.40 wt% (Table 1). Titanium (< 22–154 ppm) and V (< 22–145 ppm) contents are very low for both varieties. Chromium is found only in the blue sapphires and in very low concentrations (< 22–91 ppm). Contents of Ga2O3 are almost identical for all the sapphires (332–417 ppm) and the Ga/Mg ratio is between 5.7 and 11.3.
4.2 Oxygen isotope analyses
The oxygen isotope composition of thirteen corundum specimens from the Mbuji-Mayi kimberlite is in the range 4.3 to 5.6‰ (Table 2). Nevertheless, the Cr-bearing sapphires are distinct from the Cr-rich rubies. The latter have homogeneous δ18O values (5.5 to 5.6‰; N = 3). The pinkish to greyish sapphires have δ18O values in the range 4.3 to 5.4‰, with a mean of 4.9 ± 0.4% (N = 10). There is no correlation between the measured δ18O value and the Cr content of the corundum megacrysts, such as what has been observed for Cr-rich garnets from kimberlites (Wang et al., 2011).
Oxygen isotope compositions (in ‰, V-SMOW) of sapphire and ruby megacrysts from the Mbuji-Mayi kimberlite, and blue or yellow to brown sapphire megacrysts from the Changle alkali basalt. Ruby and sapphire hosted in mafic rocks from some other deposits worldwide such as Viala and Marvejols in France, Beni Bousera in Morocco, and Anavoha in Madagascar are also reported.
Country | Deposit | Sample | Nature | Colour | Type of deposit | δ18O | |
(‰, V-SMOW) | References | ||||||
RDC | Mbuji-Mayi | ||||||
CO1 | Ruby | Flashy pink | Megacryst | 5.5 | This work | ||
CO2 | – | Fuschia | In kimberlite | 5.5 | – | ||
CO3 | – | Red | – | 5.6 | – | ||
COa1 | Sapphire | Pinkish | – | 4.8 | – | ||
COa4 | – | Pinkish | – | 4.9 | – | ||
COb1 | – | Greyish to pinkish | – | 4.3 | – | ||
COb4 | – | Greyish to pinkish | – | 5.0 | – | ||
COe1 | – | Pinkish | – | 5.4 | – | ||
COe4 | – | Pinkish | – | 5.4 | – | ||
COe5 | – | Pinkish | – | 5.0 | – | ||
COe7 | – | Pinkish | – | 5.2 | – | ||
COe8 | – | Pinkish | – | 4.4 | – | ||
COf1 | – | Greyish to darkish | – | 5.0 | – | ||
China | Changle | ||||||
F3C1 | Sapphire | Brown | – | 2.8 | Hu et al. (2007) | ||
F3C7 | – | Brown | – | 4.5 | – | ||
F3C8 | – | Blue | – | 5.2 | – | ||
F3C10 | – | Blue | – | 5.65 | – | ||
CHA1 | Sapphire | Blue | Alkali basalt | 5.65 | Giuliani et al. (2009) | ||
F3C16 | Sapphire | Light blue | Alkali basalt | 5.2 | This work | ||
F3C17 | – | Light blue | – | 4.9 | – | ||
F3C1 | – | Deep blue | Alluvial | 5.0 | – | ||
F3C2 | – | Colourless to yellow | – | 5.2 | – | ||
F3C6a | – | Blue | – | 4.9 | – | ||
F3C6b | – | Blue | – | 4.9 | – | ||
F3C18a | – | Yellowish | – | 4.5 | – | ||
F3C18b | – | Yellow to grey | – | 4.85 | – | ||
F3C18c | – | Brown to grey | – | 4.6 | – | ||
Other deposits worldwide | |||||||
France | Viala | V1 | Ruby | Red | Amphibolite | 6.0 | This work |
V2 | – | Red | – | 6.2 | – | ||
Marvejols | M1 | – | Red | – | 6.7 | – | |
Morocco | Beni Bousera | BenB | – | Red | Pyroxenite | 4.4 | – |
Madagascar | Anavoha | Anv1 | – | Red | Amphibolite | 5.0 | – |
Anv2 | – | Red | – | 5.9 | – |
Measured δ18O values for Changle sapphires (Table 2) are in the range 4.5 to 5.65 ‰ with a mean δ18O = 4.9 ± 0.2‰ (N = 9). Previously measured oxygen isotope composition on the Changle sapphires gave δ18O values of respectively 5.65 ‰ (Giuliani et al., 2005), 5.2 and 5.65 for blue sapphire, and 2.8 and 4.5 ‰ for brown sapphire (Hu et al., 2007). The calculated mean δ18O value for all the Changle sapphires (excluding the 2.8 ‰ value) is 5.0 ± 0.4‰ (N = 13).
The measured δ18O values for other new ruby-bearing amphibolite occurrences worldwide are in the range from 4.4 to 6.7‰ (Table 2). The Viala and Marvejols ruby crystals from the French Massif Central are between 6.0 and 6.7 ‰ (N = 3), and the Anavoha rubies from Madagascar have δ18O values between 5 and 5.9 ‰ (N = 2). A ruby-bearing pyroxenite from Beni Bousera in Morocco has a δ18O value of 4.4 ‰.
5 Discussion
5.1 Trace-elements in ruby and sapphire: petrogenetic significance
The trace-element data for Fe, Ti, Cr, Ga, Mg are commonly used to discriminate the geological origin of gem corundum carried by continental alkali basalts (e.g., Peucat et al., 2007; Sutherland et al., 2009).
5.1.1 The rubies from Mbuji-Mayi
They plot in R3 mafics domain (Fig. 3a) defined in the FeO–Cr2O3–MgO–V2O3 vs. FeO + TiO2 + Ga2O3 classification diagram proposed by Giuliani et al. (2014).

(Color online.) Chemical variations of corundum megacrysts from the Mbuyi-Mayi kimberlite and the Changle alkali basalt. a: The FeO–Cr2O3–MgO-V2O3 versus FeO + TiO2 + Ga2O3 (in wt%) classification diagram (Giuliani et al., 2014). The different chemical fields of the studied corundums are reported: rubies (samples CO1, CO2, CO3) and pink sapphires (samples COa, b, e, f) from Mbuyi-Mayi, and for blue and yellow to brown sapphires from Changle; b: expanded view of a portion of the classification diagram with the report of the different samples of Muji-Mayi. Different fields are also reported for ruby from Beni Bousera (BB) in Morocco, and Soamiakatra (SOM), Anavoha (ANA), Vohitany (VOH), Beforona (BEF) and Andriba (AND) in Madagascar. r: ruby; w: white; b: blue sapphires from Juina kimberlite in Brazil.
(a) The ruby megacrysts have Cr > Fe. They form three separated fields in the R3 domain controlled by the Fe and Cr contents of ruby (Fig. 3b):
- • (a1) The field of sample CO1 overlaps the plots of rubies from Andriba in Madagascar hosted in ultramafics (Giuliani et al., 2014), the Winza deposit in Tanzania located in amphibolite and metagabbro (Schwarz et al., 2008), high-grade Proterozoic orthoamphibolites of the Bamble area in Norway (Hutchinson et al., 2004), and ultrahigh pressure ruby-rich garnetite layers from garnet lherzolite in the Sulu terrane of China (Zhang et al., 2004);
- • (a2) The field of sample CO2 is correlated to the very high Cr2O3 content of ruby up to 4.6 wt% (Fig. 3b). Cr-rich ruby is rare and found worldwide in the Brazilian São Luiz alluvial deposit and in the Juina kimberlite (Hutchinson et al., 2001, 2004; Watt et al., 1994), the Moses Rock Dike kimberlite in Utah (Padovani and Tracey, 1981), the metagabbros of Karelia in Russia (Bindeman and Serebryakov, 2011), and in the serpentinite at Hokitika in New Zealand (Grapes and Palmer, 1996);
- • (a3) The field of sample CO3 is comparable to the chemical composition of ruby-bearing garnetite xenolith in garnet peridotite from Sulu terrane in China (Zhang et al., 2004).
5.1.2 The Cr-bearing sapphires from Mbuji-Mayi
They plot in an ellipsoidal field with the main axis oriented NE–SW in R3 mafics and R4 metasomatic domains (Fig. 3b). They overlap the chemical field of the ruby and Cr-bearing sapphire crystals of the Soamiakatra deposit in Madagascar, hosted in xenoliths of metagabbro and clinopyroxenite brought-up to the surface by the Ankaratra alkali basalts (Rakotosamizanany et al., 2014). They overlap also the fields of:
- • ruby from the Chanthaburi-Trat and Pailin deposits hosted in alkali basalt fields from Thailand and Cambodia (Sutherland et al., 2009);
- • Cr-bearing sapphires and ruby micro-inclusions hosted by corganite (Mazzone and Haggerty, 1989) and corgaspinite xenoliths (Exley et al., 1983) from Jagersfontein and Bellsbank kimberlites in South Africa;
- • Cr-bearing sapphires found in kyanite eclogite xenoliths from the Roberts Victor mine kimberlite (Hatton, 1978).
The fields of samples COb1 and Coa1 from Mbuji-Mayi correlate with those of the rubies and Cr-bearing sapphires of Vohitany and Anavoha (Fig. 3b), hosted in amphibolite and meta-anorthosite from southern Madagascar (Rakotosamizanany et al., 2014).
The Ga/Mg vs. Fe diagram of Peucat et al. (2007) shows clearly the metamorphic origin for all the rubies and Cr-bearing sapphires (Fig. 4) from Mbuji-Mayi. Their domain overlaps the field defined for the rubies and Cr-bearing sapphires from the Malagasy deposits of Vohitany, Anavoha and Beforona.
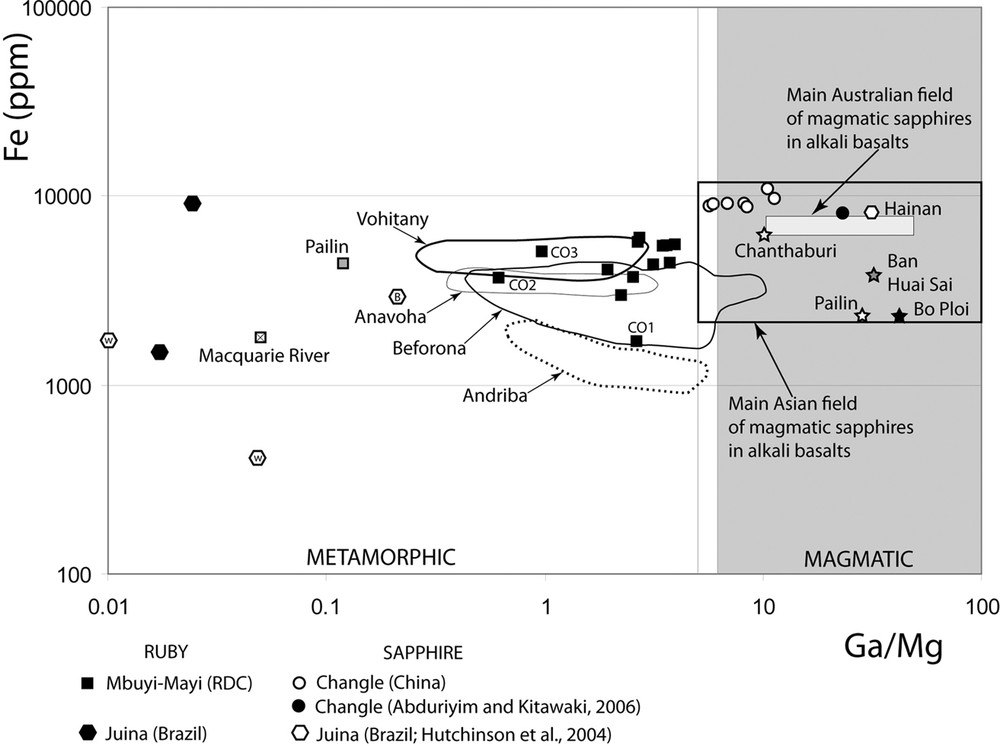
Fe (ppm) versus Ga/Mg discrimination diagram for corundums of magmatic and metamorphic origin (after Peucat et al., 2007). The electron-probe microanalyses (EPMA) analyses of crystals of corundum from Mbuji-Mayi and Changle are reported as well as LA-ICP-MS analyses of other rubies and sapphires from Australia, Thailand, Laos and Cambodia, with also the main Asian and Australian fields of BGY-sapphires (from Sutherland et al., 2009). The letters W and B in the hexagonal symbols from Juina corundum correspond respectively to white and blue colours.
5.1.3 The sapphires from Changle
The chemistries of the blue or yellow to brown sapphires are dominated by their Fe contents. Their chemical field forms a linear trend oriented NE–SW (Fig. 3a) characterized by an increase in FeO from blue to yellow to brown sapphires. The Changle field overlaps the domains of sapphires hosted in syenitic rocks (S1) and those found worldwide in alkali basalts and lamprophyres (S3). The Changle sapphires have Ga contents in the range of magmatic ones (Ga > 70 ppm). The Ga/Mg vs. Fe diagram shows clearly a magmatic origin for these sapphires (Fig. 4) as well as for blue sapphires from Bo Phloi and Chanthaburi in Thailand, Pailin in Cambodia, and Ban Huai Sai in Laos (Sutherland et al., 2009). All these blue–yellow–green sapphires plot within the Main Asian Field of magmatic sapphires in alkali basalts defined by Peucat et al. (2007).
5.2 Oxygen isotopes coupled with mineral inclusions and chemistry of gem corundum
Two O-isotope composition ranges are observed for the corundums from Mbuji-Mayi (Fig. 5), respectively between 5.5 and 5.6‰ for rubies (N = 3) and 4.3 and 5.4‰ (N = 10) for Cr-rich sapphires.

Oxygen isotope values of corundum megacrysts from Mbuji-Mayi kimberlite. The data reported in the conventional delta notation (in ‰) relative to Vienna Standard Mean Ocean Water (V-SMOW) are compared with the oxygen isotopic ranges defined for different rubies and pink sapphires deposits related to mafic rocks and alkali basalts worldwide (from Giuliani et al., 2014). The δ18O values of zircon, clinopyroxene and garnet megacrysts from Mbuji-Mayi kimberlites are from Pivin (2012).
5.2.1 The rubies of Mbuji-Mayi
They are remarkable for their size (up to 1 cm), gem-quality, colour varying from red to fuchsia and flashy pink, their high Cr2O3 contents up to 5.6 wt%, and identical oxygen isotope compositions. The mean δ18O value is 5.53 ± 0.06‰ (Fig. 5). These values demonstrate that the rubies have the same origin, with a clear mantle derivation and with no substantial variation (δ18O mantle of 5.5 ± 0.4‰; Mattey et al., 1994). They fit within the worldwide range defined for ruby in basaltic environments (1.3 < δ18O < 5.9 ‰, mean δ18O = 3.1 ± 1.1‰, N = 80) and ruby associated with mafics (0.25 < δ18O < 7.0 ‰, N = 19; Giuliani et al., 2014). The new oxygen isotope data obtained for ruby-bearing amphibolites from Viala, Marvejols and Anavoha (5.0 < δ18O < 6.7, N = 5; Table 2) also fall in the same range. The oxygen isotope compositions of ruby from Mbuji-Mayi are within the δ18O ranges of garnet (5.44 ± 0.34‰; N = 49) and clinopyroxene (5.63 ± 0.42‰; N = 41) megacrysts from the Mbuji-Mayi kimberlite (Pivin, 2012; Fig. 5). In contrast, they are distinct from the mean of zircon megacrysts (5.25 ± 0.22‰; N = 23), which are not in oxygen isotopic equilibrium with the garnet and clinopyroxene. The correction of the Cr-effect (Wang et al., 2011) on the average δ18O composition of Mbuji-Mayi garnet would give a value of 5.61 ± 0.35‰ (Pivin, 2012). The O-isotope composition of garnet, clinopyroxene and corundum are closely similar. Mineral fractionation are very consistent with Δ18Ocpx-gt, Δ18Ocpx-crd and Δ18Ogt-crd averaging 0.2, 0.1 and 0.1‰, respectively. They are also conxsistent with isotopic equilibrium at normal mantle temperatures with the mean δO18 values of peridotitic clinopyroxene (δO18 = 5.57 ± 0.32‰) and garnet (δO18 = 5.37 ± 0.36‰) commonly referenced as the mantle ranges (Mattey et al., 1994)
The inclusions, chemistry and oxygen isotope composition of the Cr-rich rubies from Mbuji-Mayi indicate that they probably formed in sub-lithospheric mantle ultramafics (Sobolev et al., 1968). The rubies indeed contain inclusions of Cr-bearing spinel, chromite and rutile. These Cr-enriched ruby megacrysts contrast with the micrometric (< 200 μm long) Cr-poor rubies described elsewhere in xenoliths from kimberlites.
The MORB-like oxygen isotope composition of the three ruby megacrysts from Mbuji-Mayi suggests different possibilities for the original protolith:
- • material originating from the upper mantle (garnet peridotite, garnetite, pyroxenite) in high pressure orogenic peridotite (Kornprobst et al., 1990). Such an origin was proposed for some of the eclogite xenoliths from the Bellsbank kimberlite (Neal et al., 1990). These group-A eclogites were considered mantle cumulates generated by crystal fractionation of garnet and clinopyroxene from a kimberlitic or proto-kimberlitic magma in the upper mantle;
- • in eclogite resulting from the subduction of the oceanic lithosphere and affected by the high pressure and temperature regime of the mantle, but with its oxygen isotope ratio buffered by the mantle δ18O through the eclogite phase transformation;
- • in corundum rich garnetite from a garnet peridotite linked to subduction-zone ultrahigh pressure metamorphism (P < 40 kbar and T > 800 °C) as found in the Sulu terrane (Zhang et al., 2004).
Nevertheless, the associations of corundum together with clinopyroxene and garnet (Sobolev et al., 1998) or Al-rich clinopyroxene have been identified in diamond (Hutchinson et al., 2001, 2004) as well as in corundum (Saminpanya and Sutherland, 2011; Sutherland et al., 2003; Sutthirat et al., 2001). At the Juina kimberlite deposit, high Cr2O3 ruby inclusions in diamond are associated with Al-rich pyroxene (up to 10 wt% Al2O3), ferropericlase and Mg-perovskite (Hutchinson et al., 2001). The Ga/Mg ratio of these rubies is less than 0.02 (Fig. 4) and is very different from that of the other rubies found worldwide in mafic rocks. The Ga/Mg ratios of the highly Cr2O3-rich rubies from the Mbuji-Mayi kimberlites are > 0.6 and fit within the values recorded from crustal mafic granulites or eclogite xenoliths carried up by alkali basalts (Fig. 4).
5.2.2 The Cr-bearing sapphires of Mbuji-Mayi
They have contrasted inclusions, chemistry and oxygen isotope composition compared to the rubies. They contain primary multi-oriented needles of rutile and Mn-rich ilmenite, while margarite is secondary. Such paragenesis is found either in eclogite xenoliths (Neal et al., 1990) or in kimberlite diatremes (Gréau et al., 2011). The chemical field of these sapphires overlaps the domain defined for metamorphic ruby and Cr-rich sapphire in mafic rocks and corundum in metasomatites (Fig. 3a). In addition, it overlaps:
- • the chemical fields of Cr-bearing corundum from Soamiakatra and Vohitany hosted in metagabbros and clinopyroxenites;
- • the domains of white and blue sapphire included in diamonds from Juina, part of those associated with the eclogitic xenoliths from Moses and Jagersfontein (Fig. 3b), and ruby from the Chanthaburi-Trat alkali basalt gemfield in Thailand.
The δ18O values of sapphires from Mbuji-Mayi range between 4.3 and 5.4‰ (mean δ18O = 4.95‰), which is lower than those of rubies; nevertheless, most of the values are within the accepted range of mantle values. These oxygen isotope values are within the range of those (Fig. 5) defined for ruby xenocrysts or megacrysts carried up to the surface by alkali basalts (Giuliani et al., 2014). They are also similar to those found for ruby in eclogites derived from oceanic crust (Shirey et al., 2013) and hosted by clinopyroxene xenocrysts from Bo Rai (Thailand) alkali basalt alluvials (Giuliani et al., 2005; Sutthirat et al., 2001; Fig. 5). Such petrographic and oxygen isotope compositions are also identical to those reported for garnet-clinopyroxene eclogites from Roberts Victor kimberlites in South Africa (Gréau et al., 2011). The lowest δ18O values of the Mbuji-Mayi Cr-bearing sapphires, between 4.3 and 4.9 ‰ (N = 4) can be generated either:
- • in subducted oceanic crust at relatively high-temperature, i.e. 300–400 °C similar to high-temperature seawater-altered oceanic basalts, the oceanic protolith being subducted to the pressure and temperature regime of the mantle while preserving its lower δ18O signature (Neal et al., 1990);
- • in mafic protoliths affected by metasomatic percolation of hydrothermal fluids;
- • by metasomatic reactions between pyroxenite or wehrlite intrusions in peridotites such as described for the Beni Bousera plagioclase-rich gabbros (Kornprobst et al., 1990) which contain ruby-bearing garnet pyroxenites (with δ18O value of ruby = 4.4 ‰; Table 2).
5.2.3 The sapphires of Changle
The blue or yellow sapphires have the same oxygen isotope range as the Cr-bearing sapphires of the Mbuji-Mayi kimberlite, but they show a contrasting chemical composition and mineralogy. The Fe versus Ga/Mg diagram reveals the high concentration of iron (up to 10,000 ppm) with a Ga/Mg ratio between 5.7 and 11.3 characteristic of magmatic sapphires, much higher in value than for the Cr-rich sapphires of Mbuji-Mayi (Fig. 6). Their oxygen isotope composition typically falls in the domain of sapphires from alkali basalt and/or syenite (Fig. 5). The δ18O values of the Changle sapphires (4.4 < δ18O < 5.7‰; mean δ18O = 5.0 ± 0.4‰, N = 13) are typical of gem sapphire in basaltic environments (2.7 < δ18O < 8.4‰, mean δ18O = 5.8 ± 1.2‰; n = 150; Giuliani et al., 2014; Fig. 6). The δ18O values of Changle sapphires overlap also the range for sapphires associated with syenite (4.4 < δ18O < 8.3‰, mean δ18O = 6.8 ± 1.4‰, N = 25; Giuliani et al., 2014), and with anorthoclasite xenoliths in alkali basalts, i.e. 4.4 < δ18O < 5.25 with a mean δ18O = 4.8 ± 0.3‰, N = 6 (Uher et al., 2012). The δ18O values of Changle sapphires (Fig. 5) are similar to, or slightly lower than, δ18O values of mantle-derived zircon megacrysts (4.7 < δ18O < 5.9 ‰; Valley, 2003) from kimberlites in Siberia and South Africa. These values suggest the magmatic origin of the sapphire xenocrysts from a subcontinental mantle reservoir without crustal contribution.

Oxygen isotope values of sapphire megacrysts from the Changle alkali basalt. The data reported in the conventional delta notation (in ‰) relative to Vienna Standard Mean Ocean Water (V-SMOW) are compared with the oxygen isotopic ranges defined for different BGY-sapphire deposits related to alkali basalts worldwide.
From Giuliani et al. (2014).
The association of sapphire, zircon, and anorthoclase megacrysts in the Changle alkali basalt is typical of assemblages crystallized from volatile- and incompatible element-rich felsic magmas (Graham et al., 2008; Upton et al., 1999). Moreover, the sapphires contain Nb-rich oxide minerals and CO2–H2O–Al2O3, Fe(Nb–Ta–Ti) oxides and minor carbonate- and sulphate-bearing fluid and melt inclusions (Song et al., 2008).
Corundum-bearing anorthoclasites are the main representative syenite xenoliths of the parental magma of sapphire in alkali basalts such as those in France, Scotland, Madagascar and Slovakia (Giuliani et al., 2014). The syenitic melt formed from a presumed mantle-derived alkali basalt magma (with a δ18O value between 5.4 and 5.8 ‰) would have an oxygen isotope composition between 6 to 7‰ (Yui et al., 2003). Sapphires crystallizing from such a melt at 1100 to 1300 °C would correspond to the homogenization temperatures of the melt inclusions from Changle sapphires (Song et al., 2008), and would have an oxygen isotope composition of 4.5 to 5.65‰ (Hu et al., 2007). These calculated values are in the range of the measured δ18O values of Changle sapphires and support its syenitic mantle oxygen isotope signature.
6 Conclusion and probable scenario for the gem conveyors
“Basaltic gem corundums” are generally upwardly transported by alkali basalt dykes and, rarely, by lamprophyres. Corundum-bearing alkaline magmas occur in crustal extensional environments impacted by the rise of upwelling mantle plumes (Fig. 7b) with a very low degree partial melting of the peridotite source. These high-temperature melts migrate rapidly during eruption and the gem corundum xenocrysts or megacrysts remain preserved without resorption except only for small etching on the crystals surface.
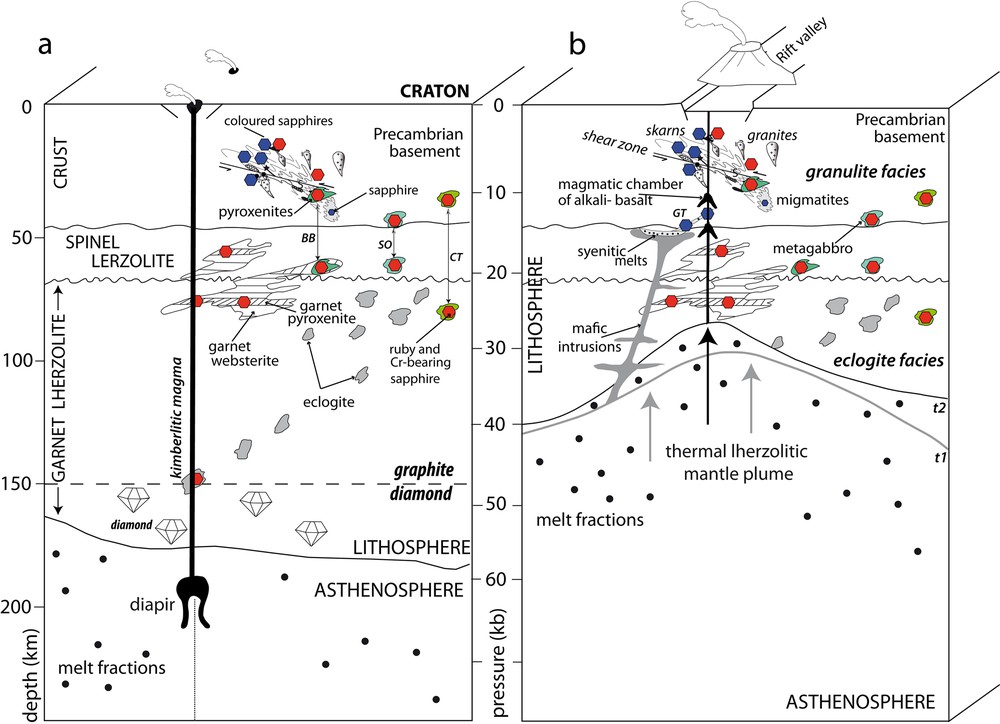
(Color online.) Schematic genetic model of gem corundum related to kimberlite (a) and alkali basalt (b) environments (see text for discussion). In the two schematic drawings are reported the different types of corundum occurrences hosted by the Precambrian basement in the crust, and by lherzolites in the lithosphere. Ruby occurrences in garnet clinopyroxenite of Beni Bousera (BB), garnet metagabbro and garnet clinopyroxenite of Somiakatra (SO) and pyroxene-bearing ruby of Chanthaburi-Trat (CT) are also reported, with their retrograde metamorphic location in the granulite facies. On Fig. 7b the syenitic dyke of Garba Tula (GT) in Kenya is reported. t1 and t2 are two successive stages of the alkali basalt magmatism.
Ruby and sapphire inclusions in diamond are rare and corundum xenocrysts/megacrysts in kimberlites are very scarce. The ruby and Cr-bearing sapphire crystals from Mbuji-Mayi kimberlite do not have the same origin as the Juina ruby inclusions in diamond. Their chemistry and oxygen isotope composition favour either:
- • an upper mantle-derived material (garnet lherzolite, garnetite, pyroxenite) that could have been infiltrated and metasomatized by the kimberlite melt, or a high pressure orogenic peridotite;
- • eclogite-type xenoliths (diopside-bearing metagabbro and/or clinopyroxenite) affected by a retrograde metamorphic stage in the granulite facies at the base of the continental crust (Fig. 7a).
The Cr-bearing sapphire could also have different probable origins: either as subducted oceanic crust transformed into eclogite, or as clinopyroxenite layers/horizons in peridotite.
The rubies and pink sapphires are of metamorphic origin. They are formed at the boundary between the continental lithosphere and the lower crust in mantle-derived mafic bodies (metagabbro, pyroxenite, wehrlite) as found in southern or in Morocco. Such ruby-bearing formations are affected by rising mantle plumes and are conveyed up to the Earth's surface by alkali basalts (Graham et al., 2008) and/or by kimberlite such as at Mbuyi-Mayi.
The homogenous oxygen isotope compositions of blue and coloured sapphires from Changle, combined with their chemistry and mineral inclusions, confirm that they have originally crystallized from evolved felsic magma derived from upper mantle melting. The sapphires are hosted in syenite derived from an igneous reservoir in the subcontinental spinel lherzolitic mantle (Fig. 7b). In the lithospheric mantle, felsic melts crystallized to form anorthoclasite, the most evolved peraluminous variant of the alkaline basaltic melt. The oxygen isotope compositions of the sapphires have a mantle signature with the same isotopic range as the Cr-bearing sapphires from Mbuji-Mayi, but in contrast, they have a magmatic origin. In this case study, the oxygen isotope tool is less discriminating than the mineralogy and chemical compositions of sapphires to decipher their geological origin. The Caenozoic alkali basalt dykes intruded these syenitic rocks and transported the megacrysts of sapphire to the surface.
Acknowledgements
The authors are very grateful for discussion and suggestions to Dr. Lin Sutherland from Geoscience Australian Museum, Sydney, Australia, the reviewers Dr. Philippe Boulvais, Géosciences Rennes, University of Rennes-1, France, and Dr. Brendan Laurs, geologist and editor of the Journal of Gemmology, Encinitas, USA, whose comments greatly improved the paper. Corundum megacrysts from Mbuji-Mayi kimberlites were generously provided by Carlos Fieremans and his son Mark Fieremans.