1 Introduction
Deep argillaceous formations are investigated as potential host rocks for High-Level radioactive Waste (HLW) disposals, of which the Callovo–Oxfordian (COx) argillite in France (Andra, 2005). These clayey rocks are mainly composed of clay minerals, carbonates, quartz, feldspar, pyrite, and may also contain small amounts of organic matter, depending on sedimentation and diagenesis conditions (Deniau et al., 2008). Most of this organic matter is intimately bound to clay minerals, constituting an insoluble macromolecular fraction called kerogen. Only a small amount of organic matter is present as dissolved organic matter (DOM) in pore water (Beaucaire et al., 2008; Courdouan et al., 2007a, 2007b; Vinsot et al., 2008).
The presence of bacteria in argillaceous media has been confirmed (Mauclaire et al., 2007; Poulain, 2006; Urios et al., 2012). Bacterial activity may be promoted by the presence of DOM used as an energetic substrate. Low-molecular-weight organic acids (LMWOA) are preferentially used by bacteria as electron donors that may enhance their development. In the context of HLW disposal, bacterial activity may have an impact on the material reactivity, especially on steel corrosion (Beech and Sunner, 2004; Dauzères et al., 2013; Esnault et al., 2010). For this purpose, bacterial impact on iron corrosion in clayey media is studied and bacterial survival has been recently confirmed (Chautard et al., 2012). Also, batch experiments performed in the presence of sulfate-reducing bacteria (SRB) have shown that hydrogen (energetic substrate for bacteria produced by anoxic corrosion of iron) plus DOM from argillite pore water may enhance the bacterial development, while hydrogen or DOM alone showed low or no effect (Chautard et al., 2013). Last, OM may exhibit complexation properties with radionuclides (RN) that might increase their mobility due to the formation of DOM-RN complexes (Maes et al., 2006) or retard their transport by formation of surface complexes with solid OM. Therefore, the nature of DOM should be carefully identified when investigating these issues as it can influence both the bacterial activity and the RN mobility.
The construction and operation of a geological disposal facility should induce physical and chemical perturbations (e.g., oxic transient, thermal transient and mechanical perturbations) that will affect locally the physical and chemical properties of the host rock and thus might impact the nature of DOM. Concerning the oxidizing perturbation induced by oxygen (air) diffusion during operation, a centimeters to meters-thick alteration profile is formed within the rock oxidized excavation damaged zone (EDZ) (De Craen et al., 2008; De Windt et al., 2014). In situ observations and laboratory experiments have evidenced that air oxidation of the kerogen present in argillite may influence the DOC concentration (Blanchart et al., 2012; Elie et al., 2004; Faure and Peiffert, 2007), suggesting a change in composition of the kerogen. Moreover, HLW is expected to produce a thermal flux for hundreds of years, with a temperature reaching a maximum of 90 °C in the host rock for the present French concept (Andra, 2005). Under temperature increase, kerogen can generate liquid and gaseous compounds whose amount and composition depend on the initial material. CO2 and soluble polar components containing oxygen (aliphatic and aromatic compounds) are produced from immature kerogen (Deniau et al., 2008). A weaker production of organic compounds is observed from kerogen with a higher degree of maturity. Finally, the pH of interstitial water in contact with metallic materials, which ranges from 9 to 11 (Bildstein et al., 2006), may influence the chemical nature and the concentration of leached organics.
Characterizing the OM is challenging, but essential to derive its reactivity (Derenne and Nguyen Tu, 2014). Furthermore, the low hydraulic conductivity and the low water content of deep clay rocks make the pore water sampling difficult without inducing important perturbations (Gaucher et al., 2006). For this reason, the method that was developed to assess the DOM (Courdouan et al., 2007a, 2007b) has also been used in this study (details about this method are briefly given in Section 2).
The French Radiation Protection and Nuclear Safety Institute (IRSN) conducts research programs on the Toarcian argillite in the Tournemire underground research laboratory (URL) (Aveyron, France). The Toarcian argillite has physical and chemical properties close to the COx argillite ones, except the level of maturity of organic matter (Deniau et al., 2008). The kerogen of the Toarcian argillite exhibits a pronounced level of maturity and has lost a large part of its oxygenated functions (Deniau et al., 2008). A DOC range of 15–45 mg·L−1 was measured in the Toarcian pore water from several boreholes (Beaucaire et al., 2008), this relatively low DOC being in good agreement with the relatively high level of maturity of the kerogen in the Toarcian Formation.
The present study focuses on the characterization of LMWOA that is dissolved in Toarcian pore water. It aims:
- • to isolate and to characterize DOC from the Toarcian argillite by performing batch extractions in the laboratory under anoxic conditions and compare with COx argillite and Opalinus clay;
- • to investigate the effects of short-term oxygen exposure, temperature and extractant pH.
2 Materials and methods
2.1 Rock samples
The core sample was horizontally air drilled in the Toarcian Clay Formation of the Tournemire URL (Aveyron, France) from the borehole BIO 01 in December 2011. They were vacuum-packed into double aluminium bags, and then flushed with nitrogen. All samples were stored under anoxic conditions. The outer part of each core was removed to avoid oxidation artifacts due to the air drilling and organic pollution (oil aerosols are always present in compressed air). The rock was crushed to a grain size smaller than 100 μm in a dry anoxic glove box (O2 < 1 ppm) and then permanently stored under anoxic conditions in the glove box. Note that the argillite was not purposefully dried, although it was ground in the dry glove box atmosphere.
The mean mineralogical composition of the Toarcian argillite is, by decreasing amount, silicate phases (86 wt.%, composed of 40% illite, 20% I/S, 30% kaolinite and 10% chlorite), carbonates (10 wt.%), pyrite (3 wt.%) and kerogen (OM) (< 1 wt.%) (Charpentier et al., 2001; Tinseau et al., 2006).
2.2 Batch extractions of water soluble organic matter
All batch experiments were conducted under anoxic conditions in a glove box (Jacomex, N2, O2 < 1 ppm). The extractions were performed with three replicates using crushed materials. Solid-to-liquid ratio of 500 g·L−1 and 1500 g·L−1 were tested (Courdouan et al., 2007a, 2007b). For the extractions, 30 mL of extractant solution were transferred into Teflon centrifugation tubes that contained 15 g or 45 g of crushed rock. The reference temperature was 60 °C. The samples were shaken for either 1 or 10 days using a shaking table, then centrifuged at 2500 g for 20 minutes and finally filtered through 0.45 μm PTFE filters. The experimental conditions are summarized in Table 1. At the end of the experiment, pH values were measured prior filtration.
Set of DOM extraction experiments with synthetic rock pore water, exploring different physical-chemical conditions representative of a HLW disposal cell.
Solid-to-liquid ratio | Series 1a Reference |
Series 1b Extraction time (10 days) |
Series 2 Alkaline pH |
Series 3 O2 exposure |
Series 4 25 °C |
500 g L−1 (12.5 g of crushed rock 25 mL of solution |
60 °C Anoxic SW 1 day pH 7 |
Series 1a but 10 days | Series 1a but SW pH 9 | Series 1a but short oxygen exposure (2 hours) | Series 1a but 25 °C |
1500 g L−1 (37.5 g of crushed rock 25 mL of solution) |
2.3 Toarcian synthetic water
The composition of the initial synthetic water (“SW pH 7”) used in the batch extractions is given in Table 2. Its composition is in good agreement with that of the Toarcian Formation at Tournemire (Beaucaire et al., 2008; Tremosa et al., 2012). The water was prepared by dissolving high purity salts in deionised water (Millipore, Integrale 5, 18 MΩ·cm−1) (Chautard et al., 2012) and then filtered on membrane (0.45 μm PTFE). The final pH was 7.4, which is consistent with the pH measured for Toarcian waters collected from boreholes. Before each extraction, the synthetic water was bubbled with high purity nitrogen gas during thirty minutes to eliminate oxygen.
Composition of the initial synthetic water (SW) pH 7, the initial synthetic water (SW) pH 9 as well as the anoxic 1-day extracted and the anoxic 10-days extracted for the solid-to-liquid ratio of 500 g·L−1.
Composition | Unit | SW pH 7 (initial) | SW pH 9 (initial) | Series 1a 1-day extract |
Series 1b 10-day extract |
pH | (−) | 7.4 | 9.0 | 7.9 | 8 |
Na+ | (mmol·L−1) | 22.5 | 36.5 | 26.5 | 25.6 |
K+ | (mmol·L−1) | 0.9 | 1.0 | 2.1 | 1.9 |
Ca2+ | (mmol·L−1) | 1.3 | 1.5 | 1.8 | 1.9 |
Mg2+ | (mmol·L−1) | 0.6 | 0.7 | 1.1 | 1.2 |
SO42– | (mmol·L−1) | 12.2 | 11.6 | 15.2 | 16.8 |
Cl– | (mmol·L−1) | 7.5 | 7.8 | 8.9 | 9.8 |
TIC | (mg·L−1) | 4.0 | 3.8 | 2.9 | 2.5 |
DOC | (mg·L−1) | – | – | 6.0 | 3.6 |
2.4 Tested parameters
The effects of solution pH, ambient air exposure, and temperature on DOC and LMWOA nature and concentration were investigated (Table 1).
pH effect: To investigate the effect of alkaline pH, a 0.1 mM NaOH solution was added to obtain a pH close to 9. This solution is called “synthetic water pH 9” throughout the paper (SW pH 9, Table 2). Similarly to the solution SW pH 7, before each extraction, the solution SW pH 9 was bubbled using nitrogen gas (high purity) during 30 minutes to eliminate oxygen.
O2exposure: To evaluate the effect of a short-term exposure of rock material to ambient air, the argillite was crushed in anoxic condition in the glove box, the ground material was exposed to atmospheric conditions and mixed during two hours (Courdouan et al., 2007a, 2007b) and then, stored again in anoxic conditions in the glove box.
Temperature effect: Concerning the temperature influence, two temperatures were tested: the reference temperature of 60 °C and a lower temperature of 25 °C.
2.5 Analyses of pore water and extracts
The total concentrations of LMWOA, anions (SO42−, Cl−) and cations (Na+, K+, Ca2+, Mg2+) were determined by ion chromatography (IC, 861 Advanced Compact IC, Metrohm, with 837 IC eluent degasser) using three different columns connected to a conductivity detector (injection volume 20 μL). The following LMWOA were analyzed (with a carbon number lower than 7): acetate, adipate, benzoate, butyrate, citrate, formate, fumarate, gluconate, glutamate, lactate, malate, malonate, oxalate and propionate. The column used to separate LMWOA was a Metrosep Organic Acids column (7.8 mm × 250 mm, 9 μm particle size, Metrohm). A solution containing 95 vol.% of H2SO4 (0.4 mM) and 5 vol.% of acetone served as mobile phase. The flow rate was set to 0.4 mL·min−1. A LiCl solution (70 mM) was used as MSM suppressor regenerant. Dissolved organic carbon (DOC) and total inorganic carbon (TIC) were determined in the pore water and in the extract solutions using a TOC analyzer (VARIOTOC, Elementar).
3 Results and discussion
3.1 Preliminary sensitivity tests
3.1.1 Influence of solid-to-liquid ratio
Table 3 gives the results obtained with the two solid-to-liquid ratios, 500 g·L−1 and 1500 g·L−1. DOC is either expressed in mg per liter of solution or normalized per kg of rock. This normalization allows discussing the solid-to-liquid ratio influence while concentration given in mg·L−1 represents the amount of organic matter available for microorganism development.
DOC and LMWOA total concentration extracted from Toarcian argillite using two different solid-to-liquid ratios (500, 1500 g L−1), two extraction times (1 day, 10 days), two rock crushing conditions (anoxic, oxic), two temperatures (25 °C, 60 °C) and two solution pH (7, 9). Each given value represents the mean (μ) of three replicates and is presented as μ (2σ), where σ is the unbiased standard deviation. % TOC represents the proportion of DOC versus the total organic carbon of the solid (argillite). % DOC represents the proportion of LMWOA versus the DOC of the solution.
Series 1a | Series 1b | Series 2 | Series 3 | Series 4 | |||||||
Concentration | unit | Reference (1 day) | Extraction time (10 days) | Alkaline pH (SW pH 9) | O2 exposure (2 h) | Lower temperature (25 °C) | |||||
500 g L−1 | 1500 g L−1 | 500 g L−1 | 1500 g L−1 | 500 g L−1 | 1500 g L−1 | 500 g L−1 | 1500 g L−1 | 500 g L−1 | 1500 g L−1 | ||
DOC | (mgC·L−1) | 6.0 (1.3) | 14.2 (0.6) | 3.6 (0.9) | 8.5 (1.1) | 19.3 (0.4) | 23.7 (0.3) | 9.3 (1.0) | 24.7 (0.4) | 1.9 (0.3) | 4.7 (0.4) |
DOC | (mgC·kg−1) | 12.1 (2.7) | 9.5 (0.4) | 7.2 (1,8) | 5.6 (0.8) | 38.6 (0.8) | 15.8 (0.2) | 18.6 (2) | 16.4 (0.3) | 3.8 (0.7) | 3.1 (0.3) |
% TOC | (–) | 0.12 | 0.09 | 0.07 | 0.06 | 0.39 | 0.16 | 0.19 | 0.16 | 0.04 | 0.03 |
LMWOA | (mgC·L−1) | 2.3 (0.2) | 4.1 (0.4) | 2.4 (0.2) | 4.5 (0.5) | 2.8 (0.1) | 4.4 (0.4) | 4.3 (0.3) | 10.5 (0.3) | 1.8 (0.1) | 3.4 (0.1) |
LMWOA | (mgC·kg−1) | 4.7 (0.4) | 2.8 (0.3) | 4.7 (0.3) | 3.0 (0.3) | 5.5 (0.2) | 3.0 (0.3) | 8.5 (0.5) | 7.0 (0.2) | 3.6 (0.2) | 2.2 (0.1) |
% DOC | (-) | 38.5 | 29.2 | 65.5 | 53.5 | 14.4 | 18.8 | 45.9 | 42.5 | 95.4 | 72.5 |
Regardless of the series (Table 3), as expected, the 500 g·L−1 ratio systematically yielded the lowest DOC concentrations (about 2.5 times less concentrated in solution than for 1500 g·L−1). Same findings have been reported for the COx argillite and the Opalinus Clay (Courdouan et al., 2007a, 2007b).
In all, the present experiments, the LMWOA concentration increased when the solid-to-liquid ratio increased, but the LMWOA proportion of DOC decreased, except for the series 2 (pH influence). Concentrations of the main LMWOA normalized per kg of rock are given in Fig. 1. Such results suggest that an additional organic matter contribution in solution, which was not detected by IC, occurs for lower liquid-to-solid ratio. It may correspond to the additional dissolution of complex organic macromolecules (humic and/or fulvic like compounds). LMWOA concentrations remained stable, except for fumarate whose concentration proportionally strongly increased at lower solid-to-liquid ratio. The strong decrease of fumarate observed at high solid-to-liquid ratio could also be explained by an increase of its sorption on the solid phase. Indeed, fumarate is the only bidentate ligand of the series (ethylene dicarboxylic acid: HOOC − CH = CH − COOH). One may also observe that fumarate has both the lowest pKa of the LMWOA series (3.03) and the highest relative concentration (mg·kg−1 rock) in Fig. 1a, while propionate with the highest pKa value (4.88) of the series exhibits the lowest relative concentration. The pKa value of the small organic acids might influence their relative concentrations as presented on Fig. 1a, but the effect is not clear for the intermediate pKa values and it might be a fortuitous coincidence. Fumarate could also be produced by bacterial attack of DOC under anaerobic conditions (Grossi et al., 2008; Mbadinga et al., 2011).
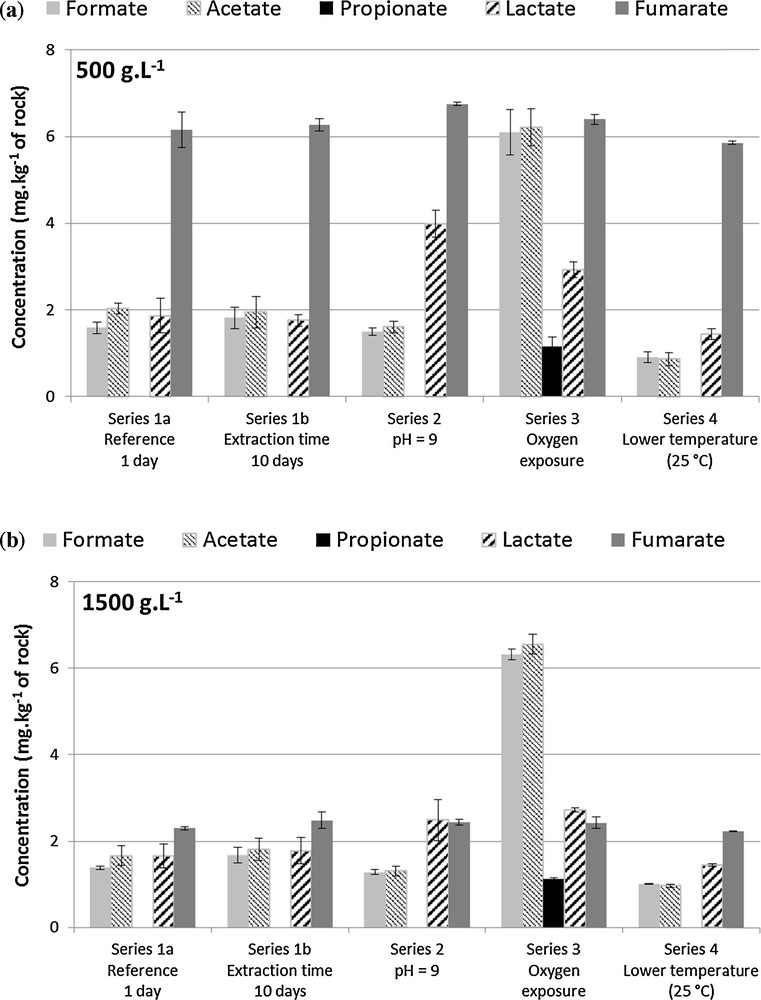
a and b: LMWOA (acetate, formate, fumarate, lactate and propionate) concentration (mean value of three replicates) in mg·kg−1 of crushed rock, extracted from Toarcian argillite at two different liquid-to-solid-ratios 500 g·L−1 (a) and 1500 g·L−1 (b). Propionate was identified in the series 1a, 1b, 2 and 3, but with a concentration below the quantification limit (0.5 mg·L−1). The error bars correspond to twice the unbiased standard deviation of three replicates. The reference temperature for extraction is 60 °C.
3.1.2 Influence of extraction time
Whatever the solid-to-liquid ratio, DOC concentrations in the 10-day extracts (series 1b) under anoxic conditions were systematically lowered by 40% compared to the 1-day extracts (Table 3). This has already been observed in previous studies (Courdouan et al., 2007a, 2007b) and seems to be due to a re-adsorption of organic compounds on clay minerals (Ramos and Huertas, 2014). On the contrary, acetate, formate, fumarate, lactate and propionate concentrations were not influenced by extraction time. Therefore, the difference in DOC concentrations might arise from re-adsorption of higher molecular weight organic compounds that were not identified by IC (Courdouan et al., 2007a, 2007b). Indeed, these compounds may be preferentially sorbed because of a probably lower solubility due to their heavier molecular mass compared to LMWOA.
Moreover, the ionic compositions of the 1-day and 10-day extracts are very close (Table 2). The slight increase in chloride and sulfate concentrations compared to the synthetic water may be due to additional contributions of the argillite pore water. This confirms that little perturbation occurred between sampling and experiments. Globally, all these results point out that a 1-day extraction is sufficient to extract DOM from Toarcian argillite and avoid re-adsorption phenomena. For this reason, 1-day duration was applied for all the other extraction tests.
3.2 Anoxic undisturbed conditions
Table 3 gives the results obtained with the two solid-to-liquid ratios under anoxic conditions (series 1a). The DOC is 6.0 and 14.2 mg·L−1 for the 500 and 1500 g·L−1 ratio, respectively. The total organic carbon (TOC) of the argillite is about 0.9 wt.% (Deniau et al., 2008). The very low proportions of DOC versus TOC (0.12 and 0.09%) indicates that only a low fraction of total organic carbon was extracted from the rock material by aqueous solution, as expected from the high maturity degree of the Toarcian argillite kerogen. The DOC also represents a very small fraction (0.06 to 0.14%) of the total organic carbon of the Callovo-Oxfordian clayey rock (Courdouan et al., 2007a). Batch DOC extracts were lower than DOC measured in situ at Tournemire, i.e. about 15–45 mg·L−1 (Beaucaire et al., 2008), and correspond to the lower boundary of the DOC range measured in the COx Formation, i.e. 9–50 mg·L−1 (Courdouan et al., 2007a; Vinsot et al., 2008). However, the comparison between laboratory and in situ measurements is not easy because of the huge difference of solid to liquid ratio.
The proportion of LMWOA with respect to the DOC (Table 3) is 38.5 and 29.2% for the 500 and 1500 g·L−1 ratio, respectively. This is close to the relative proportions found for the COx (21%, 1500 g·L−1) and Opalinus Clay (29%, 1500 g·L−1) formations (Courdouan et al., 2007a, 2007b). Acetate, formate, fumarate, lactate and propionate were identified under anoxic conditions (Fig. 1). Adipate, benzoate, butyrate, citrate, gluconate, glutamate, malonate and oxalate were below the detection limit (0.2 mg·L−1). The chemical formulae, molecular weights and functional groups of the identified LMWOA are reported in Table 4. The acids are present as their conjugated bases (e.g., acetate instead of acetic acid) in the pH range of the extraction solutions. The normalized concentration of fumarate was the highest (2.5–6.0 mg·kg−1), the concentration of propionate the lowest (< 0.5 mg·kg−1). Acetate, formate and lactate were at a concentration of 2 mg·kg−1 approximately. The LMWOA extracts of the Callovo-Oxfordian showed a similar distribution (Courdouan et al., 2007a), except for fumarate. Acetate, formate and lactate were also the three major species with equivalent concentrations whereas propionate was found at a low concentration. Contrarily to the Tournemire case, fumarate was not present (or not analyzed) in the extracts. The extracts from the Opalinus clay were different from both the Callovo-Oxfordian and Toarcian rocks with a major contribution of acetate against the other LMWOA (Courdouan et al., 2007b).
Composition, molecular weight and functional groups of the main LMWOA found in this study.
LMWOA | Carbon | Molecular weight (g·mol−1) |
pKa | Formula | Structural formula |
Formic acid | C1 | 46 | 3.77 | C H2O2 | HCOOH |
Acetic acid | C2 | 60 | 4.76 | C2H4O2 | CH3–COOH |
Propionic acid | C3 | 74 | 4.88 | C3H6O2 | CH3–CH2–COOH |
Lactic acid | C3 | 90 | 3.86 | C3H6O3 | CH3–CH(OH)–COOH |
Fumaric acid | C4 | 116 | 3.03/4.44 | C4H4O4 | HOOC–CH = CH–COOH |
3.3 Effects of disturbed conditions
3.3.1 Effect of pH
As shown in Table 3, the DOC concentration was higher using SW pH 9 (series 2), by a factor of 3 at 500 g·L−1 and a factor of 1.5 at 1500 g·L−1. The LMWOA total concentration was not significantly increased and represented only 14–19 % of the total DOC (39–29 % in the reference series 1a). More alkaline pH conditions increase the solubility of high molecular weight organic acids (Elie et al., 2004) and favor a kerogen hydrolysis. The organic compounds solubility depends on the charge density, which in turn depends on the pKa distribution, the pH of aqueous solutions and the polar properties of organic compounds. Therefore, LMWOA proportion is lower at higher pH values. This is in agreement with the behavior of the Opalinus clay, where more organic compounds of higher molecular weight were extracted by diluted NaOH solution than by deionized or synthetic pore water (Courdouan et al., 2007b).
The five organic acids present in the latter case (series 1a) were also identified under the more alkaline conditions. Acetate, formate, fumarate and propionate concentrations were not affected by the pH increase, whereas lactate concentration was raised by a factor of 1.5 approximately.
3.3.2 Effect of a short-term exposure to oxygen
The DOC content increased by one third after a short-term oxygen exposure of the crushed rock material (9.3 mg·L−1 at 500 g·L−1 and 24.7 mg·L−1 at 1500 g·L−1, Table 3). This suggests an additional DOM release, as already observed in previous studies (Blanchart et al., 2012; Courdouan et al., 2007a, 2007b; Elie et al., 2004) due to the formation of low molecular weight acids from the kerogen oxidation (Faure et al., 1999). Therefore, the LMWOA represented a higher proportion of the DOC extracted after a short-term oxygen exposure (45 % of DOC) than under anoxic conditions.
Again, the same five organic acids were identified in the oxic case. As shown in Fig. 1, same fumarate concentrations were obtained from rock material under both oxic and anoxic conditions. On the contrary, acetate, formate, lactate and propionate concentrations were about three times higher after the short oxic stage. Incorporation of oxygen during oxidation modifies the structure of kerogen and enhances its reactivity. Kerogen oxidation tends to release high molecular weight compounds (Blanchart et al., 2012; Elie et al., 2004), which could not be identified here by IC. Moreover, oxidation enhances the polarity of organic compounds after oxygen incorporation. Consequently, these compounds become more hydrophilic and more easily leached from the rock material (Courdouan et al., 2007a, 2007b).
3.3.3 Effect of temperature
The effect of a lower temperature was investigated by comparing DOM extracted for 1 day with SW at 25 °C (series 4) and 60 °C (series 1a). Regardless of the solid-to-liquid ratio, DOC concentration increases with temperature by a factor of 3 (Table 3). The LMWOA proportion of DOM decreased with increasing temperature (95–73% DOC at 25 °C, 39–29% at 60 °C). The increase in temperature is correlated with the release of higher molecular weight polar compounds (not identified by IC) in the leachates from the argillite solid phase (Drouin, 2007).
4 Conclusion
The characterization of dissolved organic matter (DOM) release from the clayey Toarcian formation sampled in Tournemire URL reveals that the DOM present in the pore water of this rock represents less than 0.1 % of the total organic carbon (TOC). The low molecular weight organic acids (LMWOA) proportion in this dissolved organic carbon (DOC) is about 38.5% under anoxic conditions at 60 °C (500 g·L−1 ratio). The main LMWOA are acetate, formate, fumarate, lactate and, to a lesser extent, propionate. DOC is mostly influenced by both the temperature and pH. The LMWOA nature and release are more specifically affected by a short exposure to oxygen whereby LMWOA concentration is multiplied by 2. Therefore, from an experimental point of view, strictly anoxic conditions during sampling, storage and analyses are required to evaluate DOM properties and reactivity in argillaceous rocks. Furthermore, in the context of radioactive waste disposal, the dissolution and formation of LMWOA during the oxic transient (due to gallery excavation) or the thermal transient (due to the heat-emitting waste) cannot be excluded during radioactive waste disposal, which may modify complexation capacity of OM with radionuclides and enhance bacterial development.
Acknowledgements
Our colleague Alexandre Dauzères (IRSN) is acknowledged for access to the Tournemire URL and his help during sampling.