1 Introduction
The significance of peridotites and associated rocks exposed in mountain belts is a main issue to understand orogenic systems. In the Pyrenees, the geological setting of the lherzolites bodies and associated breccias, more particularly their relationships with host rocks, have long been debated. Since their discovery in the late 18th century around the Lers Lake in the French central Pyrenees, various emplacement scenarios have been proposed, involving magmatic, tectonic, sedimentary or fluids-assisted processes, operating in divergent or convergent settings (see Lagabrielle and Bodinier, 2008 and references therein). Recent works on the Pyrenean lherzolites have shown that two main types of geological setting can be distinguished (Lagabrielle et al., 2010): (i) a “sedimentary” type (S-type), in which the peridotites constitute clasts of various sizes within monogenic or polymictic sedimentary breccia reworking Mesozoic metasediments, and (ii) a “tectonic” type (T-type), in which the mantle rocks form tectonic lenses associated with crustal slices, interpreted as remnants of mid-Cretaceous detachments faults inverted during the Late Cretaceous–Cenozoic orogeny.
This recent reinterpretation of the lherzolite emplacement has caused a spectacular revival of the Pyrenean geology, which was somewhat dormant since the studies following the ECORS program during the 1980s (Choukroune et al., 1989). The major breakthrough is the similarity between the Pyrenean peridotite-bearing sedimentary breccia and the geological formations exposed at the present-day continent–ocean transition in the distal part of continental margins. These formations show fine to coarse breccias and turbidites reworking exhumed mantle rocks (Boillot et al., 1985; Lagabrielle and Auzende, 1982). Many authors now interpret this type of formation as evidencing hyper-extended passive margins (Whitmarsh et al., 2001), as recently proposed in the Alps (Manatschal, 2004) and the Pyrenees (Lagabrielle and Bodinier, 2008; Lagabrielle et al., 2010). The presence of these types of peridotite-bearing breccia is a first-order constraint on the Pyrenean pre-orogenic geodynamic setting, and shows that the Pyrenees could now be considered as a fossil hyper-extended passive margin, even though rifting aborted and no oceanisation occurred.
The S-type lherzolite model essentially relies on geological descriptions in the well-studied Lherz area (Clerc et al., 2012; Lagabrielle and Bodinier, 2008; Lagabrielle et al., 2016). The purpose of this article is to present new geological data on a poorly described region east of Lherz, which also contains spectacular examples of peridotite–carbonate sedimentary breccias. We show that these data support and further constrain the S-type peridotite formation model and the condition of their inversion during the Pyrenean orogeny.
2 Geological setting
The Pyrenean mountain belt was formed along the boundary between the Iberia and European plates and results from the Late Cretaceous–Cenozoic inversion of a Cretaceous transcurrent hyper-extended rift created during the opening of the Bay of Biscay (Choukroune and Mattauer, 1978; Choukroune et al., 1973; Jammes et al., 2009; Lagabrielle and Bodinier, 2008; Le Pichon et al., 1970; Olivet, 1996).
The Pyrenees are characterized by a structural zonation parallel to the elongation of the belt (Fig. 1a). Our study area is located in the narrow internal metamorphic zone (IMZ), in the southern part of the North Pyrenean Zone (NPZ) (Choukroune, 1976). It is separated from the Axial Zone (AZ) by the North Pyrenean Fault (NPF), which constitutes the limit between the Iberian and Eurasian plates (Van der Voo and Boessenkol, 1973). The IMZ contains low- to high-grade Mesozoic metasedimentary rocks (HT–LP metamorphism, 110–85 Ma: Albarède and Michard-Vitrac, 1978; Clerc et al., 2015; Golberg and Leyreloup, 1990; Golberg and Maluski, 1988), carbonate breccias enclosing peridotite fragments (Clerc et al., 2012; Lagabrielle and Bodinier, 2008; Lagabrielle et al., 2010), meso- to catazonal Variscan units (the North Pyrenean Massifs, NPM) (St Blanquat et al., 1990; Vielzeuf, 1984), and alkaline intrusive and effusive magmatic rocks (Azambre and Rossy, 1976; Garralda, 2013; Le Fur Balouet and Cabanis, 1988).
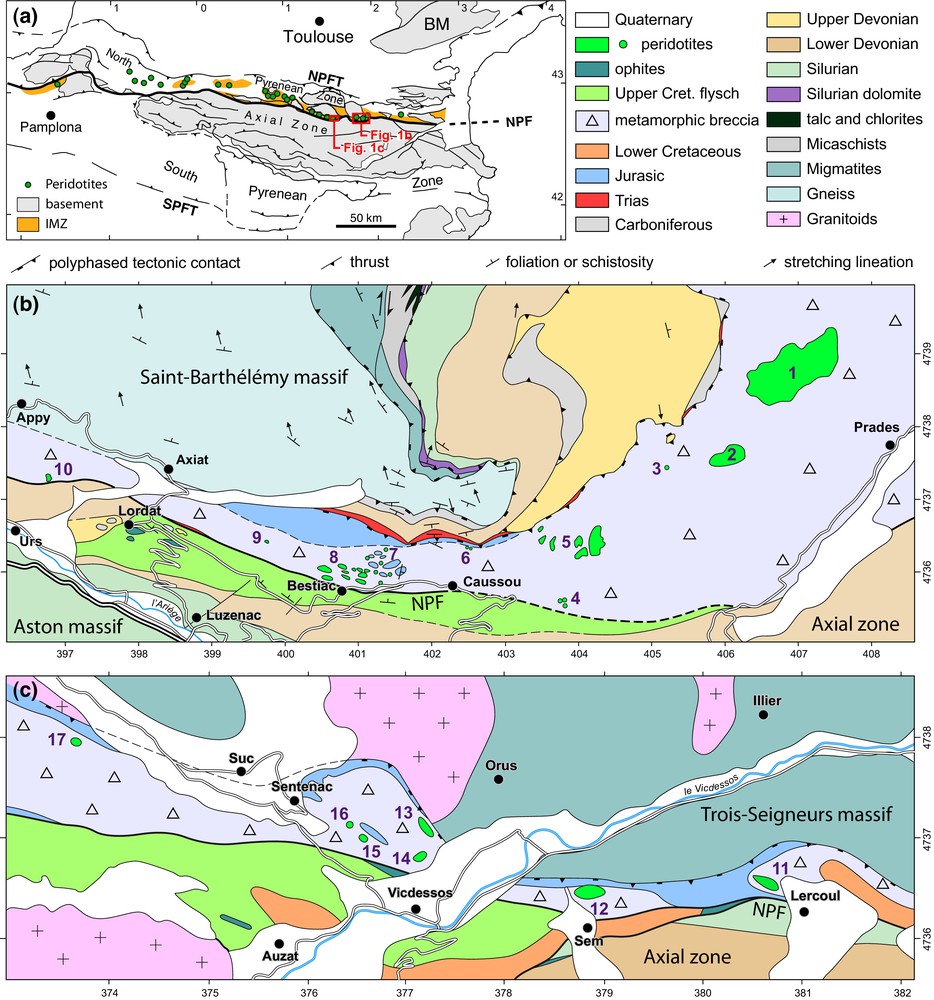
Geological maps of the studied areas (modified from Castéras et al., 1969 and Monod, 2013): (a) location of the two studied areas on the structural map of the Pyrenees; (b) geological map of the Prades–Caussou–Bestiac area; (c) geological map of the Vicdessos area. (b) and (c): UTM 31T grid (km). Location and name of the peridotite massifs: 1, Bois de la Pinouse, 2, Pic de Géral, 3, Rocher de Scaramus, 4, Amblaux, 5, Bois du Fajou, 6, Caussou, 7, former road of the Trimouns talc quarry, 8, Bestiac, 9, Pic Calmont, 10, Urs, 11, Croix de Sainte Tanoque, 12, Sem, 13, Les Rouges, 14, Porteteny, 15, lower Pic Couder, 16, upper Pic Couder, 17, Roc de Souberque.
The close spatial association in a narrow elongate HT–LP metamorphic zone of such a variety of protoliths questioned generations of geologists who have proposed different models of lithospheric extension centered on that area (Dauteuil and Ricou, 1989; Golberg and Leyreloup, 1990; St Blanquat et al., 1986; Vielzeuf and Kornprobst, 1984). Recent works have demonstrated that the IMZ is the exposed remnants of an area that experienced an extreme crustal thinning, leading to the exhumation and denudation of the subcontinental mantle (Clerc et al., 2015; Clerc and Lagabrielle, 2014; Lagabrielle and Bodinier, 2008; Lagabrielle et al., 2010).
The studied peridotites and associated breccias are located in the IMZ between the Trois-Seigneurs and Saint-Barthélémy NPM and the Aston gneiss dome of the AZ (Fig. 1b and c). In this area, the IMZ could be very thin (sometimes less than 100 m), and pinched between the AZ and the NPM by Late Cretaceous–Cenozoic inversion. Its basal contact is underlined by Triassic evaporites and is a major pre- and synorogenic polyphased tectonic contact. The IMZ is constituted by anastomosed tectonic lenses of metamorphic Mesozoic rocks, which are gradually brecciated upward. The lherzolite-bearing breccias are located at different structural levels within the IMZ, but are mainly associated with the principal polymictic breccia layer. The Mesozoic metamorphic carbonates show a first phase of syn-metamorphic ductile deformation, and subsequent phases of folding and fracturing (Clerc et al., 2015). The IMZ is in fault contact with the Turonian to Lower Senonian flysches that are variously metamorphosed and deformed.
The presence of peridotite is one of the original and main geological characteristics of the Pyrenees. Monchoux (1970, 1971) provides a field description and a petrological characterization of all the known peridotite massifs of the range (Fig. 1a). Recent structural and geochemical studies show that the lherzolites were formed from harzburgites by a refertilization reaction with basaltic magmas (Le Roux et al., 2007). The Pyrenean peridotites are variously serpentinized, some massifs being constituted by fresh peridotite, whereas others are completely serpentinized.
Since their discovery in the late 18th century by Lelièvre, an abundant literature was devoted to their emplacement process and geological significance. The most important advances in this topic are relatively recent and relate to the discovery of subcontinental mantle rocks at the base of the present-day passive margins (Boillot et al., 1985), followed by progresses in our understanding of the structure and formation of ocean–continent transitions (OCT) and associated mantle exhumation at hyper-extended passive margins. Sampling and drilling in these settings have shown that exhumed mantle rocks experienced tectono-sedimentary reworking and transport leading to local accumulation of ultramafic-rich clastic sedimentary sequences such as fine-grained turbidites, debris-flows and rock slides (Lagabrielle and Auzende, 1982; Manatschal, 2004; Whitmarsh et al., 2001). The similarity between these formations and the gravity-emplaced mafic and ultramafic-rich bodies reported from various orogenic belts such as the Appenine (Abbate et al., 1970; Marroni and Pandolfi, 2001), the Californian Coast Ranges (e.g., Lockwood, 1971), or the internal Alps (Deville et al., 1992; Masini et al., 2012) has renewed the interpretation of the emplacement of orogenic peridotite in a direction already suggested by some pioneering works (Lemoine et al., 1987), some of them from the Pyrenean example (Duée et al., 1984; Fortané et al., 1986).
3 Description of the studied peridotite massifs
During this work, detailed geological maps were realized at the 1/1000 scale, and the geometry of contacts was drawn, when possible, with the help of continuous GPS mapping. The location and the main characteristics of the studied peridotites are summarized in Table SM 1 in Supplementary Material. They can be divided into an eastern sector, including the Prades, Caussou and Bestiac areas (Fig. 1b), and a western sector, including all the massifs outcropping around Vicdessos (Fig. 1c). Due to limited printed space, all the photos and detailed maps cannot be presented directly in this article, but they are available in the Supplementary Material. We present no detailed maps for the Rocher de Scaramus, Caussou, Croix-de-Sainte-Tannoque, Pic Coudère inf. and sup., and Roc de Souberque bodies, due to their small size and/or because of the absence of outcropping contacts.
3.1 Prades area (Bois de la Pinouse, Pic de Geral, Rocher de Scaramus bodies)
The easternmost studied peridotite massif is the Bois de la Pinouse massif above the Prades village (Fig. 1b). With its kilometric size, it is one of the largest massifs of the Pyrenees (Fig. SM 1). We observe gradual and brecciated contacts all around the massif (Fig. 2a) that support the hypothesis of an S-type peridotite thus opposing to the former interpretation of fault-bounded massif (Debroas et al., 1977). The outcropping conditions are not good enough to determine, whether it is composed of a single large or several smaller brecciated lenses of peridotite. The relationship between the mapped contact and topography suggests that the peridotite body is roughly horizontal. Due to the vegetal cover, the outcropping conditions of the Pic de Geral massif are not good, except along the summit ridge and west side. We did not find any outcrop of the contact, but loose blocks with brecciated lherzolite and ophicalcites were found in some places (Fig. SM 1). East of the Pic de Geral massif and below the Rocher de Scaramus, we have found two decametric lenses of brecciated peridotites that had never been described before (Fig. SM 2a). All these massifs are comprised of fresh lherzolite and are only locally affected by planar fissural serpentinisation associated with slip surfaces (Fig. SM 2b). The matrix of the surrounding metamorphic polymictic breccia of the IMZ within which all the peridotites are embedded seals these surfaces.
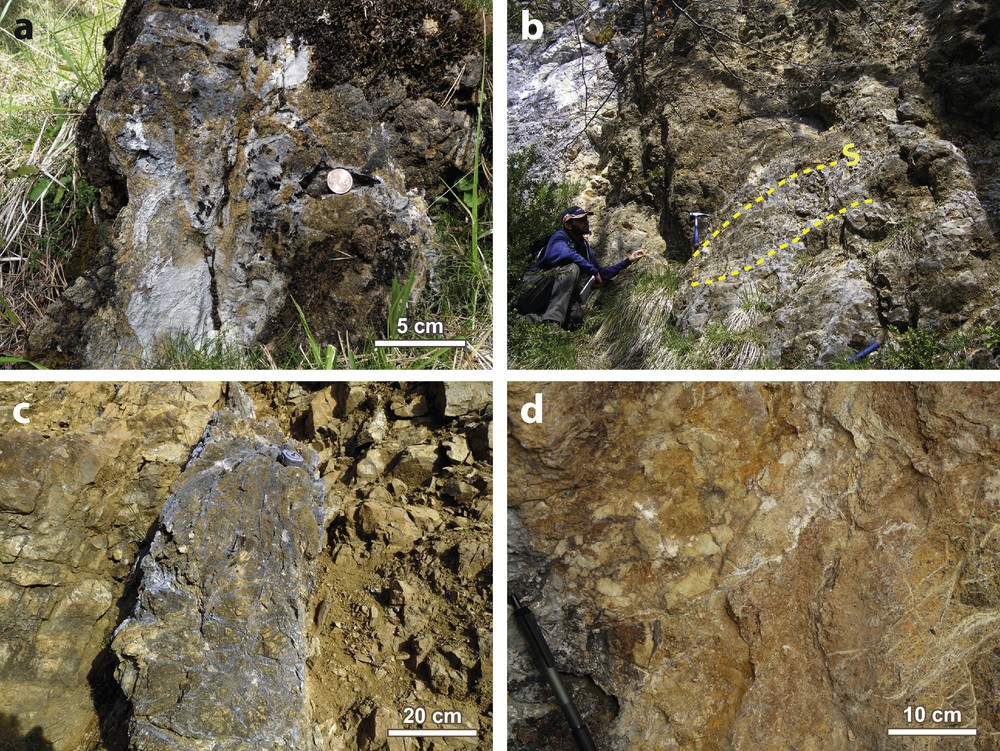
Field photographs of the peridotites in the Prades–Caussou area; (a) brecciated contact in the Bois de la Pinouse massif; (b) brecciated contact in the Bois du Fajou massif, with bedding underlined (S0); (c) meter scale Neptunian-like ophicalcite dyke in the Bois du Fajou massif; (d) brecciated contact in the Amblaux massif. See location of photos in Figs. SM 1, SM 3, and SM4.
3.2 Caussou area (Bois du Fajou, Amblaux, and Caussou bodies)
Our detailed mapping of the Bois du Fajou massif allowed us to precise the contours proposed by Monchoux (1970). The massif, which outcrops in a high relief area and is not easily accessible, consists in seven sub-vertical lenses of various sizes, from 50 to 500 m long (Fig. SM 3). Portions of the contact between the peridotite and the surrounding breccia are visible around each lens, and, in all cases, we observe a progressive contact of variable thickness between massive peridotite and polymictic carbonate breccia (Fig. 2b). In the larger lens, the peridotite is cut by decametric long and meter wide parallel fractures filled by peridotite and marbles blocks cemented in a crystallized carbonate matrix (Fig. 2c). The serpentinisation is generally weak or absent, but as in the previous sector, we observe some serpentinite veins associated with slip surfaces. In a few locations, we found a high density of massive serpentinite veins, and a massive serpentinite body in a single place. Previously considered as a single unit, the Amblaux massif is in fact constituted by numerous – at least ten – small peridotite lenses (Fig. SM 4). The northern part of the massif considered by Lacroix (1894) as a representative example of an intrusive contact of the lherzolite inside the marbles is in fact formed by small peridotite blocks, and their rounded appearance is due to superficial precipitation of carbonates. All the contacts are progressive (Fig. 2d). The Amblaux massif is a rare example of amphibole-peridotite and is serpentine-free. Just above the village of Caussou, we discovered small lenses of peridotite breccia similar to those of the Rocher de Scaramus.
3.3 Bestiac area (Bestiac, Pic Calmont, Urs bodies)
The Bestiac area represents the most spectacular examples of S-type peridotites in the Pyrenees thanks to its exceptional outcropping conditions and easy accessibility. A first group of previously unknown outcrops was observed all along the former road to the Trimouns talc quarry. They consist in small outcrops of metric size composed of peridotitic breccias (Figs. 3 and 4a) interbedded within the main breccia of the IMZ. The main bodies are located on the slope north of the Bestiac village.
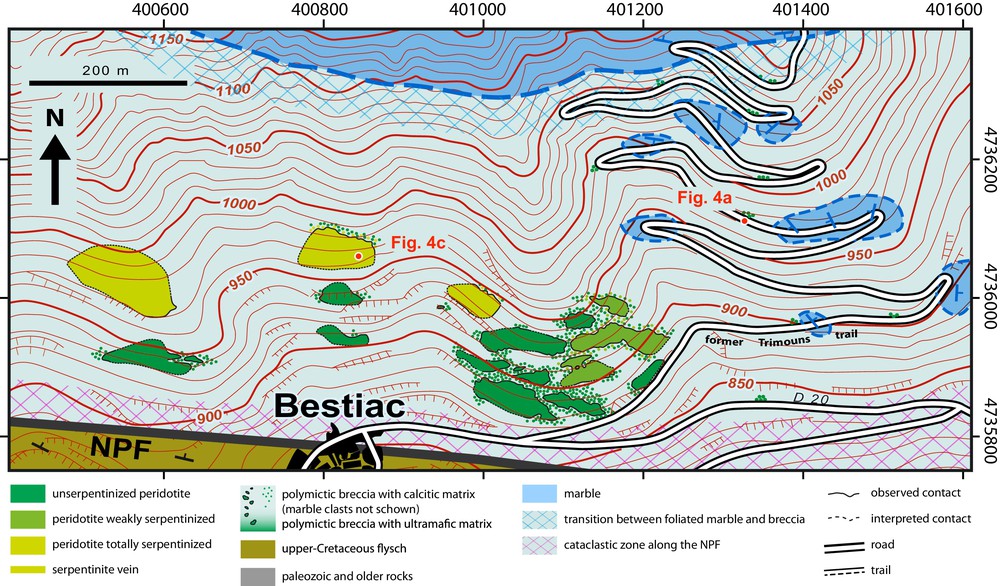
General map of the Bestiac area. UTM 31T grid (m).

Field photographs of the peridotite of the Bestiac area; (a) example of one of the small isolated peridotite clast in the polymictic breccia along the Trimouns quarry former road; (b) lherzolite cut by two networks of serpentinite veins in the Bestiac massif, black V1 and green V2; (c) totally serpentinized peridotite in the Bestiac massif; (d) sedimentary layering of the breccia in the Bestiac massif; (e) polymictic breccia with ultramafic matrix and marble clasts, Bestiac massif; (f) sedimentary reworking of the peridotite in the Urs massif. See location of photos a–e in Figs. 3 and 5.
3.3.1 The Bestiac body
The general map of the Bestiac area is given in Fig. 3 and a detailed map of the eastern part is given in Fig. 5. The Bestiac massif is comprised of about twenty sub-vertical lenses of brecciated peridotite, mainly spinel-lherzolite. The peridotite exhibits a clear magmatic layering in a few places. One unique feature of Bestiac massif is its serpentinisation: with the Bois du Fajou massifs, it is the only Pyrenean peridotitic massif known to date showing a mixture between peridotite blocks that have undergone different serpentinisation histories. Most of the lenses are constituted by fresh lherzolite. Others are made of fresh lherzolites cut by at least two generations of serpentines veins (Fig. 4b). Finally, three lenses are constituted by totally serpentinized peridotite (Fig. 4c). All the visible contacts are progressive and in a few places the breccia exhibits clear sedimentary bedding (Fig. 4d). In some places, non-serpentinized peridotitic fragments are located close to the edges of totally serpentinized large lenses. The breccia is polymictic and mainly composed of lherzolite fragments cemented in a crystallized calcite matrix, but often evolves toward marble fragments cemented in an ultramafic matrix (Fig. 4e). The marble clasts are mainly constituted by white-gray marbles of probably Jurassic age, but also by blocks of various colors (black, beige, brown…) of unknown age (Palaeozoic? Lower Cretaceous?) Rare clasts of pelite were observed. The matrix of the breccia is slightly recrystallized and contains metamorphic minerals, mainly white micas. All the lenses of peridotite are cut by carbonate-filled fractures forming ophicalcites (Clerc et al., 2014), especially near their contact with host rock. The orientation of structural elements is given in Fig. 6, and clearly shows the relative chronology between pre-, syn- and post-brecciation events: the spatial coherence increases from intra-peridotite or intra-marbles structural elements, like magmatic and tectonic foliations and serpentinite veins, to the bedding of the breccia, and finally to the late fracture cleavage and faults that affect the whole breccia and show a north–south shortening (Fig. 6).
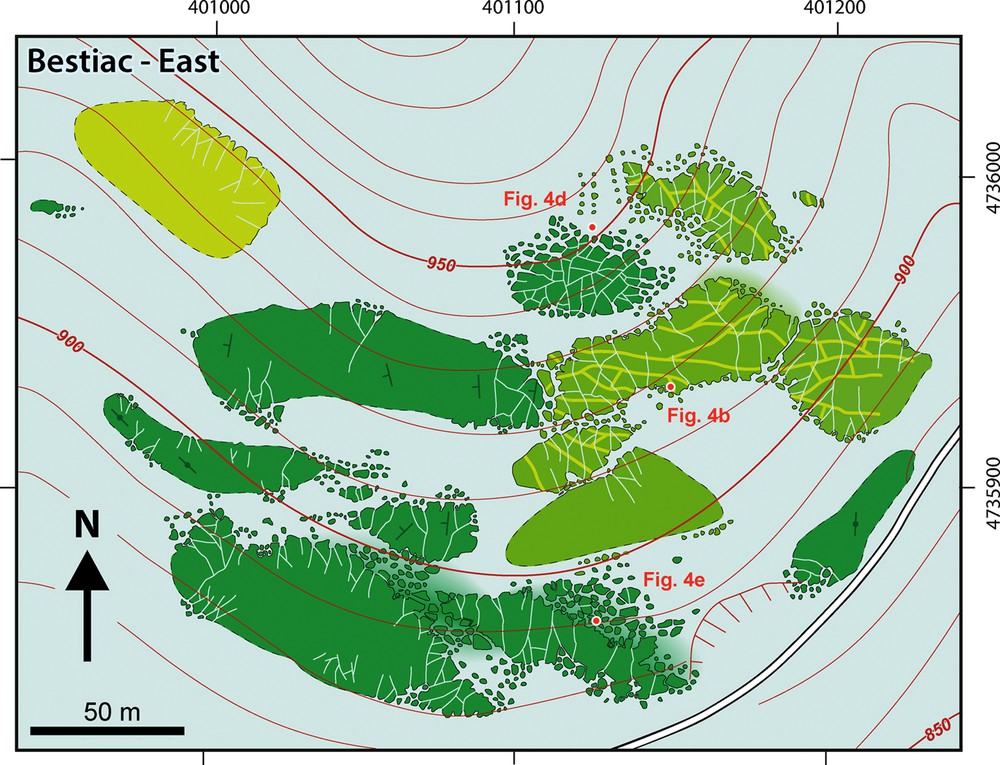
Detailed map of the eastern part of the Bestiac massif. UTM 31T grid (m). See Fig. 3 for a caption.
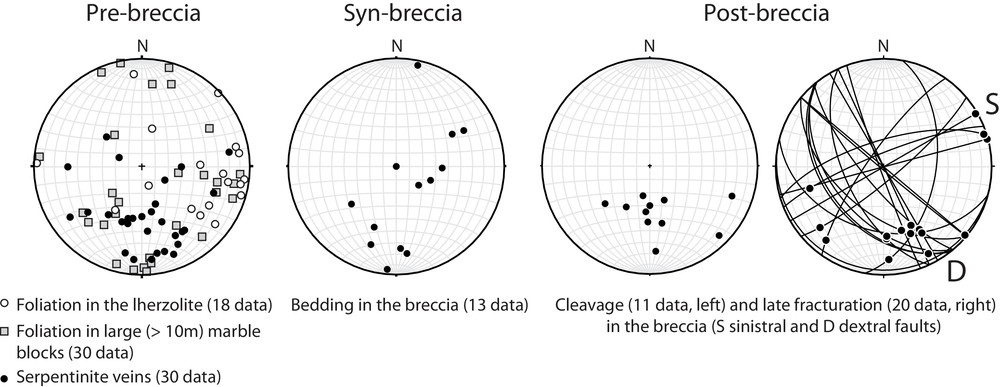
Structural data from the Bestiac area organized chronologically with respect to the field relationships (equal area, lower hemisphere).
3.3.2 The Pic Calmont body
The Pic Calmont body is located close to the Bestiac massif, in a northern structural position, near the tectonic lenses of massive Mesozoic marbles, which constitute the northern part of the IMZ in this area. It is composed of a unique small lens of brecciated peridotite, whose dimensions are 30 m × 10 m and which follows an east–west long axis (Fig. SM 5). It is comprised of fresh normal lherzolite.
3.3.3 Urs body
Urs body is structurally located on the southern side of the IMZ, close to the NPF (Fig. 1b). It is constituted by a single pluridecametric 70 m × 20 m vertical lens of brecciated fresh lherzolite, which is elongated along a NNE–SSW direction (Fig. SM 6). The sedimentary reworking of the lherzolite is spectacularly exposed (Fig. 4f) and more or less rounded lherzolite fragments are found far from the main brecciated lens.
3.4 Vicdessos area (Croix de Sainte Tanoque, Sem, Les Rouges, Porteteny, lower and upper Pic Coudère and Roc de Souberque bodies)
The best exposed massif in this area is Les Rouges, but more or less all the massifs of this area are not as well exposed as the ones described above (Figs. SM 7 and 8). They are mainly constituted by lherzolite, and are all characterized by a progressive transition between the peridotite and the surrounding breccia, except perhaps the lower Pic Couder and Croix de Sainte Tanoque massifs, where no contact is visible. These massifs are generally weakly serpentinized by late fissural serpentinite veins, except for the Croix de Sainte Tanoque massif, which shows an increasing serpentinisation gradient from east to west. Typical features visible in these massifs are presented in Fig. SM 9.
4 Synthesis
4.1 Common characteristics
4.1.1 Structural position
All the breccia and enclosed peridotites are located above (Prades, Caussou and Vicdessos area) or south (Bestiac area) of a set of tectonic slices of ductily deformed Mesozoic marbles. The transition between the breccia and the marble is progressive.
4.1.2 Map view pattern
All the studied peridotite massifs show the same pattern in map view. They are constituted by one or several peridotitic bodies which all have an elongated lens shape, which is parallel to the main structural trend of the IMZ. These bodies are fragmented and contain fractures filled by a mixture of carbonated and peridotite clasts and a calcitic or ultramafic matrix. The map pattern clearly shows a fragmentation and a dispersion of both the peridotite and the marbles in the breccia.
4.1.3 Nature of contacts
The contacts of all the studied massifs are irregular and not deformed. No fault or shear marker was observed between peridotitic fragments of any size and the enclosing breccia. The continuity between the calcite veins inside the peridotite fragments and the matrix of the surrounding breccia clearly underlines the sedimentary nature of the contact.
4.1.4 Nature and organization of the breccia
All the studied peridotite massifs and fragments are enclosed in a polymictic breccia made principally of deformed metasediments of various ages within a calcitic matrix which contains metamorphic minerals. The breccia is mainly bimodal (metasediment – peridotite) and is characterized by progressive transitions of various thicknesses between 100% carbonate and 100% ultramafic layers, for both the matrix and the fragments. This breccia is poorly sorted, but from place to place, there is a planar organization of the elements. The elongation and alignment of the main peridotite dismembered lenses define by itself the bedding of the breccia at the decametric to hectometric scale.
4.2 Differences
4.2.1 Nature of the peridotite
Despite the fact that the majority of the studied peridotites are made of spinel-lherzolites, previous authors (Monchoux, 1971) and our ongoing works show that the petrology of the peridotite differs from one massif to the other and is sometimes variable inside a single massif. This is obvious especially in Bestiac, where the protolith of one of the totally serpentinized lens was probably harzburgitic (Delacour, pers. com.). This variability is also underlined by the presence of variable amounts of amphiboles in the Amblaux, Bois du Fajou, Caussou and Bestiac massifs.
4.2.2 Serpentinisation history
In most of the studied massifs, the serpentinisation is weak and only fissural, but our mapping clearly shows that some bodies, in particular Bestiac, are comprised of variously serpentinized blocks. This shows first that the main phase of serpentinisation predates the breccia formation, and second that the denudation history of the peridotites sampled in the breccia may be heterogeneous at the massif's scale. This suggests a tectonic context where fragments of peridotites from different structural levels were sampled more or less synchronously.
5 Discussion
As detailed below, the similarities (map pattern, nature of the contacts, organization of the carbonate-peridotite breccia), but also the differences (observation at the same place of peridotites of different compositions with various serpentinisation histories), collectively indicate the involvement of tectono-sedimentary processes active on the basin-floor to account for the formation of these breccia.
The map pattern and the detailed study of the peridotite–breccia contacts clearly indicate simultaneous sedimentary reworking of both the peridotite and the Mesozoic metamorphic series at the surface. Both the non-peridotitic and peridotitic clasts (different types of marbles, lherzolites, serpentinites…) are poorly sorted and texturally immature (dominant angular clasts). Although the elongation and alignment of the main peridotite dismembered lenses define the general bedding of the breccia, its internal geometry is generally poorly defined. These observations suggest that the breccia was deposited in a tectonically active environment with a relatively short travel distance for the peridotite and marble fragments. Any model of emplacement should explain how the peridotites are supplied to a basin and mixed with sediments derived from erosion and collapse of the supra-Permian Mesozoic cover with only very rare contribution of the lower crustal layers (Palaeozoic series and crystalline basement). The model proposed by Lagabrielle et al. (2010) and Clerc et al. (2012) involving tectono-sedimentary processes associated with mantle exhumation in a context of narrow intracontinental basins suffering extreme crustal thinning is in agreement with all our new data.
The originality of our finding is that we show that all the studied peridotites share a common emplacement mode, but could differ by their pre-brecciation history, i.e. by their magmatic evolution within the mantle, and/or by their exhumation history from mantle condition to the Earth's surface. This suggests that the structural context that exhumed the mantle allowed more or less simultaneously the sampling of fragments from different depths and their incorporation into the same breccia. This is not easy to achieve in a pure cylindrical extensional setting, and is better explained by strike-slip faults cutting zoned slices of exhumed mantle, marked by a gradient of serpentinisation.
The breccia is characterized by the absence of syn-brecciation tectonic structures such as faults and shear planes, at any scale. In some rare places and mainly along major tectonic contacts like the NPF, we can observe the development of sparse fracture cleavage and conjugate strike-slip faults that can be correlated to the inversion events. Consequently, the formation of the breccia clearly predates the inversion, and therefore could not be attributed to a syn-inversion tectonic cataclasis event. As the beginning of inversion is well defined at ca. 85 Ma at the scale of the whole Pyrenees (Dubois and Seguin, 1978), this defines a youngest age for the brecciation event. The other main constraint is the presence of fragments of Mesozoic carbonate and pelitic rocks metamorphosed before their incorporation into the breccia. As the HT–LP metamorphic event started around 105–110 Ma (Clerc et al., 2015; Golberg and Maluski, 1988), it defines an older age and consequently a maximum duration of around 20–25 Ma for the brecciation event. As the matrix of the breccia is slightly metamorphosed and contains neoformed minerals, we posit that it was formed at the end rather than at the beginning of this time period. The burial, consolidation, and moderate heating of the breccia may have occurred before and/or just at the beginning of inversion.
6 Conclusions
We have remapped all the peridotite massifs located in eastern Ariège, in the Prades, Caussou, Bestiac, and Vicdessos areas. This includes 17 peridotite massifs among which 3 were unknown and 10 are constituted by large hectometric to pluri-hectometric lenses. Our detailed geological maps and field relationships allow us to draw the following conclusions.
- 1. Numerous peridotite outcrops, not described so far, are constituted by decametric to sub-metric bodies, which are scattered between and around the main larger outcrops identified by previous workers and described by Monchoux (1970). This shows a spatially more continuous outcropping of the peridotite breccia than previously thought, but this also shows that all peridotite exposures have probably not been discovered to date, particularly in remote areas.
- 2. In the studied area, the vast majority of peridotites form dismembered blocks and lenses of various sizes, from millimetric monomineral grains and isolated decimetric blocks to pluri-hectometric olistoliths, embedded in debris flows involving mainly Mesozoic metamorphic sediments; the peridotite of the studied area can therefore be linked to the S-type peridotites of Lagabrielle et al. (2010), and are of the same nature as the peridotites of the Lherz region (Clerc et al., 2012). As such, their origin can be attributed to sedimentary processes in an active tectonic environment associated with mantle exhumation in a context of extreme crustal thinning and crustal breakoff.
- 3. All the studied massifs share the same mode of emplacement, but the constituting peridotites do not necessarily have the same origin and do not necessarily share the same history. The slightly different petrographic characteristics of these peridotites (lherzolite versus harzburgite, amount and nature of amphibole…) and the heterogeneity in their degree of serpentinisation, sometimes at the massif's scale, imply a specific mode of emplacement mixing mantle fragments of different origins and exhumation histories. This could suggest that the structural context that exhumed the mantle allowed more or less simultaneously the sampling of fragments from different depths. This is not easy to achieve in a pure cylindrical extensional setting, and suggests the presence of strike-slip faults that will cut zoned slices of exhumed mantle, marked by a gradient of serpentinisation and/or composition.
- 4. Field relationships clearly show that the formation of the breccia predates the inversion, and consequently cannot be attributed to a syn-inversion tectonic cataclasis event. This defines a Santonian minimum age (ca. 85 Ma) for the end of the brecciation event. The presence of metamorphic blocks in the breccia defines a maximum age of ca. 110 Ma for the beginning of the brecciation event. This gives a maximum duration of ca. 20–25 Ma for the emplacement of peridotite massifs and the breccia formation, more likely at the end rather than at the beginning of this time period. We posit that burial, consolidation, and moderate heating of the breccia have occurred just before and/or at the beginning of inversion.
Acknowledgements
This article is the synthesis of a series of Master's 1 degree mapping projects that were realized by FB, AGH, AP and MZ between 2007 and 2012. We gratefully acknowledge funding through the “Bureau de recherches géologiques et minières” (BRGM) geological mapping program and the ANR PYRAMID project. Thierry Nalpas and Thierry Baudin are thanked for their constructive comments and Marguerite Godard for final remarks and editorial handling. We would also like to thank Pierre Monchoux for sharing his memories in search of the location of some remote and vegetated outcrops, and Élie-Jean Debroas for vigorous discussions in the field. Christiane Cavaré-Hester and Anne-Marie Cousin are sincerely thanked for their help in drawing geological maps.