1 Introduction
The main source of sediment in the Amazon River and its major tributaries is Andean erosion (Filizola and Guyot, 2009; Filizola et al., 2011; Gibbs, 1967; Martinez et al., 2009; Meade et al., 1979). Most of the rivers originating in the Andes Mountains present a high-suspended load (80%) and low bed load (20%) (Meade et al., 1985). During transport from the Andes to the Atlantic Ocean, sediments are exposed to many processes: sedimentation, temporary storage, resuspension and reworking (Dunne et al., 1998; Moreira-Turcq et al., 2014; Santini et al., 2014). It indicates that this transport through lowland Amazonia involves sediment exchanges between the channel and the floodplain. These exchanges occur through bank erosion, bar deposition, and overbank sedimentation in floodplain (Irion et al., 2006; Moreira-Turcq et al., 2014). Sediment dynamics are complex, and floodplain deposits have been reworked and incorporated into Amazonian streams. The estimated rates of recycling are less than 5000 yr in the case of the modern floodplain of the downstream Óbidos in lower Amazon Basin and 2000 cal yr BP upstream (Mertes et al., 1996).
The lowland Amazon Basin shows a relatively immobile channel, unlike the strongly active migration processes and sedimentary dynamics in the upper Amazon (Quintana, 2015). Floodplains in the upper Amazon Basin are impacted by channel migrations that can be triggered by flood events (Jerolmack and Mohrig, 2007), sediment loads (Constantine et al., 2014) or drainage network segmentation induced by tectonic activity (Costa et al., 2001). Past fluvial dynamics are not well documented in this region. In general, channel lateral migration is related to Holocene neotectonics, which suggests that the shifting of rivers may be caused by either tilting of the basin surface or subsidence of blocks in the basin basement (Dumont, 1996; Dumont and Fournier, 1994; Franzinelli and Igreja, 2002; Latrubesse and Franzinelli, 2002; Räsänen et al., 1990). However, hydrological changes are also important, Constantine et al. (2014) suggested that rivers with high sediment loads experience annual migration rates greater than those of rivers with lower sediment loads. Meander cut-off occurred more frequently along rivers with higher sediment loads, and the authors concluded that the imposed sediment loads influenced the changes in fluvial processes in the lower Amazon Basin. Nevertheless, Quintana (2015) points out the importance of temporal and spatial scales to study fluvial dynamics. Finally, all these studies show that sediment deposition in Amazonia floodplain lakes is not constant over time and can be influenced strongly by several factors. Understanding how paleoclimatic changes and geomorphological processes can affect sedimentation in the Amazonian floodplain lakes remains a challenge.
The main purpose of this work is to understand and determine the main processes responsible for lacustrine deposition in floodplain lakes of the upper Amazon Basin during the late Holocene. For this, was collected four sedimentary cores in floodplain lakes from the Ucayali and Marañon meandering rivers, which present different characteristics in terms of sediment load, sinuosity and water flux.
2 Material and methods
2.1 Study area
The Amazon River drains the largest basin of the planet, with an estimated area of 6.1 × 106 km2 (Goulding et al., 2003) and is formed at the confluence of the Ucayali and Marañón Rivers in Peruvian Amazonia (Fig. 1A). The Ucayali River drains the southern part of the Peruvian Andean Cordillera and flows into the Marañón River, draining 360,000 km2, with a mean annual discharge of approximately 11,200 m3·s−1. The Marañón River drains 350,000 km2 from the northern and central parts of the Peruvian Andean Cordillera to its confluence with the Ucayali, with a mean annual discharge of 16,200 m3·s−1 (Espinoza-Villar et al., 2012).
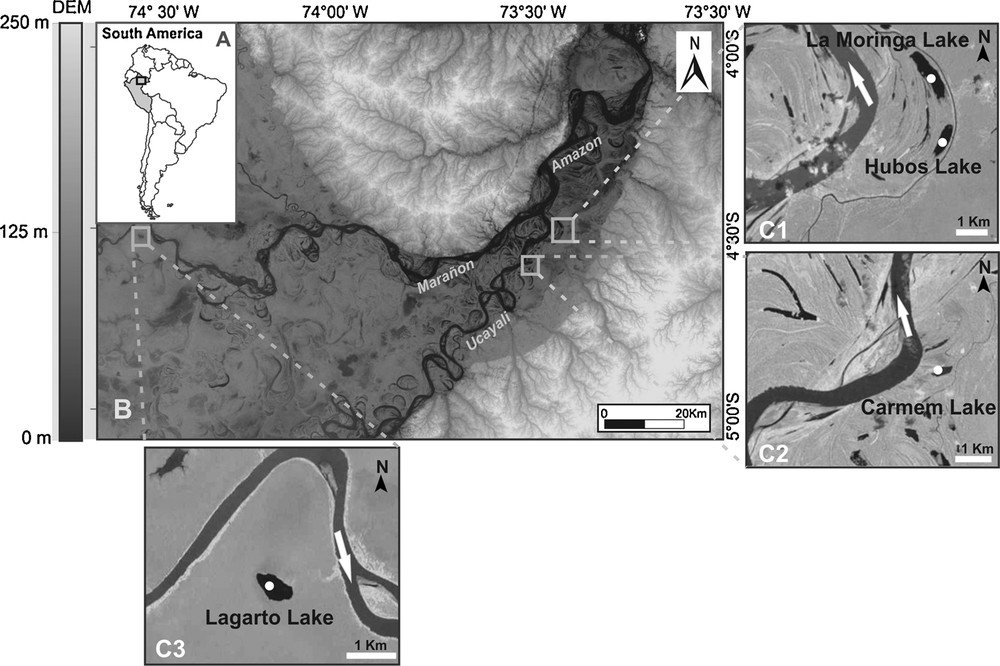
Location of the confluence of the Ucayali and Marañón Rivers. A, Peru location. B, DEM of the study area. C1, Lakes La Moringa and Hubos. C2, Lake Carmen. C3, Lake Lagarto in the Marañón floodplain.
The suspended sediment load varies according to the season. The annual average concentration of suspended sediment is 395 mg·L−1 at the Requena station (Ucayali River) and 173 mg·L−1 at the San Regis station (Marañón River) (Armijos et al., 2013). The width of the Ucayali River varies from 500 m to 1250 m. The average sinuosity is 1.94, the belt meandering current is 30 km wide and is characterized by multiple abandoned channels (Dumont, 1996). The width of the Marañón River varies from 1000 to 2500 m, with numerous islands in the straight sections. The average sinuosity is 1.33. The Marañón's flow is 14% greater than that of the Ucayali River. Average annual rainfall between 1946 and 1994 was of about 3080 mm·yr−1 (Marengo et al., 1998). Precipitation is most intense between January and May. The hydrological cycle is very similar in the Marañón and Ucayali rivers, with flood peaks occurring between March and May, and low waters between August and October.
The study area is in Peruvian Amazonia, in the department of Loreto between 5°00'S–74°40’W and 4°00'S–73°20’W (Fig. 1B). This study analysed three lakes in the Ucayali River floodplain: Lakes Hubos and La Moringa (Fig. 1C1), Lake Carmen (Fig. 1C2), and one lake in the Marañón River floodplain, Lake Lagarto (Fig. 1C3), which is located 200 km from the confluence with the Ucayali River.
2.2 Sampling and analyses
Four cores were collected in the upper Amazon Basin (Fig. 1). The CAR2 (4°35’23“S and 73°28‘.45”W) and HUB1 (4°30’29.19“S and 73°23‘.40”W) cores were collected in Lakes Carmen and Hubos using a “vibro-core.” The MOR1 (4°28‘48.61“S and 73°24’11.04”W) and LAG1 (4°31’04.81“S and 74°34’55.15”W) cores were collected manually in Lakes La Moringa and Lagarto, respectively. All cores were collected using aluminium tubes 7.5 cm in diameter. The CAR2, HUB1, MOR1 and LAG1 cores (measuring 600, 140, 120 and 98 cm, respectively) were opened, described and analysed with SCOPIX X-ray equipment at the EPOC (“Environnements et paléoenvironnements océaniques et continentaux”) laboratory at the University of Bordeaux-1 (France). Scopix uses classical X-ray equipment (X-ray source: 160 kV, 19 mA), coupled with new radioscopy instrumentation (CCD camera 756 Å ∼ 581 resolution), connected to a computer for data acquisition and processing (Migeon et al., 1998).
2.2.1 Water content and bulk density
Soon after opening, the sediment was sampled in aluminium U-channels to determine sediment bulk density and water content. Sub-samples were taken every 1 cm along the cores, and the water content was measured after oven drying at 40 °C for several days to obtain a final stable weight.
2.2.2 Radiocarbon dating
14C measurements were performed with an Artemis accelerator mass spectrometry (AMS) system based on a 3-MV Pelletron from National Electrostatics Corporation (NEC, Middleton, Wisconsin, USA) at the “Laboratoire de mesure du carbone 14” (LMC14) — UMS 2572 (CEA/DSM CNRS IRD IRSN—“Ministère de la Culture et de la Communication”). The age–depth model was built using CLAM (non-Bayesian code ‘classical’ age-depth models). CLAM is written in the open-source R statistical environment and allows calibration of 14C dates, building an age model based on decisions made by the user, and producing graphs and text files (Blaauw, 2010). The four cores were dated, and the age models use the IntCal 13 14C calibration curve and a polynomial regression. Calibrated ages are expressed in conventional cal yr BP.
2.2.3 Particle size analysis
A laser particle size analyser (CILAS 1064) was used to measure the grain-size distribution. Samples were treated with hydrogen peroxide to remove organic matter, and a sodium hexametaphosphate (Na16P14O43) solution (40 mg·L−1) was used to assist in dispersing the particles.
2.2.4 Elemental carbon composition
Total organic carbon (TOC) composition was determined with a CHN analyser (FISIONS NA-2000) at the University of California, Davis, CA, USA. Samples were first treated with 0.5 N HCl to remove carbonates.
2.2.5 Digital Elevation Model (DEM)
Free Landsat-5 sensor/TM images were acquired through the INPE (“Instituto Nacional de Pesquisas Espaciais”) website (http://www.dgi.inpe.br/CDSR/), using the 006/063 scene. Free SRTM (Shuttle Radar Topography Mission) data were available on the US Geological Survey website (http://www.gdex.cr.usgs.gov/gdex/), with a resolution of 30 m. Data were processed using Global Mapper 13 software, where different colour levels were assigned to highlight the different dimensions of the region and to evidence morphological and topographical features (Fig. 1B).
3 Results
3.1 Chronology and lithology
Eighteen samples along the CAR2 core were dated and presented an older age of 4612 cal yr BP (Table 1). Eight radiocarbon dates were obtained from the HUB1 core, with an age of 3215 cal yr BP for the basal section (Table 1). Twelve TOC AMS radiocarbon dates were obtained for the MOR1 core, with an age of 2961 cal yrs BP (Table 1). In the MOR1 and CAR2 cores collected in the Ucayali floodplain, the age model (Fig. 2) was based on a cubic-spline curve to the calibrated 14C ages and in the HUB1 core, the age model was based on a polynomial regression. A gap in sedimentation records was observed in the three sediment cores. This gap is well dated in the HUB1 record and occurs between 2950 and 719 cal yr BP. A sediment package of remobilized sediment, likely due to reworking of older sediment, occurred at the top of the three cores and was not considered in the age model. Four TOC AMS dates defined the sedimentary chronology of the LAG1 core, with a basal age of 630 cal yr BP and a probably modern top age (Fig. 2). No upper package of reworked sediment is observed in this core.
Radiocarbon and calibrated dates of the LAG1, CAR2, HUB1 and MOR1 core samples.
Core | Lab. Number | Depth (cm) | Radiocarbon age | Calibrated age (cal BP) | cal BP ages (2 sigma) |
LAG1 (Maranon) | SacA 24998 | 0–1 | 110 ± 30 | 115 | 12–269 |
SacA 24999 | 29–30 | 310 ± 30 | 400 | 301–463 | |
SacA 25000 | 62–63 | 445 ± 30 | 607 | 465–534 | |
SacA 25001 | 97–98 | 605 ± 30 | 630 | 545–653 | |
CAR2 (Ucayali) | Beta 319436 | 0–1 | Post-modern | Post-1955 | – |
SacA 29547 | 38–39 | 2105 ± 30 | 2077 | 1997–2148 | |
SacA 29548 | 61–62 | 1385 ± 30 | 1302 | 1275–1344 | |
SacA 29549 | 78–79 | 840 ± 30 | 747 | 686–796 | |
SacA 29550 | 102–103 | 2345 ± 30 | 2354 | 2320–2443 | |
SacA 29551 | 160–161 | 1740 ± 30 | 1653 | 1566–1713 | |
SacA 29552 | 220–221 | 2670 ± 30 | 2775 | 2748–2809 | |
SacA 29553 | 253–254 | Post-modern | Post-1955 | – | |
SacA 29554 | 261–262 | Post-modern | Post-1955 | – | |
Beta 319438 | 268–269 | 170 ± 30 | 180 | 133–228 | |
SacA 29555 | 280–281 | 600 ± 30 | 604 | 542–653 | |
SacA 29556 | 330–331 | 2050 ± 30 | 1995 | 1932–2072 | |
SacA 29557 | 390–391 | 3315 ± 30 | 3535 | 3459–3614 | |
Beta 319439 | 410–411 | 3640 ± 30 | 3952 | 3869–4005 | |
SacA 29558 | 474–475 | 3320 ± 30 | 3545 | 3475–3632 | |
SacA 29559 | 534–535 | 3555 ± 30 | 3840 | 3723–3960 | |
Beta 319440 | 574–575 | 4120 ± 30 | 4620 | 4528–4815 | |
SacA 29560 | 578–579 | 4100 ± 30 | 4612 | 4452–4811 | |
HUB1 (Ucayali) | SacA 26760 | 2–3 | 1535 ± 30 | 1489 | 1358–1522 |
SacA 26761 | 33–34 | Post-modern | Post-1955 | – | |
SacA 26762 | 40–41 | Post-modern | Post-1955 | – | |
SacA 26763 | 44–45 | Post-modern | Post-1955 | – | |
SacA 26764 | 80–81 | Post-modern | Post-1955 | – | |
SacA 26765 | 117–118 | 810 | 719 | 681–770 | |
SacA 26766 | 121–122 | 2840 ± 30 | 2950 | 2886–3037 | |
Beta 305452 | 139–140 | 3020 | 3215 | 3140–3271 | |
MOR1 (Ucayali) | SacA 25002 | 0.5–1.5 | 1330 ± 30 | 1275 | 1184–1208 |
SacA 25003 | 13–14 | 860 ± 30 | 765 | 694–800 | |
SacA 25004 | 19–20 | Post-modern | Post-1955 | – | |
SacA 25005 | 22–23 | Post-modern | Post-1955 | – | |
SacA 25006 | 30–31 | 335 ± 30 | 390 | 309–476 | |
SacA 25007 | 45–46 | 320 ± 30 | 388 | 305–466 | |
SacA25008 | 53–54 | 1045 ± 30 | 954 | 921–1000 | |
SacA 25009 | 70.5–71.5 | 940 ± 30 | 905 | 792–924 | |
SacA 25010 | 80–81 | 670 ± 30 | 660 | 559–676 | |
SacA 25011 | 94–95 | 1160 ± 30 | 1070 | 983–1176 | |
SacA 25012 | 107–108 | 2535 ± 30 | 2724 | 2494–2746 | |
SacA 25013 | 115–116 | 2850 ± 30 | 2961 | 2876–3059 |
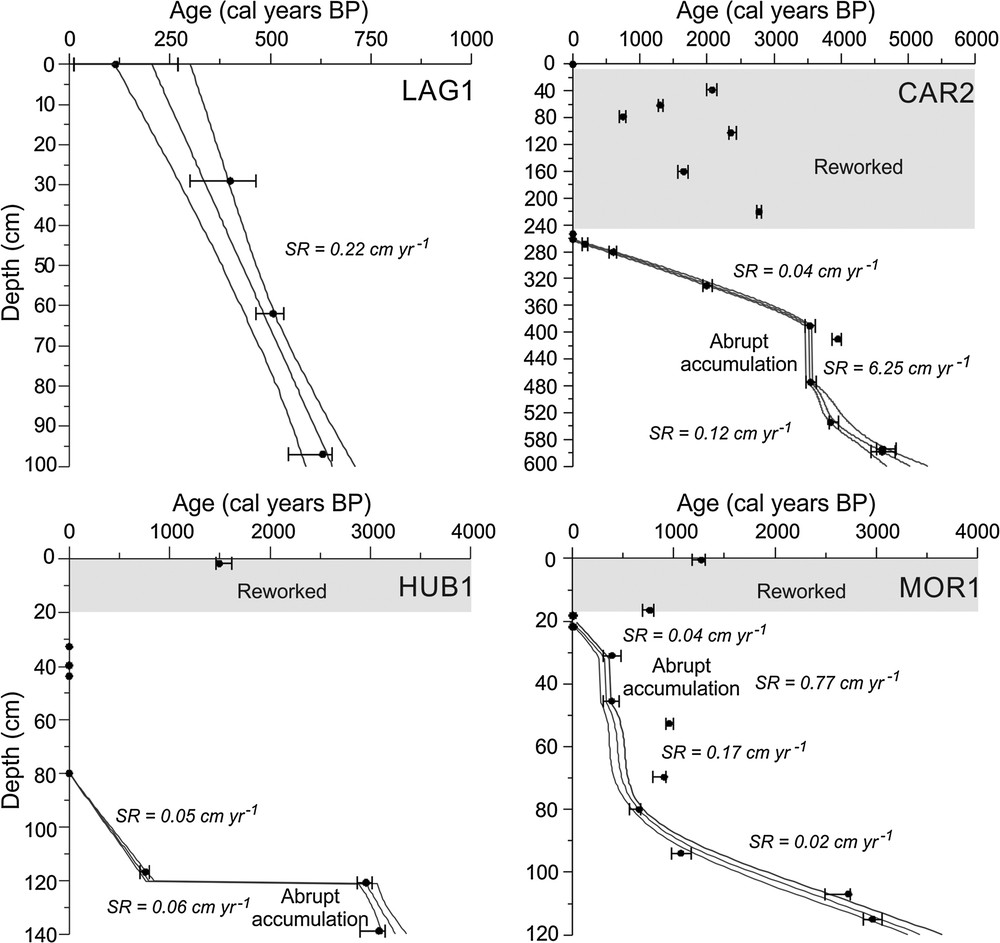
The chronological age models for the four studied cores.
The CAR2, MOR1 and HUB1 cores are comprised of sediments that are poor in organic matter, with a predominance of silt and sand in the CAR2 core and silt and clay in the MOR1 and HUB1 cores (Fig. 3). Nevertheless, low carbon content, organic-rich layers containing plant remains can be identified along the three cores. These layers can be observed:
- • in the CAR2 core between 449–420 cm and 76–56 cm;
- • in the MOR1 core between 81–64 cm, 45–32 cm and 20–0 cm;
- • in the HUB1 at 45–44 cm.
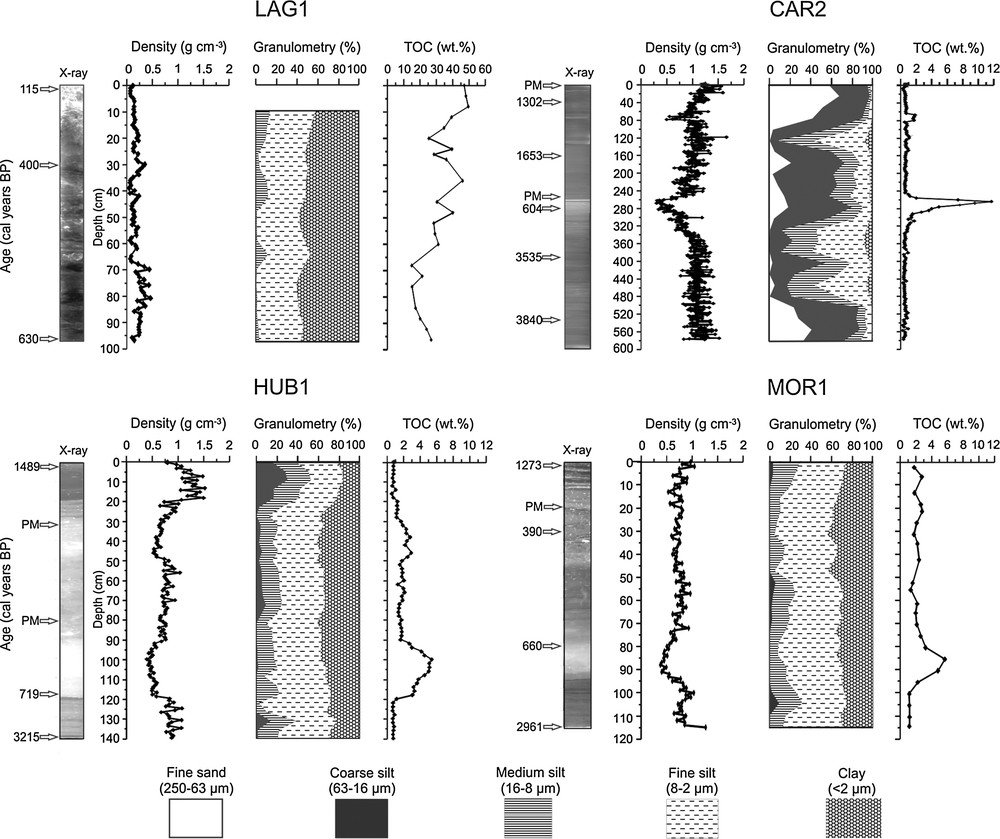
14C data, X-ray images, density, particle size analysis, and total organic carbon for the four studied cores.
The LAG1 core is composed of organic-rich clay with plant remains.
3.2 Bulk density
The sedimentary bulk density in the CAR2, HUB1 and MOR1 cores averages was 1.01 g·cm−3, 0.78 g·cm−3 and 0.73 g·cm−3, respectively, ranging from 0.31 g·cm−3 to 1.65 g·cm−3, 0.40 g·cm−3 to 1.53 g·cm−3 and 0.39 to 1.26 g·cm−3, respectively (Fig. 3). The LAG1 core presented lower bulk density values than the Ucayali cores, with an average of 0.19 g·cm−3, ranging from 0.05 g·cm−3 to 0.45 g·cm−3 (Fig. 3).
3.3 Grain-size fractions
The sediment grain size analysis of the CAR2 core was characterized by a predominance of coarser sediments. Mean values for clay and fine silt are 3% and 20%, respectively, with values for medium silt, coarser silt, and fine sand of 19%, 40% and 18%, respectively (Fig. 3). Analysis of the MOR1 and HUB1 cores showed predominance of clay and fine silt. The mean percentages of clay, fine silt, medium silt and coarse silt, were 33%, 51%, 15% and 1% in MOR1 and 32%, 46%, 17% and 5% in HUB1, without traces of sand (Fig. 3). In the LAG1 core, a predominance of clay and fine silt can be observed, with mean values of 53% and 42%, respectively, and with low values for medium silt, with an average of 5% (Fig. 3).
3.4 Total Organic Carbon
The carbon content of the CAR2 core averaged 1.01 wt%, ranging from 0.25 wt% to 11.7 wt%, with a significant increase between 256 cm and 288 cm (Fig. 3). The HUB1 core had relatively low TOC levels, between 0.56 wt% and 5.38 wt%, with an average of 1.94 wt% (Fig. 3). The MOR1 core had also low TOC levels, between 1.09 and 5.4 wt%, with a mean content of 2.40 wt% (Fig. 3). The LAG1 core TOC values were very high, with mean values of 25.7 wt%, ranging from 8.06 wt% to 50 wt% (Fig. 3).
4 Discussion
4.1 Recent fluvial dynamics of the Ucayali and Marañón Rivers
The Ucayali, Marañón and Beni Rivers are responsible for nearly all of the sediments transported by the Amazon (Guyot et al., 2007). More than 540 million tons of sediments are transported each year in the upstream part of the Amazon River (Filizola and Guyot, 2009; Filizola et al., 2011; Gibbs, 1967; Guyot et al., 2007; Martinez et al., 2009; Meade et al., 1979). The transport and deposition of this large amount of sediment significantly influences the fluvial dynamics of the Amazonian rivers (Constantine et al., 2014). Historical geomorphological data from the Maranon and Ucayali rivers show that the confluence of the two rivers has migrated from SW to NE during the last century (Dumont, 1996). There are few studies dedicated to the recent dynamics of the Ucayali and Marañón Rivers (Dumont, 1994; Quintana, 2015). Through the study of LANDSAT images, Quintana (2015) estimated an average lateral migration of 140 m·yr−1 for the Ucayali River, reaching a peak of 2500 m·yr–1 between 1975 and 2011. In contrast, the Marañón River presented a low migration rate during this period, as shown in Fig. 4. Changes in climate and environmental conditions may trigger a range of alterations in the fluvial system (May et al., 2015).
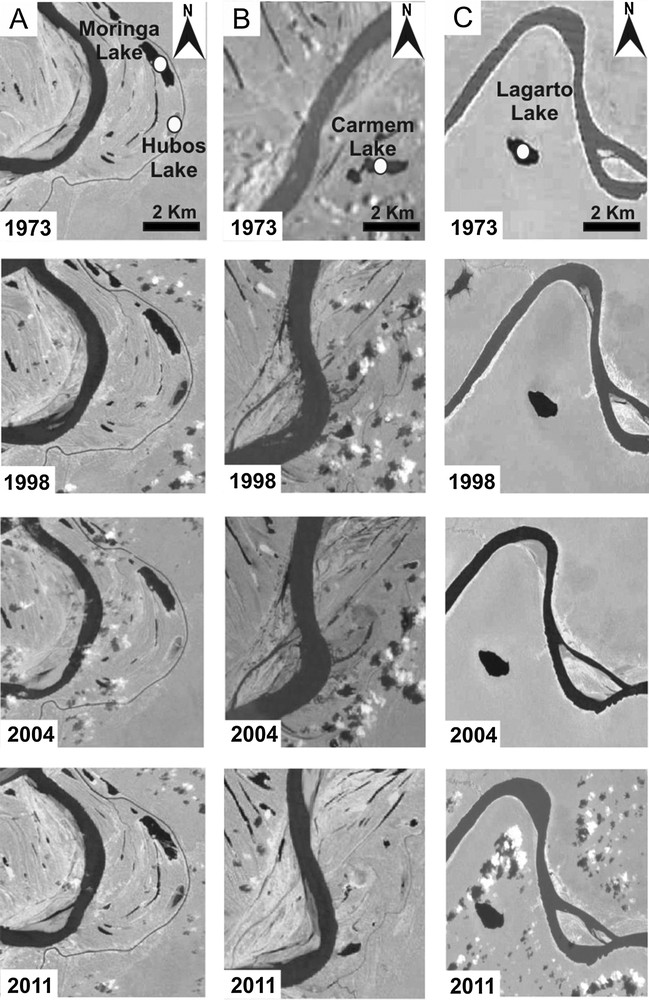
Temporal evolution of meander migration on the Ucayali and Marañón Rivers floodplains, with core location.
4.2 Late Holocene deposition
During the late Holocene, sedimentation in the Ucayali and Marañón floodplain lakes was characterized by different depositional processes. The chronological models obtained from the three sediment cores collected in the Ucayali floodplain lakes show that sedimentation was not gradual and continuous. The CAR2 core, from Lake Carmen, is the only one with expressive sandy sedimentation, mainly at its base and its top, indicating stronger hydrodynamics in this lake. The base of the core is characterized by sandy silt (Fig. 3) deposited between cal 5000 and 3540 ± 80 cal yr BP (0.06 g·cm−2·yr−1). At 3540 ± 80 yr BP, a 90-cm sequence of coarsening-up silts (480–390 cm) settled very quickly. A rapid sedimentation event is also observed at 3000 cal yr BP in the HUB1 core from Lake Hubos (Fig. 2), but it does not present a granulometric trend. Periods characterized by abrupt accumulation (i.e. sediment packages of the same age) were also reported for the late Holocene by Moreira-Turcq et al. (2014), and have been interpreted as rapid deposition of sediment packages that probably correspond to one or several successive events of extreme flooding of the Amazon River. This type of deposition by sediment packages has also been evidenced in modern sediments from the Beni floodplain (Aalto et al., 2003), where they correspond to extreme floods associated with La Niña events. The CAR2 480–390-cm and HUB1 140–120-cm layers also seem to correspond to that kind of sedimentary process, related to overbank deposition and associated with the erosion of inlet channels and levees during very high floods. These flood occurrences are in agreement with a relative humid episode evidenced between 3500 and 2800 cal yr BP in a speleothem from Huagapo Cave, in the central Peruvian Andes (Kanner et al., 2013). After this event, the record of CAR2 shows the deposition of fine silt sediments, with a lower accumulation rate of 0.04 g·cm−2·yr−1, while in HUB1 the sedimentation stopped at 3000 cal yr BP. Both reflect a decrease in river sediment supply to the lakes, which may be due to a reduction in river discharge or, more likely, considering the high dynamics of river migration in the Ucayali floodplain (see Fig. 1), a migration of the main river course away from the lakes. The trend of increasing precipitation recorded in the central Andes between ca 6500 and 2500 cal yr BP (Bird et al., 2011; Kanner et al., 2013; Seltzer et al., 2000; Thompson et al., 1995) reinforced the hypothesis of a change in river position rather than a decrease in river discharge. In Lake La Moringa, core MOR1, the accumulation rate is also low (0.013 g·cm−2·yr−1) between 2960 and 660 cal yr BP, reflecting a weak influence of the river during this period. At 720 ± 45 cal yr BP, deposition resumed in HUB1 with a rate of 0.03 g·cm−2·yr−1, while at 620 ± 60 cal yr BP in MOR1, the accumulation rate increased to 0.09 g·cm−2·yr−1, evidencing renewed influence of river supply, culminating with a 15-cm sediment package at 390 cal yr BP. This deposition increase was probably due to the return of the river's main channel to a closer location to Lakes Hubos and La Moringa, until it reached its present-day position.
In contrast, sedimentation in the LAG1 core from Lagarto Lake in the Marañón floodplain is very different, although this core only reached the last 600 cal yr BP. The core is characterized by fine sediments with high TOC values and a rapid constant sedimentation rate of 0.22 cm·yr−1 from the base to the top of the core. Hydrodynamics are weaker in that lake, which is surrounded by palms and characterized by low-density sediment, resulting in an accumulation rate between 0.01 to 0.09 g·cm−2·yr−1, which is greatly supported by accumulation of organic matter (20% to 50% TOC). Organic sedimentation in Lake Lagarto reflects significant inputs from the local watershed (dominated by Mauritia flexuosa) and probably in situ primary production. Lake Quistococha, in the Iquitos region, also presents an organic sedimentation due to Mauritia flexuosa and algae production during the last 2600 yr cal BP (Aniceto et al., 2014). We estimated the mean river sediment supply to the lake at about 0.06 g·cm−2·yr−1 between 630 and 500 cal yr BP, which however lowered to 0.02 g·cm−2·yr−1 after 400 cal yr BP. This decrease in sediment supply may be due to lateral migration of the river's main channel, although there is no evidence of recent meander migrations in that section of the Marañón River (Fig. 1), or to a decrease in river discharge since 600 cal yr BP. The analysis of Landsat images confirms the relative stability of the Marañón meanders (Dumont, 1996; Quintana, 2015). Lower precipitation is observed in the Andes since 1600 AD (Apaéstegui et al., 2014; Bird et al., 2011; Kanner et al., 2013; Thompson et al., 1995) and would explain the reduced discharge of the Marañón River during that period.
4.3 Extreme events deposition
Channel migration is a natural process that remobilizes old alluvial sediments, which mix with modern sediments transported by the river from actively eroding hill slopes (Wittmann et al., 2011). Our data show that sediment stratigraphy in Ucayali floodplain lakes over the past 4000 years is characterized by the presence of packages of very high sedimentation rates (abrupt accumulation) in the three Ucayali cores (Fig. 2). The very short duration of this deposition is below the 14C resolution, and the age of these packages varies between 400 and 3500 cal yr BP. These packages are interpreted as the rapid deposition of sediment that probably corresponds to one or several successive events of extreme flooding of the Ucayali River (Moreira-Turcq et al., 2014). These are characterized by the presence of older 14C age values in the sediment layer, which are interpreted as a contribution of older reworked alluvium. We also observed reworked sediments in the top unit of the Ucayali floodplain cores (Fig. 2), whose ages vary between 600 and 2800 yr BP, deposited upon lake sediment younger than 1950 AD (post-bomb). These sediment packages have a thickness of 250, 30, and 15 cm in Lakes Carmen, Hubos, and La Moringa, respectively. These packages were thicker in the lake nearest the river main channel, as observed in the Carmen sedimentary records. They record a modern period of very high floods that reworked past alluvial sediments of the Ucayali floodplain.
In the case of Lake Carmen, this modern deposition is directly linked to the invasion of the lake by a meander of the Ucayali River (Fig. 4). Due to lateral meander migration, the river levee migrated into Carmen Lake and rebuilt itself over past lacustrine deposits. Based on several satellite images (Fig. 4), we can determine that, in 2004, the river levee reached the lake and cut almost half of its surface. In the case of Lakes La Moringa and Hubos, however, the river remains farther away, and the modern deposition of reworked sediments implies an occurrence of extreme flooding during the past 60 years, at most.
Extreme hydroclimatic events can multiply the effects of the natural channel migration process, and Carmen Lake modern deposition, related to meander migration, may also be a consequence of an increase in flood magnitude. This migration began in 1989, after a stability of several millennia. The river had migrated to the south 3500 years ago, leaving the proximity of Lake Carmen, but never went north of its position of 1973, as shown by the pattern of older paleochannels on the left bank of the river (Fig. 4). If the resumption of meander migration is linked to the extreme floods registered in Lakes Hubos and La Moringa, it means that these extreme events occurred after 1989. Based on hydrography data, Espinoza et al. (2012) have shown an increase in flood levels in Amazonian rivers after 1980, probably due to current anthropic-driven climate change. Our data confirm that floods from the last 60 years, and probably from the last decade (Espinoza et al., 2009, 2011, 2012), have had a higher impact, in term of alluvial sediment reworking, than any other floods in the past 3000 years.
5 Conclusion
The change in sedimentation of floodplain lakes near the Marañón and Ucayali River confluence during the late Holocene may be related to the combined effects of geomorphological fluvial processes of channel migration and palaeoclimatic changes during the late Holocene. The sediment accumulation rate appears to be a good proxy for river supply for these lakes, which have a limited watershed. The lower deposition rate in Ucayali floodplain lakes since 3540 cal yr BP is interpreted as being related to the changing course of the Ucayali River, which affected the three Ucayali lakes. Events of rapid sediment deposition at 3000 and 3540 cal yr BP, however, reflect extreme floods that occurred during a period of higher precipitation in the central Andes. In the Marañón River Lake, the decrease of river influence since 400 cal yr BP corresponds well with the decrease in precipitation in the Andes.
The modern deposition of reworked sediments, observed in the three Ucayali floodplain lakes, indicates a resumption of meander migration and a strengthening of extreme floods that could be related to present-day climate change.
Acknowledgments
This research was supported by the French Research Institute for Development (IRD) through the SO-Hybam Research Program's cooperation agreement with the Federal Fluminense University (UFF), Brazil. This study was also supported by the INSU Paleo2–PASCAL project (Past Climate Change Impacts on Carbon Accumulation in Amazonia Floodplain Lakes (2010–2012)). The authors would like to thank the technical groups of IRD (ALYSES, Bondy) for their help during the laboratory work. We also thank the SENAMHI-Iquitos group for assistance during core collection. I. Quintana's work is supported by a fellowship from CAPES, Brazil.