1 Introduction
Our understanding of the driving forces of carbon fluxes in the Amazon River needs to be improved to better explain CO2 outgassing pathways (Abril et al., 2014; Richey et al., 2002). Stable isotope data are powerful tools to constrain the sources of respired carbon from upland soils, flooded forest C3 plants, macrophyte C4 plants, and phytoplankton, but the majority of δ13C data were measured on either dissolved inorganic carbon (DIC) or particulate organic carbon (POC) (Ellis et al., 2012; Mayorga et al., 2005; Moreira-Turcq et al., 2013; Mortillaro et al., 2011; Quay et al., 1992). In existing data, δ13C-DOC values are generally slightly lower than δ13C-POC values, and natural variation seemed limited (Aufdenkampe et al., 2007; Ellis et al., 2012; Hedges et al., 1994; Mayorga et al., 2005). Limited natural variations in δ13C-DOC compared to δ13C-POC may be consistent with a limited contribution of in situ aquatic primary production and the advanced degradation status of the river-transported dissolved organic matter pool (Hedges et al., 1994; Quay et al., 1992), which is also generally more recent in origin (Marwick et al., 2015; Mayorga et al., 2005). The aim of this study was to test if the variability in natural DOC sources is not underestimated due to the relative scarcity of DOC stable isotope data for the middle Amazon Basin reach, with the risk to incorrectly identify the carbon flux sources at the level of water–mass exchanges in the floodplain or to underestimate specific (production/transformation) mechanisms. Thus, particular efforts were made to document the spatial and temporal variation of DOC concentrations and δ13C-DOC in the central Amazon River, to explore the links between the mainstem, tributaries, and the floodplain. We used a detailed spatial and temporal sampling strategy to describe how DOC from various potential sources might be transported in the river in relation with the flood pulse. We also take advantage of a river section located along a floodplain gradient to document potential contrasts between upland soils, C3 and C4 wetland sources, and phytoplankton.
2 Material and methods
2.1 Field campaign and sampling
Water samples were collected on a ∼800-km transect along the lower Amazon River basin from Manacapuru on the Solimões River, to Santarem at the mouth of the Tapajós River, located in a gradient of decreasing flooded forest area and increasing open lake area (Fig. 1). The three characteristic types of water found in the Amazon (Sioli, 1984) were sampled in the mainstem and tributaries: the “white waters” characterized by high levels of suspended sediments, high nutrient concentrations, and high conductivity in the Solimões and Madeira rivers, the two main tributaries of the Amazon River flowing from the Andes; the “black waters” with low conductivities in the Manacapuru and Negro rivers draining the lowest Amazonian forest dominated by podzol soils and intensely colored by humic organic matter; the organic poor “clear waters” with low suspended sediment concentrations, and low conductivity and nutrient concentrations in the Tapajós, Urubu, and Trombetas. We also sampled five floodplains along the gradient from flooded forest to open lakes. Upstream, the Várzea of Cabaliana and Janauacá receive large inputs of white waters from the Solimões River and are surrounded by large areas of flooded forest (Fig. 1). In the middle region of the Amazon River, we sampled two floodplain lakes just downstream of the Amazon–Madeira confluence (Fig. 1), Lakes Canaçari and Miratuba, mainly receiving white water from the flooding of the Amazon and Madeira Rivers. Lake Miratuba, receiving Madeira's waters from the south through a network of small channels, is surrounded by large flooded forests and dries up substantially (a 70% decrease in surface area) during the low waters. This is not the case in Lake Canaçari, which is a very homogeneous lake, connected to small flooded forests and which does not dry up by more than 20% at low water (Fig. 1). Canaçari mainly receives white waters from the Amazon, as the clear waters from the Urubu River that drains the northern local basin are by-passed to the north and rarely enter the lake. Finally, in the most downstream part of the studied area (Fig. 1), the Várzea of Curuaí is composed of more than 30 interconnected lakes of mostly white water temporally or permanently connected to the Amazon mainstem by small channels (Bonnet et al., 2008; Hess et al., 2003). Moreover, the Várzea of Curuaí is not strongly connected to flooded forests, most of the floodplain consisting of open lakes (Fig. 1).
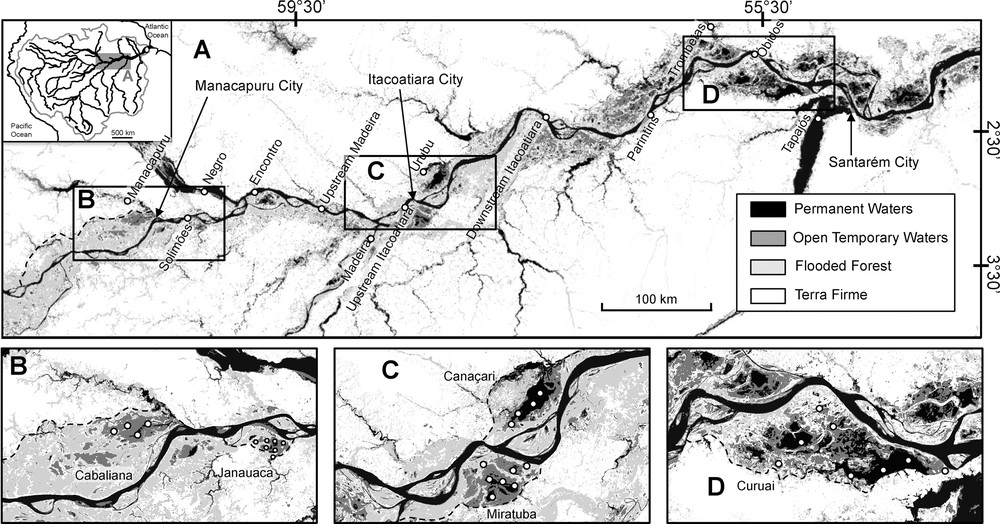
Study area showing the Amazon Basin mainstem, the tributaries and floodplain lakes, the sampling stations, and the localities cited in the text.
Sampling for DOC and δ13C-DOC was performed during five cruises at different stages of the hydrograph (Fig. 2). The low-water (LW) season was sampled in October 2008 and October 2009, the rising water (RW) season was sampled in January–February 2011, the high-water (HW) season in June–July 2009, and the falling water (FW) season in August–September 2010. During 2009, the lowest water stage was delayed, thus the October cruise sampled the second part of the falling period. For the more downstream stations, the end of this period shows water levels equivalent to the 2008 LW period (Fig. 2). Concerning the data used in this study (DOC concentrations and δ13C-DOC values), the values measured in October 2009 were in good agreement with those found in October 2008 (see Fig. 3), and are different from those found during the FW period in August–September 2010. We sampled the major tributaries (Solimões, Negro, Madeira, and Tapajós), and six stations in the Amazon mainstem during the four seasons (Fig. 2), whereas the Manacapuru, Urubu and Trombetas Rivers were sampled only occasionally (Table 1). In each floodplain lake, as a function of the water level, we sampled 3 to 6 stations. Dense phytoplankton blooms are known to occur in some of these floodplain lakes (Moreira-Turcq et al., 2013); we therefore investigated whether diurnal variations in DOC and δ13C-DOC occur by sampling during 24 h at a frequency of 1 to 4 hours. 24-h sampling was performed in Lake Janauaca during HW and FW, and in Lake Canaçari during FW. In addition, we performed sampling along three vertical profiles in the floodplain lakes during HW conditions to document potential effects of water column stratification. Finally, during the RW cruise, a set of specific samples was taken for DOC, DIC, δ13C-DOC and δ13C-DIC analysis. The sampling took place in the southern part of Lake Canaçari (Fig. 1) from the open water area into a decaying and sulphide-rich plant raft known as “capim”. During this period, the water depth under the plant raft was about 5 m, as in the open lake area. These floating meadows are mainly composed of C4 aquatic macrophytes with δ13C values around –13‰, but to a lesser extant of C3 plants that have δ13C values ranging from −28 to −35‰ (Moreira-Turcq et al., 2013; Mortillaro et al., 2011).
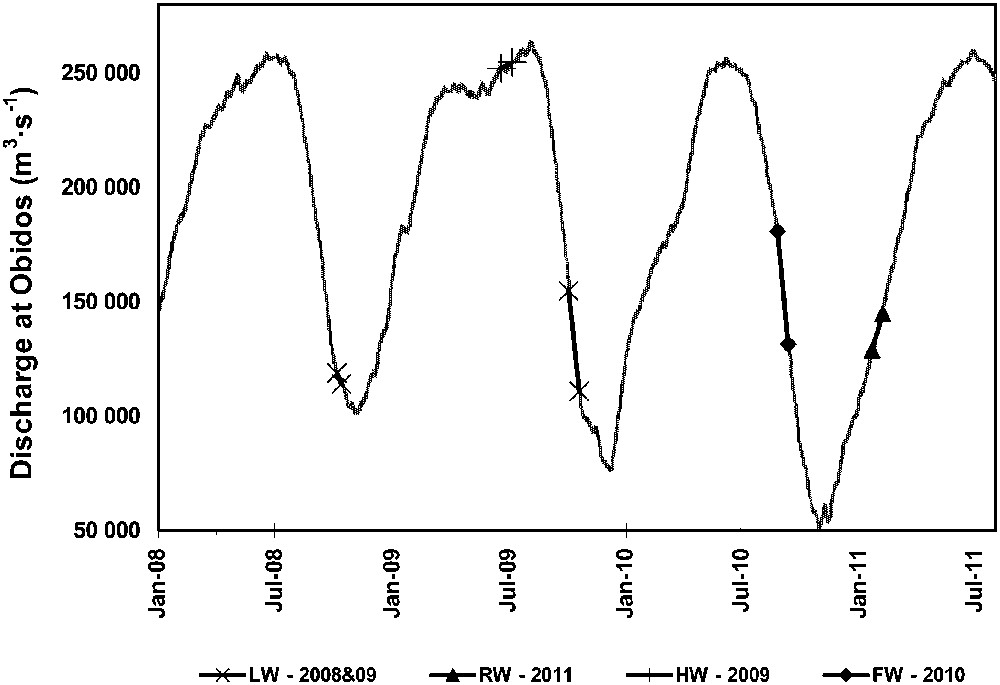
Distribution of the five CARBAMA cruises during which data were collected, along with the Amazon hydrograph at Óbidos during years 2008–2011 (start and end plotted for each cruise).

DOC (a) and δ13C-DOC (b) values for CARMAMA cruises (October 2008 to January 2011) from upstream to downstream Amazon mainstem and tributary stations according to the hydrographical regime. Analytical standard deviation error bars for triplicates are smaller than plot mark size. For the low-water periods (2008 and 2009), the mean and SD values were plotted, except for the Tapajós and the Amazon upstream of the confluence with the Madeira (only 2009 data). The Tapajós had a very low δ13C-DOC value (−34.6‰) during high waters, which falls outside the Y-axis range.
Mainstem and tributaries 2008–2011. DOC average concentrations (mg·L−1) and δ13C-DOC mean values (‰ versus VPDB).
Site | Water color Cond./pH/TSS (μS·cm−1/–/mg·L−1) |
Season | DOC (mg·L−1) | δ13C (‰) | n |
Amazon mainstem | |||||
Encontro to Obidos | White/57/6.7/39 | LW | 3.5 ± 0.2 | –28.9 ± 0.2 | 11 |
White/70/6.8/146 | RW | 3.4 ± 0.2 | –29.1 ± 0.3 | 6 | |
White/52/6.3/22 | HW | 4.4 ± 0.2 | –29.7 ± 0.4 | 6 | |
White/53/6.8/36 | FW | 4.8 ± 1.3 | –29.3 ± 0.9 | 6 | |
All | 3.9 ± 0.9 | –29.2 ± 0.6 | 29 | ||
Encontro | White/63/6.7/20–150 | All | 3.7 ± 0.5 | –29.0 ± 0.5 | 5 |
Upstream Madeira | White/66/6.6/20–140 | All | 3.9 ± 0.4 | –29.0 ± 0.4 | 4 |
Upstream Itacoatiara | White/58/6.7/28–133 | All | 3.5 ± 0.5 | –29.0 ± 0.3 | 5 |
Downstream Itacoatiara | White/56/6.7/20–136 | All | 4.0 ± 0.9 | –29.5 ± 0.6 | 5 |
Parintins | White/54/6.7/25–131 | All | 4.1 ± 1.2 | –29.5 ± 0.4 | 5 |
Obidos | White/53/6.7/25–137 | All | 4.1 ± 1.2 | –29.2 ± 0.8 | 5 |
Tributaries | |||||
Solimões | White/75/6.8/25–184 | All | 3.0 ± 0.6 | –28.9 ± 0.1 | 5 |
Madeira | White/65/6.8/24–353 | All | 2.5 ± 0.9 | –27.8 ± 0.7 | 5 |
Negro | Black/10/4.9/8 | All | 6.7 ± 0.9 | –29.3 ± 0.1 | 5 |
Manacapuru | Black/12/5.3/6 | All | 5.4 ± 0.7 | –29.8 ± 0.4 | 4 |
Urubu | Clear/15/5.9/8 | All | 4.1 ± 0.9 | –29.6 ± 0.6 | 4 |
Trombetas | Clear/14/5.3/4 | HW | 3.8 | –30.1 | 1 |
Tapajós | Clear/18/6.6/4 | All | 3.0 ± 1.0 | –30.5 ± 2.5 | 4 |
All water samples were taken from a 25-m vessel board with a modified Niskin bottle sampler or manually from a smaller boat (Abril et al., 2014).
Samples for δ13C-DOC were filtered in the field through precombusted GF/F glass fiber filters (0.7 μm), either under vacuum or by use of syringes and acidified to pH 1–2 with Suprapur® phosphoric acid.
Samples for δ13C-DIC were collected using 120-mL glasses serum bottles sealed with a rubber stopper and poisoned with 0.3 mL of HgCl2 at 20 g·L−1 to avoid any microbial respiration during storage. Vials were carefully sealed, taking care that no air remained in contact with the samples, and stored in the dark to prevent photo-oxidation. In the case of the capim transect, additional samples of surface water were sampled manually with a funnel, then filtered trough a GF/F mini-filter tipped on syringes, poisoned with one drop of a saturated HgCl2 solution and stored in headspace-free 7-mL Exetainer® vials.
2.2 DOC and δ13C-DOC analysis
DOC and δ13C-DOC values (2009 to 2011) were obtained by a wet oxidation method (Albéric, 2011) using a commercially available liquid chromatography (LC) interface (LC-Isolink, Thermo Scientific) coupled with a continuous flow isotope ratio mass spectrometer (IRMS, Delta V, Thermo Scientific). Briefly, for the 1–10 mg·L−1 DOC range, 100 μL of He purged water sample were injected in-line via the LC-interface. Mixing of the MilliQ water mobile phase with reactants (H3PO4 1.5 M; Na2S2O8 0.4 M) is performed via the LC-Isolink interface and DOC is oxidized into CO2 around 100 °C and then transferred by the carrier gas (He) to the ion source of the IRMS. The analysis in triplicates took ∼10 min; the repeatability and accuracy were estimated to be within the range of 0.1 and 0.3‰, respectively (Albéric, 2011).
The samples taken in October 2008 were analyzed using a customized wet oxidation analyzer (Thermo HiperTOC) coupled with a Thermo Delta +XL IRMS (Bouillon et al., 2006).
2.3 DIC and δ 13C-DIC analysis
We calculated DIC from pCO2, total alkalinity (TA), and temperature measurements using the carbonic acid dissociation constants of Millero (1979) and the CO2 solubility from Weiss (1974), as implemented in the CO2SYS program. pCO2 was measured continuously with an equilibrator connected to an infrared gas analyzer (Abril et al., 2014). TA was analyzed by automated electro-titration on 50-mL filtered samples with 0.1 NHCl as titrant. The equivalence point was determined with a Gran method from pH between 4 and 3. The precision based on replicate analyses was better than ± 5 μM (Abril et al., 2015).
The δ13C-DIC was measured following the procedure of Gillikin and Bouillon (2007). The measurements were performed using an Isotope Ratio Mass Spectrometer (Micromass Isoprime), equipped with a manual gas injection port. To correct for the partitioning of CO2 between the headspace and the water phase in the samples, and to calculate the δ13C of the total DIC, the isotopic fractionation of CO2 at the water–air interface as a function of the lab temperature of Miyajima et al. (1995) was applied.
In the case of the capim transect additional samples, DIC and δ13C-DIC values were obtained by the same method as above, for DOC and δ13C-DOC, but by manual injection, pushing 10 μL of the Exetenair content through the injector and without heating the LC-Isolink interface reactor (Brandes, 2009). δ13C-DIC data obtained with this second method were discarded because of obvious isotope exchanges during sampling in some of the small volume vials. Hence, only the δ13C-DIC values acquired by the first method (120 mL samples) were retained in this study.
3 Results
3.1 Amazon mainstem and tributaries
DOC concentrations in the Amazon mainstem averaged 3.9 ± 0.9 mg·L−1 (Table 1). Higher concentrations were found in the Rio Negro and Rio Manacapuru black waters (6.7 ± 0.9 and 5.4 ± 0.7 mg·L−1, respectively). The lowest concentrations were found in samples from Rio Solimões and Rio Madeira (3.0 ± 0.6 and 2.5 ± 0.9 mg·L−1, respectively) and the clear waters of the Tapajós (3.0 ± 1.0 mg·L−1). Concentrations in the mainstem were the highest in the lower reach and during FW (Table 1; Fig. 3a).
The average δ13C-DOC values (−29.2 ± 0.6‰, Table 1) did not vary significantly in the mainstem and tributaries between Manaus and Óbidos-Santarém irrespectively of the type of water, except for the Rio Madeira that showed higher δ13C-DOC values (−27.8 ± 0.7‰) (Table 1 and Fig. 3b). A different pattern is noticed when looking at seasonal variability: upstream of the confluence of the Rio Solimões and the Rio Negro (Encontro das Aguas), the δ13C-DOC values of both rivers were also relatively constant throughout the hydrological cycle (Fig. 3b). The mean δ13C-DOC values were –28.9 and –29.3 ± 0.1‰ for the Rio Solimões and the Rio Negro waters, respectively (Table 1). In contrast, in the most downstream part of the studied river section, a large seasonal variability was observed, with a range of up to 2 ‰ at Óbidos (Table 1 and Fig. 3b). In the Amazon mainstem, an opposite trend occurred upstream and downstream of Itacoatiara (Fig. 3b). Upstream of Itacoatiara, δ13C-DOC values higher than the mean value for each station were measured during FW; they were similar to those measured in the Rio Madeira. In contrast, downstream of Itacoatiara, during the same FW period, lower δ13C-DOC values were found as well as during HW. These low δ13C-DOC values coincided during FW with higher DOC concentrations (Fig. 3a). In addition, the Madeira waters were characterized by δ13C-DOC values higher than any values reported for other stations at every period. The largest variation was observed in the Rio Tapajós clear waters between –34.6‰ during HW and –28.0‰ during LW.
3.2 Floodplain lakes
The results for the floodplain lakes are summarized in Fig. 4 and Table 2. Within each lake, 3 to 8 different sites were sampled, leading to a larger spatial variability in DOC and δ13C-DOC when compared to riverine sites. In contrast, diurnal and vertical profile variabilities were found to be small, with δ13C-DOC ranging from 0.2 to 0.7‰ (Table 2). DOC concentrations were generally higher than in the main channels. While the highest concentration was found during RW in the upper reach lake (Cabaliana), small seasonal variations are reported in the other lakes surrounded by large flooded forests (Janauaca and Miratuba). In contrast, higher concentrations were measured during FW in the lakes the least connected to the flooded forest (Canaçari and Curuaí). Concerning δ13C-DOC, three principal observations can be made: (1) despite the wider range in δ13C-DOC, the average value found in floodplain lakes (with the exception of Lago Miratuba) was similar to the mean value measured for mainstems, i.e. –29.2 ± 0.3‰; (2) during all seasons, the δ13C-DOC values were higher in Lago Miratuba compared to the values for the other lakes. With an average value of −27.8 ± 0.4‰, δ13C-DOC values in Lago Miratuba resemble the one measured in the Rio Madeira. (3) Maximal seasonal variability was found at the more downstream locations, i.e. for the Várzea of Curuaí, where the highest δ13C-DOC values were found during LW and the lowest values during HW, which is similar to what was observed in the Tapajós and to a lesser extant in the main channel at Obidos (Figs. 3b and 4b).
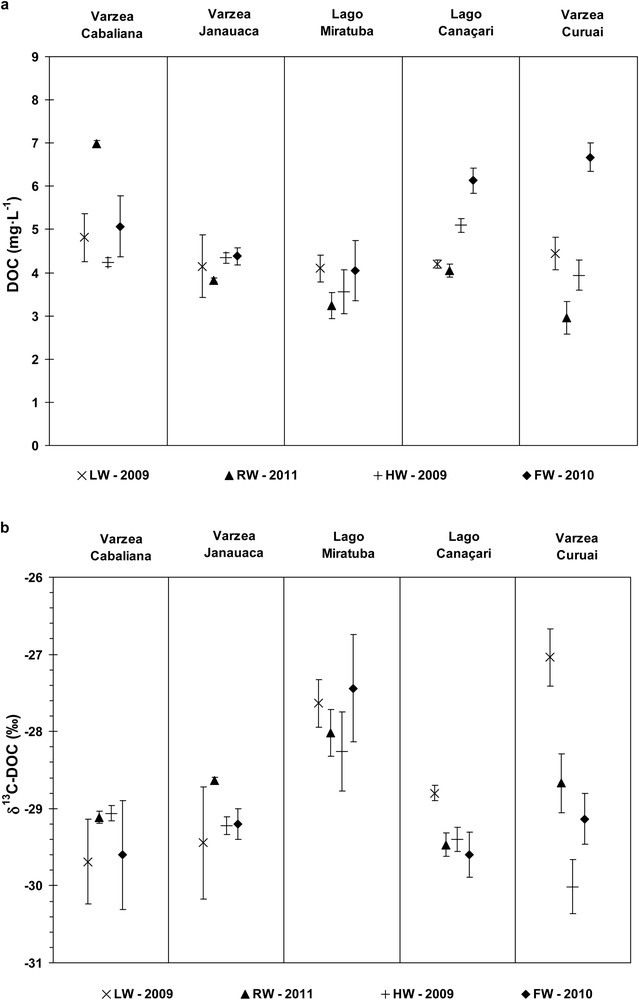
DOC (a) and δ13C-DOC (b) values for CARBAMA cruises (October 2008 to January 2011) from upstream to downstream floodplain lakes.
DOC concentrations (mg·L−1) and δ13C-DOC values in floodplain lakes (‰ versus VPDB).
Locations | Cond./pH/TSS (μS·cm−1/–/mg·L−1) | Season | DOC (mg·L−1) | δ13C (‰) | n |
Várzea Cabaliana | |||||
75/6.9/10 | LW | 4.8 ± 0.1 | –29.7 ± 0.6 | 4 | |
120/7.0/14 | RW | 7.0 ± 0.4 | –29.1 ± 0.1 | 2 | |
74/6.5/4 | HW | 4.2 ± 0.1 | –29.1 ± 0.1 | 5 | |
69/6.7/6 | FW | 5.1 ± 0.7 | –29.6 ± 0.7 | 3 | |
Várzea Janauacá | |||||
52/7.1/15 | LW | 4.1 ± 0.5 | –29.4 ± 0.7 | 7 | |
60/6.6/75 | RW | 3.8 ± 0.5 | –28.6 ± 0.1 | 3 | |
41/6.2/6 | HW | 4.3 ± 0.1 | –29.2 ± 0.1 | 5 | |
60/6.7/6 | FW | 4.4 ± 0.3 | –29.2 ± 0.2 | 5 | |
Daily variationa | HW | 4.4 ± 0.2 | –29.6 ± 0.2 | 27 | |
Daily variationb | FW | 4.8 ± 1.0 | –29.2 ± 0.7 | 7 | |
Depth profile (0.5–10 m) | HW | 4.4 ± 0.2 | –29.8 ± 0.2 | 6 | |
Depth profile (0.5–10 m)c | HW | 4.2 ± 0.1 | –29.1 ± 0.2 | 6 | |
Lago Miratuba | |||||
56/6.8/65 | LW | 4.1 ± 0.3 | –27.6 ± 0.3 | 3 | |
52/–/28 | RW | 3.2 ± 0.1 | –28.0 ± 0.3 | 2 | |
47/6.3/26 | HW | 3.6 ± 0.1 | –28.3 ± 0.5 | 5 | |
61/7.6/29 | FW | 4.0 ± 0.8 | –27.4 ± 0.7 | 5 | |
Lago Canaçari | |||||
44/7.9/23 | LW | 4.2 ± 0.1 | –28.8 ± 0.1 | 2 | |
13/6.2/19 | RW | 4.0 ± 1.4 | –29.5 ± 0.2 | 3 | |
46/6.4/11 | HW | 5.1 ± 0.2 | –29.4 ± 0.2 | 4 | |
53/–/11 | FW | 6.1 ± 0.8 | –29.6 ± 0.3 | 3 | |
Daily variationb | FW | 6.5 ± 0.7 | –28.8 ± 0.3 | 7 | |
Várzea Curuaí | |||||
42/8.1/51 | LW | 4.4 ± 0.7 | –27.0 ± 0.4 | 5 | |
59/6.4/22 | RW | 3.0 ± 0.3 | –28.7 ± 0.4 | 4 | |
45/6.5/15 | HW | 3.9 ± 0.1 | –30.0 ± 0.3 | 8 | |
57/9.5/60 | FW | 6.7 ± 0.5 | –29.1 ± 0.3 | 3 | |
Depth profile (0.5–2 m) | HW | 4.1 ± 0.1 | –30.3 ± 0.5 | 3 |
a Sampled every hour at a depth of 0.5 m at a single location.
b Sampled every 4 h at a depth of 0.5 m at a single location.
c Profile in the northern part of the várzea.
3.3 Capim transect Lago Canaçari
The concentrations of DOC approximately doubled when going from open waters into the plant raft, while δ13C-DOC values increased from –30‰ to –28.5‰ (Fig. 5a). The trend line in the δ13C-DOC versus 1/DOC plot (insert Fig. 5a) points to a value around −27‰ for the added carbon, clearly not corresponding to a C4 plant source.

Variation of δ13C-DOC values and DOC concentrations (a) and δ13C-DIC values and DIC concentrations (b) from inside a C4 plant raft to open water in Lago Canaçari (RW, January 2011). A δ13C-DOC versus 1/DOC plot for the samples closest to the plant raft is inserted in Fig. 5a. DIC concentrations are from two sampling sets; one analyzed by a method adapted from Brandes (2009) in black squares and the other (3 data, open diamonds) by the regular method used during this program for 13C-DIC analysis (see section 2). Analytical errors are comprised within the symbols.
Along the same transect, the concentrations of DIC increased by a factor of 10 (from 2 to 20 mg·L−1), while the δ13C-DIC values increased from –13.3‰ (pH 6.5) to –10.7‰ (pH 6.3) (Fig. 5b). δ13C-DIC values measured in the mainstem during this period (detailed results not shown) typically ranged around –13.8 ± 0.1‰ (pH 6.9), which is consistent with previous data from this region (Quay et al., 1992).
4 Discussion
4.1 Comparison with previous δ13C-DOC and δ13C-POC data in the Amazon River basin
Since the work of Cai et al. (1988), it has been well recognized (Aufdenkampe et al., 2007; Hedges et al., 2000; Mayorga et al., 2005; Quay et al., 1992) that POC in the upper reaches of the Amazon River has less negative δ13C values than in the central and lower reaches. The mechanism behind that general down-river trend has been proposed to be the smaller degree of isotope fractionation during photosynthesis by the C3 plants at the lower pCO2 found in altitude. Our average δ13C-DOC values (–29.3 ± 0.6‰) for the lower Amazon were in the same range as those reported in previous studies (Mayorga et al., 2005; Pérez et al., 2011; Quay et al., 1992). In particular, the less negative values we report for the Rio Madeira agree well with the high-water stage value (–28.0‰) given by Hedges et al. (1994) in the same predominantly upland-draining river (Quay et al., 1992). The small variability of δ13C-DOC values we measured during the hydrological cycle for the Rio Negro and the Rio Solimões at the entry of the studied river section suggests relatively constant and homogeneous DOC sources from upland soils and flooded forests, in agreement with the degraded pattern of the dissolved organic matter which has been reported in the lowland Amazon reach (Hedges et al., 1994, 2000).
The seasonal variability for δ13C values of organic C-species are less documented. The data from our study show a clear seasonal trend, with lower δ13C-DOC values during HW and FW downstream of Itacoatiara. A similar trend was observed, in this area, with lower δ13C-POC values during the HW period than during other periods (Moreira-Turcq et al., 2013). Moreover, in the lowland main channel, Quay et al. (1992) found lower δ13C-POC values (for the fine POC fraction) during the FW period, yet did not observe any clear seasonal variation for δ13C-DOC. These lower δ13C-POC values were interpreted as an increased contribution of C3 sources from tributaries draining lowland regions and from floodplain soils and vegetation.
In contrast, we report higher δ13C-DOC values in the Madeira River, the main channel upstream of Itacoatiara during FW and at the lower reach stations during LW (Figs. 3b and 4b). While the persistence of the upland effect due to a weaker density of flooded forest compared to the Solimões Basin may explain the higher δ13C-DOC values found in the Madeira River and the associated lake (Lago Miratuba), the question arises whether a larger influence of C4-grasses can explain the higher δ13C-DOC values we observed in the lower reach during LW. Although decaying matter in these massive plant rafts was found to increase δ13C-DOC values locally by 1.5‰, reaching –28.5‰ (Fig. 5a), the raise appears poorly linked to C4 plants, since δ13C-DOC values, in microcosm experiments in which C4 plants sorted out from these mats were incubated, reached –24‰ to –17‰, depending on the amount of macrophyte biomasses used (Mortillaro et al., 2016). In contrast, the impact of decaying plant material in floating meadows on DIC concentrations was found to be very strong, with an almost 10-fold increase in DIC concentration, while δ13C-DIC values raised to –10.7‰ (Fig. 5b), a value clearly higher than that in open lake and mainstem waters at pH 6.3 (Quay et al., 1992), which may likely correspond to the respiration input of C4 plant carbon. We therefore hypothesize that most of the organic matter produced by these macrophyte mats is decayed in situ, and that its effect on the DOC pool in lake waters is relatively small. This observation agrees with previous results showing the small contribution of C4 macrophytes to particulate matter and food-web in the lake and mainstem waters despite their high biomasses in the ecosystems (Moreira-Turcq et al., 2013; Mortillaro et al., 2011, 2016; Quay et al., 1992).
4.2 New insights from variations during the runoff cycle
The data in this work point to a contrasting seasonality of δ13C-DOC values in the main Amazon channel between the reaches upstream and downstream of the confluence with the Rio Madeira (from upstream to downstream of Itacoatiara). Apart the specific impact of the Rio Madeira waters, the upstream reach is characterized by values clustering around the whole lowland Amazon average value (i.e. –29‰), while the downstream reach is characterized by lower δ13C-DOC values during HW and FW and, to a lesser extent by higher values during LW. The lower δ13C-DOC values during FW coincided with an increase in DOC concentrations in the main channel (Fig. 3b). Fig. 6 illustrates the upstream versus downstream trend between DOC concentrations and δ13C-DOC during the FW period. We hypothesize higher planktonic influence from flooded lakes downstream of Itacoatiara during HW and FW. More 13C-depleted values found in Curuaí water during HW (Fig. 3b) support our hypothesis although not much variation was observed in Lago Canaçari. As indicated by Archer (2005), significant differences in floodplain characteristics occur at the confluence of the Rio Madeira with the Rio Amazonas. Upstream of the Rio Madeira mouth, there are relatively few lakes in the floodplain, while downstream of the Madeira, the area is characterized by large meanders and secondary channels with incomplete levees resulting in inundation of large floodplain lakes. The channel-floodplain geomorphology and valley width differences on either side of Itacoatiara are shown in Fig. 7a, based on the data of Mertes et al. (1996, their Fig. 2A) and Dunne et al. (1998, their Fig. 3A). We hypothesize that the larger floodplain lake area downstream Itacoatiara accounts for the lower δ13C-DOC values during the HW and FW periods (Fig. 7b). The high phytoplankton biomass found in these larger floodplain lakes, with likely low δ13C values (–33‰ to –37‰), as suggested by Ellis et al. (2012), may be the source explaining the trend observed in the mainstem during these periods of large export of organic matter from the floodplain to the river main channel (Moreira-Turcq et al., 2013). However, the δ13C values of plankton sources are difficult to constrain over larger scales, since in the same studied area, values ranging from –23 to –40‰ during LW, –31 to –43‰ during HW (Mortillaro et al., 2011), and reaching up to –18‰ during LW dense (cyano)-phytoplankton blooms (Moreira-Turcq et al., 2013) have been reported. The large variation in δ13C-DOC values observed in the Tapajós clear waters, which matches that reported for δ13C-POC values in the same river (Mortillaro et al., 2011), may be also linked to seasonal differences in phytoplankton nature and biomass.
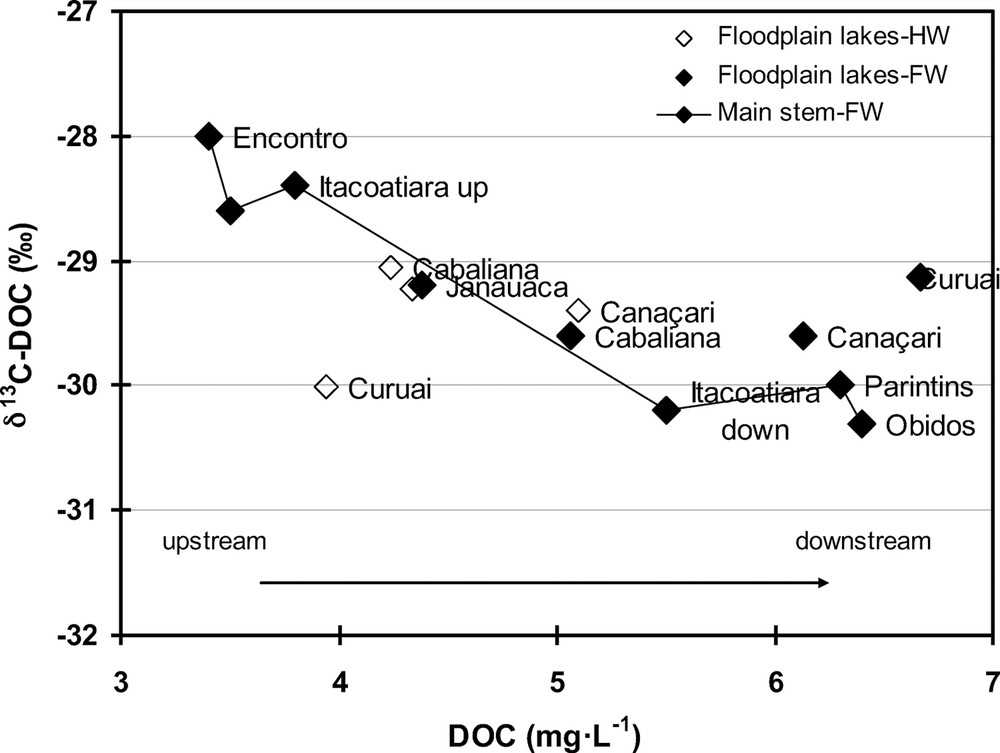
δ13C-DOC versus DOC concentrations in the Amazon mainstem and floodplain lakes during falling waters.
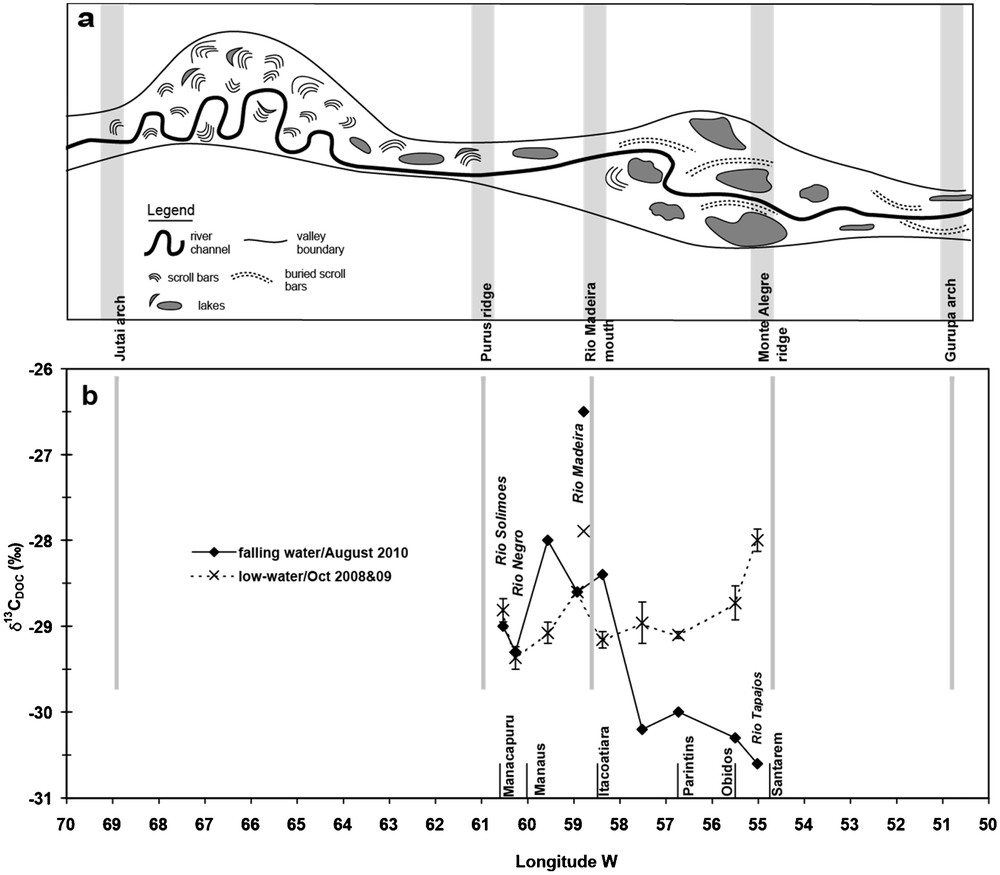
a: schematic illustration of along-stream pattern of channel-floodplain geomorphology and valley width according to Mertes et al. (1996) and Dunne et al. (1998); b: δ13C-DOC values in the mainstem and tributaries between Manacapuru and Santarém at low water and falling water, highlighting the divergence of values downstream of Itacoatiara (data from this study, Fig. 3b).
5 Conclusions
Three reaches in the lowland Amazon mainstem between the Rio Negro confluence and the Tapajós mouth could be distinguished with regard to δ13C-DOC variations: (1) upstream of the Rio Negro and the Rio Solimões confluence, almost no seasonal variation was observed around the regional average of –29.2 ± 0.6‰; (2) upstream of Itacoatiara (Rio Madeira mouth), higher δ13C-DOC values were observed during the falling water period, but the origin of this 13C-enrichment remains to be determined unambiguously; and (3) downstream the Rio Madeira mouth, larger meanders secondary channels and lakes in the floodplain may result in an increased plankton contribution to DOC, leading to a seasonal pattern with lower δ13C-DOC values during high and falling water periods, and higher values during low waters. Our results are based, on the one hand, on 4 to 5 discrete sampling dates in the mainstem along the hydrological cycle, and, on the other hand, on hourly sampling in lakes that have not suggested significant daily variations. To go further would demand a different sampling strategy, coupling phytoplankton dynamic and δ13C studies at a recording rate allowing a better follow of the river regime.
Acknowledgments
This research is a contribution to the CARBAMA project, funded by the French National Agency for Research (grant number 08-BLAN-0221), the French INSU national programme EC2CO, and the National Council of Research and Development (CNPq), Brazil (Universal Program number 477655/2010-6). It was conducted under the auspices of the Environmental Research Observatory HYBAM, supported by the INSU and the IRD (Institute for Research and Development, France). G.A. benefits from a Brazilian CNPq “Science Without Border” fellowship. Particular thanks are addressed to the crew and the captain of the Yane-José-IV (Manaus) for assistance during the cruises. The comments and suggestions of the two anonymous reviewers were a welcome help to improve the manuscript.