1 Introduction
River confluences are ubiquitous features of river networks, representing a region where converging tributary flows determine changes in hydrodynamics, geochemistry, channel geometry, and bed morphology. Since the pioneer study on confluences by Best (1987, 1988), the interest of the scientific community on confluences has grown, as demonstrated by the constant increase of published papers on this topic during the successive two decades (Rice et al., 2008). At that time, most of the studies were realised either on laboratory simulation or on small-scale natural confluences (Parsons et al., 2008). During the last decade, observational studies of large river confluences have been carried out to understand how scale-related changes in confluence geometry will affect the flow structure (e.g., Rhoads, 2006). According to Best and Rhoads (2008), the morphodynamics of the confluence hydrodynamic zone (CHZ; Kentworthy and Rhoads, 1995) can be influenced by factors such as: planform geometry and confluence angle; discharge ratio and bed discordance of the tributaries; as well as difference in density of the incoming flows. Lane et al. (2008) suggest that in the Paraná River the channel width-to-depth ratio (usually larger in large rivers) may play a role in mixing dynamics. In the same river, Szupiany et al. (2009) studied a large braid-bar confluence with a relatively high width-to-depth ratio (∼100), reporting a substantial similarity with features observed in smaller confluences.
Thus, confluence bed morphology appears to be a key factor, related to the different hydrodynamics zones (e.g., Best, 1987, 1988). Common morphological features often observed about confluences include: a scour hole normally orientated along the region of maximum acceleration where both flows begin to converge; sediment avalanche faces at mouth of each tributary; sediment deposition within the stagnation zone; and bars formed within possible flow separation zones or midstream in downstream channel (e.g., Szupiany et al., 2009). Another morphological feature observed at a high portion of confluences is a bed discordance (i.e. one tributary bed is higher than the other), which can be formed through differences in channel discharges and bed geology (e.g., Gaudet and Roy, 1995). More generally, the patterns of erosion and deposition within the CHZ reflect the spatial variations in bed shear stress (Rhoads et al., 2009). The bed shear stress and sediment transport can be related to the localized fluctuations in flow velocity (e.g., turbulence) generated through the interaction of the flow with both vertical and horizontal variations in channel bathymetry causing the flow to accelerate or decelerate (e.g., Best and Rhoads, 2008).
The Negro/Solimões confluence has been the focus of several relevant studies aiming to describe the mixing process of the black and white waters, which show a marked difference in terms of physicochemical characteristics that persists for long distances downstream (Laraque et al., 2009). Bouchez et al. (2010) attributed the lateral heterogeneity across the Solimões River at Manacapuru to the poor mixing of the Purus and Solimões waters and observed that most large rivers are heterogeneous over distances of several tens of kilometres, being bed morphology and channel geometry factors that might affect lateral mixing. Thus, knowledge of bed morphology is important as a boundary condition for hydrosedimentology and morphodynamic studies of rivers.
The present research was carried out in the framework of the CLIM-Amazon Project, a Brazil-Europe bilateral and multidisciplinary scientific cooperation, with the aim of contributing to a better understanding of the hydrodynamic and morphodynamic processes at large confluences of the Amazon River, where the Negro/Solimões confluence represents a peculiar case due to the differences in water and sediment discharge, as well as bed morphology, of the tributaries. In particular, the present paper aims to:
- a) describe the morphodynamic characteristics of a confluence between a bedrock (Negro) and an alluvial (Solimões) river;
- b) verify if the scale-invariance hypothesis (e.g., Sambrook Smith et al., 2005) can be applied to such large confluence;
- c) characterize the geological aspects of the channel morphology and confluence bed.
The observations collected during three field campaigns carried out in 2014 and 2015, which included single-beam and multibeam bathymetry, as well as high-resolution seismic data, are presented with the aim of providing insight on riverbed morphology and stratigraphy, as well as evidences of sediment and flux dynamics about the Negro/Solimões confluence, which ranks as one of the largest confluences on the Earth. A joint paper (Gualtieri et al., 2018) describes and discusses the key findings relative to the hydrodynamic aspects of the confluence, including sediment transport and mixing.
1.1 Geological setting
The confluence of the Solimões and Negro Rivers is situated in the central part of the Amazonian Craton, characterized by a Precambrian basement, outcropping in its northern and southern parts as Guiana and Central Brazil Shields respectively (Almeida et al., 1976). The central part of the Craton, which includes the Amazon Basin, was affected by regional thermal subsidence at the end of the Brazilian Cycle, and covered by Phanerozoic sedimentary and igneous rocks in two different events, represented as two first-order mega-sequences that occurred in the Palaeozoic and Mesozoic–Cenozoic, the latter event being composed of the Cretaceous and Tertiary sequences, which are represented by the Alter do Chão and Solimões Formations (Cunha et al., 2007), forming the Javari Group. Fig. 1 illustrates the location of the study area (lower panel) over a Landsat image, as well as the geology (upper-left panel) obtained from the Geological Map of Amazonas State at the 1:1,000,000 scale (CPRM, 2005). The Alter do Chão Formation (K2ac in Fig. 1) is the sedimentary record of a high-energy fluvial system, flowing from east to west, that floors all the area of the confluence, outcropping around Manaus and on the left margin of the Amazon River, whose deposits have been described as sandstones, argillites and conglomerates, with levels of silicified sandstones (Souza and Nogueira, 2009). After the uplift of the Andean Ridge, in the Miocene, this area received scarce deposition, most of it occurring as Pleistocene–Holocene alluvial deposits (Q2a in Fig. 1) along the river banks (Rossetti et al., 2005). The segment just downstream of the confluence zone is bounded by the outcrop of sandstones from the Alter do Chão formation, and on the left margin by the Quaternary alluvial deposits of Careiro Island.
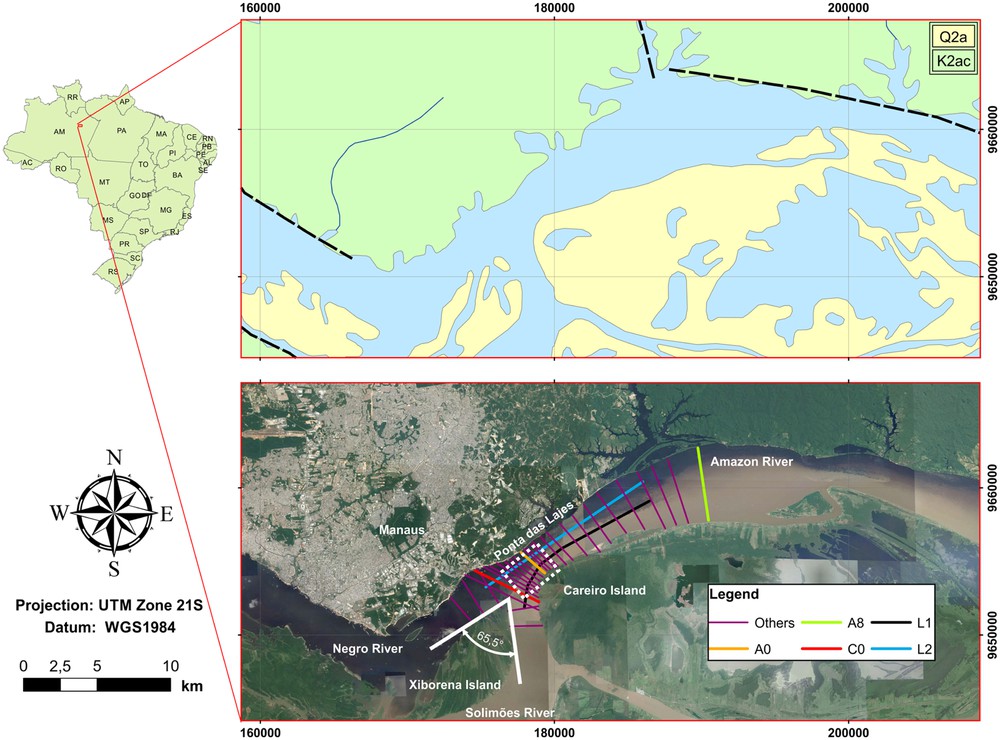
Upper panel: geological setting, with lithology K2ac corresponding to the Alter do Chão Formation and Q2a to recent alluvial deposits and black broken lines representing mapped faults (Geological Map of Amazonas State at the 1:1,000,000 scale–CPRM, 2005). Lower panel: Landsat satellite image of the confluence zone, with location of survey lines for ADCP, seismic and SBES bathymetric sections (FS-CNS1 and FS-CNS2). Area surveyed with MBES is delimited by white dotted polygon (FS-CNS3). The junction angle is measured at the northern tip of Xiborena Island.
1.2 Tectonic setting
While palaeotectonics shaped the Amazonian Craton, the influence of neo-tectonics is evident in the deformations suffered by the Alter do Chão Formation. Following the description of the structural setting proposed by Igreja (2012) and Franzinelli (2011), the area of the confluence is situated in the east–west neo-tectonic transcurrent belt that hosts the Amazon Basin, and is characterized by the main lineaments N40E, N65W, and N75E, representative of the following fluvial geomorphologic features: the last segments of the Solimões and Negro Rivers, as well as the segment just downstream of the confluence. The last segments of the Solimões and Negro rivers follow the direction N40E and N65W, respectively, while the Holocenic deposition in the Xiborena Island, formed by modern sediment banks aligned north–south and curved towards the west, determines the actual confluence angle (Franzinelli, 2011).
1.3 Hydrosedimentologic setting
The mean water discharge of the Negro and Solimões Rivers is approximately 30,000 m3/s and 100,000 m3/s respectively, representing about 14 and 49% of the total fresh water discharge from the Amazon River into the Atlantic Ocean (Filizola and Guyot, 2009). The two rivers have different average hydrological cycles: the Negro shows two distinct discharge peaks over the year, the first, of low amplitude, during the first three months of the year, and the second, larger, in the middle of the year; while the Solimões shows one peak between May and June (Filizola et al., 2009).
In terms of sediment discharge, the difference between the two rivers is striking: the basin of Amazon–Solimões River includes the eastern margin of the Andes, where the combination of high declivity and erodible rocks gives origin to high sediment production (Guyot, 1993); the basin of the Negro River drains the Guiana Shield, which is characterized by gentle gradients and densely vegetated margin, which implies in low sediment production (Filizola and Guyot, 2011). According to data of the Brazilian national data set, the white waters Solimões River has an average discharge of suspended solids of 447·106 t/year at the Manacapuru station, accounting for more than half of the total discharge of the Amazon River into the Atlantic Ocean, while the dark water Negro River has an average suspended load of 8·106 t/year at the Paricatuba station, which is a very low sediment load for the relatively large water discharge of the Negro River (Filizola and Guyot, 2011). It is worth mentioning that recent estimates using remote sensing techniques suggest that the sediment discharge at the Manacapuru fluviometric station could be as high as 825·106 t/year (Espinoza-Villar et al., 2018), almost doubling the previously published values.
Regarding the sediment on the riverbed, no direct comparison is possible from the scarce published data. According to data collected in the period 1982–1984 by Mertes and Meade (1985), the sediments on the bed of the Solimões River, 20 km upstream of the confluence, have a particle size distribution, with more than 99% finer than 0.5 mm, thus classified as fine-to-medium sand in the Wentworth scale. For the Negro River, Latrubesse and Franzinelli (2005) described the bed load of the lower reach as composed of white quartz sand from the Cretaceous sandstone of the Alter do Chão Formation; the proximal origin of the sediments is confirmed by the work of Ferreira (2013), who also describes the grain size distribution as bimodal, with mean diameters of 133.59 μm and 26.69 μm, for the sand and silt fractions, respectively. In an area about 30 km downstream of the confluence, dominated by large bedforms, Almeida et al. (2016) reported an average mean grain size varying from 213 to 375 μm, with coarser average grain sizes located in the deeper thalweg area and finer grain sizes in shallower areas, showing a clear relationship of average grain size and depth.
1.4 Confluence hydrodynamics
ADCP transects were collected during the FS-CNS1 (relatively low-flow conditions) and FS-CNS2 (relatively high-flow conditions) to study the local hydrodynamic, mixing and sediment transport processes about the confluence of the Negro and Solimões Rivers. The ADCP transects collected just upstream of the confluence showed that the cross-sectional averaged flow velocity on the Negro and Solimoes–Amazon Rivers were 0.37 and 1.42 m/s, respectively, with a discharge ratio QN/QS = 0.39 during FS-CNS1 (Gualtieri et al., 2017). During FS-CNS2, the cross-sectional averaged flow velocities were 0.38 and 1.65 m/s on the Negro and Solimões Rivers, respectively, with QN/QS = 0.32 (Gualtieri et al., 2018). Fig. 2 shows the location of hydrodynamic and morphodynamic features observed about the confluence of the Negro and Solimões Rivers during FS-CNS1 as presented in Trevethan et al. (2015b).

Key hydrodynamic and morphodynamic features observed about the confluence during FS-CNS1: (1) stagnation zone, (2) region of deflection, (3) downstream separation zone, (4) region of maximum velocity, (5) region of flow recovery, (6) mixing interface/shear layer, and (7) end of the confluence hydrodynamic zone, (8) scour hole, (9) deposition about stagnation zone, (10) and (10a) deposition about downstream separation zone, and (11) deposition bars about centre of channel, as well as (12) large bedforms on Solimões side of Amazon channel (Trevethan et al., 2015b).
The Xiborena Island, which determines the junction angle of the two rivers, is composed by modern sediments deposited on north–south banks (Franzinelli, 2011) developing in the flow stagnation zone near the upstream junction corner produced by the resultant of the opposite east–west velocity components. Just downstream of the confluence, the flow bends to the right, constrained between the sandstone outcrop of the Alter do Chão Formation on the left margin of the Amazon River, locally known as “Ponta das Lajes” (Franzinelli and Igreja, 2011), and Careiro Island on the right margin of the Amazon River, where modern fluvial deposits blanket the impeded floodplain deposits (Latrubesse & Franzinelli, 2002). As a result of the physical funneling of the flow, acceleration is observed with the maximum vertically-averaged velocity attained on the centre-right part of the channel of the cross-section in correspondence to the narrower part, and the overall velocity increases throughout the section. Past the junction, a bank-attached bar was observed on the right margin of the Amazon River as a result of the deposition occurring in the flow separation region (10) produced by the high junction angle. Trevethan et al. (2015a) found that, during the low flow conditions of FS-CNS1, (5) indicated the position where the flow direction of both the Negro and Solimões sides of the Amazon channel start to align with the channel banks, which indicates the beginning of the flow recovery zone (Best, 1987). It was also observed that at (5) there is still some asymmetry in velocity magnitude either side of the mixing interface. However, by (7) the flow velocity magnitude was observed to be symmetrical across the channel, indicating that the river flow had recovered from the confluences’ hydrodynamic influence and marking the approximate end position of the CHZ, which is expected to vary according to the discharge ratio and total discharge (e.g., Gualtieri et al., 2018).
2 Material and methods
Three field surveys were carried out in and about the confluence hydrodynamic zone of the Negro and Solimões Rivers between October 2014 and July 2015, these being: FS-CNS1 in October 2014 under low-flow conditions (QNp = 28,700 m3/s, QSm = 71,520 m3/s); FS-CNS2 in May 2015 under relatively high-flow conditions (QNp = 26,000 m3/s, QSm = 141,900 m3/s); and FS-CNS3 in July 2015 at the end of the high-flow season on the Solimões river (QNp = 47,200 m3/s, QSm = 149,500 m3/s). Please note, here QNp and QSm refer to the volumetric discharge measured monthly upstream of the confluence with ADCP on the Negro River at Tatu Paricatuba (approximately 30 km upstream) and on the Solimões River at Manacapuru (approximately 100 km upstream), respectively.
The campaigns FS-CNS1 and FS-CNS2 prioritized the study of hydrodynamic and mixing processes about the confluence, while during FS-CNS3 the emphasis was on detailing the morphological structures of the confluence bed. Fig. 1 shows the study region about the confluence with the location of the transects undertaken in FS-CNS1 and FS-CNS2, as well as the area surveyed during FS-CNS3. During FS-CNS1, an Edgetech 3100P chirp sub-bottom profiler operating in the frequency band of 2–15 kHz, allowing a penetration of about 10 m in sandy deposits, with decimetre resolution was used for acoustic characterization of bedrock and sedimentary structures (Ianniruberto et al., 2012). During FS-CNS2, an Odom Echotrack CVM, dual-frequency (33/200 kHz) echosounder was installed over the vessel side to complement the ADCP survey with more detailed bathymetric data. Resolution of the bathymetric data was further improved during FS-CNS3, aiming at a more detailed description of the central part of the CHZ, by the use of a Teledyne–Reson Seabat 7101 swath system, operating at a frequency of 240 kHz, with 511 beam achieving a resolution of 12.5 mm.
3 Results
The data presented here have the purpose of highlighting the characteristics of such large confluence, which is embedded in a complex structural setting that, along and with sediment load and water discharge, contributes in shaping the actual channel. The results obtained by the different sensors is analyzed and integrated in order to provide a more complete understanding of the characteristics of the confluence.
3.1 Bathymetry
Bathymetric survey was carried out during FS-CNS1, FS-CNS2 and FS-CNS3 with different sonars/methods: bathymetric data were obtained by digitising sub-bottom profiles gathered during FS-CNS1, by high-frequency echosounder during FS-CNS2, and multibeam during FS-CNS3. Therefore, the sounding accuracy and density were different across the three surveys; nonetheless, it was possible to merge and integrate the results obtained, and all soundings were referred to the reduction level defined for the Port of Manaus, on the Negro River, established as the 10th percentile of the minimum levels observed in two decades (1986–2006). The river slope was neglected, being on the average 2 cm/km for the Amazon River (Leopoldo et al., 1987). Since the main focus of studies FS-CNS1 and FS-CNS2 was the hydrodynamics of the confluence, bathymetric data were collected along the same sections as ADCP transects, which were planned with density decreasing with distance downstream of the junction (Fig. 1). To account for such different data density, two separate bathymetric models were created by kriging, with a resolution of 50 × 50 m for the region up to 5 km downstream of the junction, and 200 × 200 m from this point to the end of the CHZ. For the interpolation, an overlapping zone of about 1 km in width was used to grant seamless transition.
Fig. 3 presents the bathymetric map obtained by interpolating seismic profiles and echosounder data collected about the confluence during FS-CNS1 and FS-CNS2, respectively. The map reveals structures that correlate with the hydrodynamic and morphodynamic features presented in Fig. 2, namely stagnation zone, region of deflection, downstream separation zone, region of maximum velocity, region of flow recovery, and end of the confluence hydrodynamic zone. Just upstream of the confluence, the beds of the two rivers appear almost concordant, with the thalweg of the Solimões about 10 m deeper than that of the Negro. Around Xiborena Island, it is possible to observe an asymmetric depth gradient on the Negro and Solimões side, being steeper on the latter in response to the higher cross-channel velocity gradient and sediment load. On the Solimões side, the isobaths follow the same north–south alignment of the emerged bars of Xiborena Island.
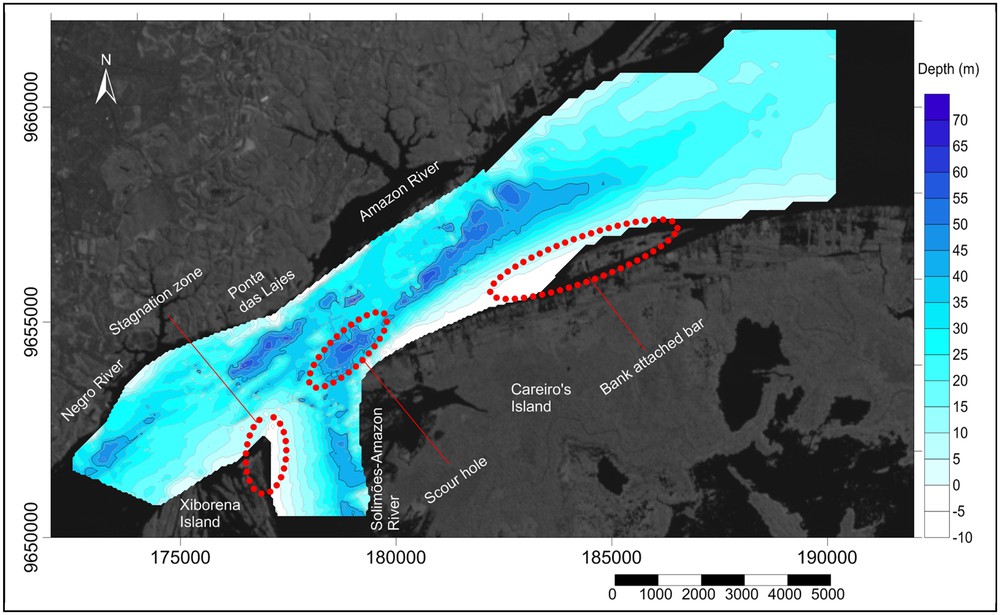
Bathymetry contours obtained by integration of data from FS-CNS1 (seismic profiles) and FS-CNS2 (echosounding). Red dotted areas represent the main morphologic features of the confluence.
Downstream of the confluence, scour holes are observed along the north–east axis (the same as the structural trend) on both margins of the Amazon River. The scour hole next to the right margin coincides with the hydrodynamic flow acceleration region, where the flow attains its maximum velocity due to a funneling effect. On the left margin, scour holes with the same axis direction and greater depths are observed; however, no correspondence with the observed hydrological conditions could be established. The satellite image of the confluence (e.g., background of Fig. 1) shows the presence of a vegetated bar separated by a narrow channel from the margin of Careiro Island, on the right margin of the Amazon River, whose upstream region corresponds to a flow separation and recirculation region, associated with a shallower region (i.e. depth less than 20 m) with a relatively smooth depth gradient, similar to bank-attached bars observed in previous confluence studies (e.g., Best and Rhoads, 2008). This observed shallower region begins downstream of the observed flow separation zone (e.g., Feature (3) in Fig. 2) where the Amazon channel becomes wider and corresponds to regions of decreased flow velocities and bed shear stress (see Gualtieri et al., 2018). Past the maximum velocity zone, the flow starts to recover as the transversal velocity shear decreases and the channel bed becomes wider, flatter and shallower; this region marks the transition to the end of the CHZ (Gualtieri et al., 2018; Trevethan et al., 2015a).
Fig. 4 presents the bathymetry data collected by multi-beam echosounder during FS-CNS3. The MBES survey carried out in a small area just downstream of the confluence gave origin to a high-resolution bathymetric model, with 5·5 m grid cell size, which allowed a more detailed description of some morphologic features, such as a possible sediment avalanche face, scour holes, and terraces. This possible avalanche face is observed on the Solimões side of the confluence and extends beyond the junction into the proximal side of the scour hole.
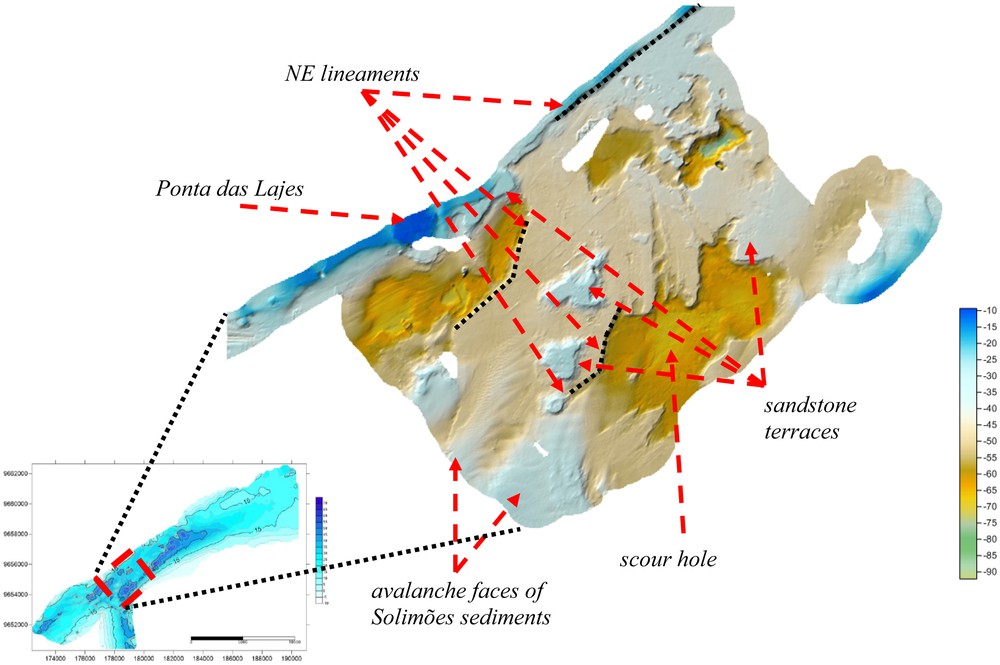
MBES bathymetry obtained during FS-CNS3.
This central part of the CHZ shown in Fig. 4 is characterized by a sediment by-pass in correspondence to the flow funnelling between the “Ponta das Lajes”, on the left margin, and Careiro Island, on the right. The erosive effect of the flux exposes the rocky basement of the river channel, formed by terraces at different levels, cut by lineaments of neotectonic origin. The underwater continuation of the Alter do Chão Formation outcropping in “Ponta das Lajes” is observed at depth of about 10 m, and extending along the left margin of the Amazon River following the direction N50E, which controls the direction of the channel. Three more terrace levels are recognized at average depths of about 18, 28, and 36 m. All these terraces could possibly be lithified sandstone layers, fractured by neo-tectonics and shaped by hydrodynamic action.
Three scour holes, with major axis aligned along the direction N50E, mark the deeper part of the channel, with depths of more than 70 m, being the one close to the right margin, next to Careiro Island, the larger and the only one that corresponds to the observed flow acceleration region. The other scour holes next to the left margin cannot be explained by the observed hydrodynamic conditions during field investigations, as these fall outside the maximum acceleration zone (Feature (4) in Fig. 2). It is also worth observing that the deeper part of the confluence (∼80 m), is observed in the centre-left part of the channel, closer to the Amazon left bank. Such feature is located outside the flow acceleration region (Fig. 2), and could be possibly related to past configuration of the confluence (i.e. junction angle and discharge ratio), or result from neo-tectonics. A depression of similar depth is encountered in the Negro River upstream of the confluence and related to local tectonics (Franzinelli, 2011).
3.2 Seismic
High-resolution seismic profiling provided insight into the shallow sedimentary structures of the confluence region during the FS-CNS1. From these profiles (Figs. 5 and 6), it was possible to observe some sedimentary and rocky features of the confluence bed that correspond to the flow velocities regions and bed shear stresses described in Gualtieri et al. (2018). Two seismic lines, C0 and L1 (Fig. 1), were selected to illustrate the occurrence of the features, transect C0 (Fig. 5) being situated just downstream of the confluence and transversal to the main channel, and longitudinal profile L1 (Fig. 6), which runs parallel to the right margin of the Solimões/Amazonas Rivers, and extends from the upstream reach of the Solimões till the end of the CHZ.
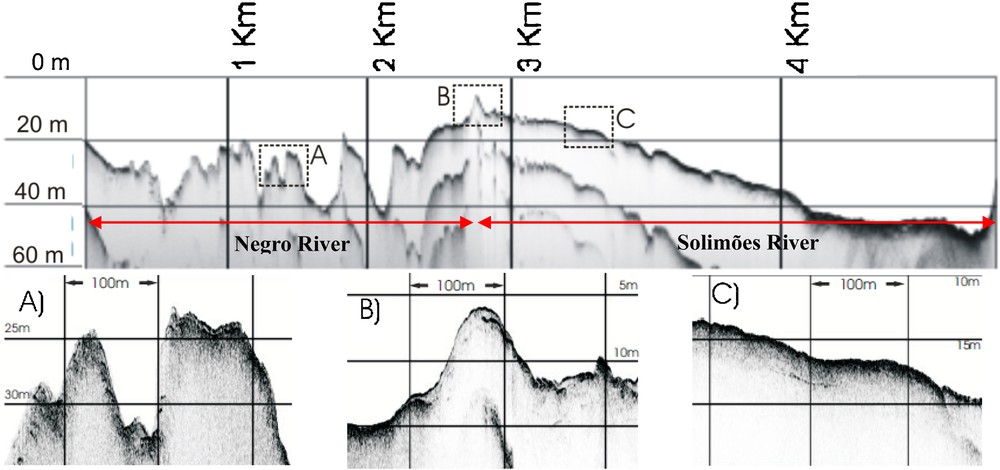
Upper panel: Transversal seismic line C0, just downstream of the confluence from FS-CNS1. Red arrows separate the Negro (left) and Solimões (right) channels. Lower panel: Insets showing the detail of the seismic features.

Upper panel: longitudinal seismic line L1 from FS-CNS1, parallel to the right margin of the Solimões River. Red arrow indicates flow direction. Lower panel: insets showing the detail of the seismic features.
3.2.1 Seismic line C0
Fig. 5 shows the seismic line C0, which is oriented NW–SE, crosses the Amazon channel approximately 200 m downstream of the confluence junction during FS-CNS1 (see line A–A in Fig. 2), where the characteristics of the Negro and Solimões beds still retain the original unmixed features. Given the same gain settings, the Negro side of the profile shows a darker appearance due to the lower conductivity (i.e. salinity) and sediment load, which implies minor attenuation losses (absorption + scattering) of seismic signal than on the Solimões side. The channel on the Negro side is characterized by a bedrock with V-shaped valleys (Fig. 5, Feature A), aligned along N40E, the same structural trend of the Aleixo Fault described by Igreja (2012) and Franzinelli (2011). These observations agree with Latrubresse and Franzinelli (2005), who describe the evolution of Negro River as an incomplete progradational system, due to the change on transported sediment load and increase of the base level as a consequence of the backwater effect, which became a “blocked valley”. The last reach of the Negro River thus was not filled by sediments, retained in upstream reaches, and shows limited sediment cover, with a thickness of about 1 m, which is mostly concentrated in structural traps.
The contrast with the channel on the Solimões side is striking, as on this side the bed changes gradually from bedrock to alluvial across the transition, marked by a shallow anomaly that represents the underwater continuation of the deposits of Xiborena Island at the stagnation zone, just downstream of the junction corner (Fig. 5, Feature B). On the Solimões side, lateral accretion bedforms accompany the progradation into the deeper part of the channel and migration of the thalweg towards Careiro Island (Fig. 5, Feature C). The seismic response of the river bed seems to suggest a coarsening of the sandy sediment, as shown by the diffraction hyperbolas, behaviour that confirms direct observation by Strasser (2008) in other sections of the Amazon River.
3.2.2 Seismic line L1
The Seismic line L1 presented in Fig. 6 starts on the last reach of the Solimões, just before the confluence, and runs downstream parallel to the right margin. At the beginning of the seismic profile, it is possible to observe the presence of bedforms characterizing the avalanche of sediments entering the confluence (Fig. 6, Feature D), ending at the edge of the scour hole, where the line crosses a sequence of three outcropping rocky terraces, with depth of about 25 m. A sharp drop of about 18 m follows the latter outcrop, getting to a dune-covered terrace at depth of about 42 m (Fig. 6, Feature E), before reaching the deeper part of the scour, at about 62 m in depth. After the scour hole, it is observed a wide sandstone terrace at depth of about 35 m, gently sloping downstream, followed by two V-shaped incisions whose downstream limit is marked by a sharp 30-m decrease in depth. Downstream of this large depression, bedforms were observed, marking the beginning of a dune field on the Solimões side as the river thalweg begins the transition from the Solimões to the Negro side of the river (see Fig. 3). The bedform size increases and the shape changes with downstream distance. In Fig. 6, these bedforms can be observed developing into compound dunes from 4.5 km and then from compound dunes into large dunes (wavelength > 200 m; height > 5 m) between 7 and 8 km (Fig. 6, Feature F). It is possible to observe that the height and wavelength of the bedforms increase steadily from the foot of the cliff to the end of the profile, while water depth decreases. Limited signal penetration allows observing only a week reflector just below the beginning of the stoss side of the dunes (Fig. 6, Feature F), which could be interpreted as a reactivation surface similar to those described by Almeida et al. (2016) a few kilometres downstream, next to Jatuarana.
In order to gain better insight into the dynamics of the observed dune system between the two field surveys, the bathymetric profile collected during FS-CNS2 was plotted on the seismic profile (FS-CNS1) of the line L1 (Fig. 7). It is possible to observe that the profile relative to FS-CNS2 is showing large dunes (wavelength > 200 m; height > 5 m) only between 5 and 6 km, while the bed flattens further downstream. It is worth noting that during FS-CNS1, the transect L1 was collected after seven days of near-constant low-flow discharges, while FS-CNS2 was conducted during a period of continuously rising flow discharges (Trevethan et al., 2015a; 2016). This would seem to indicate that the dune field may have obtained a stable equilibrium relative to the flow conditions during FS-CNS1, while dune field was in a transitional phase during FS-CNS2 as it adjusted to the increasing flow discharge.

Comparison of seismic (FS-CNS1) and bathymetric profile (FS-CNS2) along the longitudinal survey line L1. The red arrow indicates the direction of the flow.
4 Discussion
The Negro/Solimões confluence zone is characterized by the same morphologic features as other confluences, with some peculiar aspects linked to the apparent influence of tectonics in shaping the channel, which is limited by outcropping of the Alter do Chão Formation on the left and the floodplains of Careiro Island on the right. Such morphodynamic features are generally related to the characteristic flow zones described by Best (1987), and these relationships were also observed herein and in Gualtieri et al. (2018), namely: regions of flow stagnation; flow deflection; flow separation; maximum velocity; downstream flow recovery; and shear layers. In the following, the main findings of the field survey are related to previous studies highlighting common points and differences.
4.1 Confluence planform and bed
The geometry of the confluence is strongly influenced by the geological control exerted by the outcrops of the Alter do Chão Formation. The work of Gonçalves et al. (2016) on the evolution and reassembly of the Solimões River in the reach between Negro and Madeira tributaries showed that (a) during the last 240,000 years, the channel underwent a northward migration and a transition from meandering to the actual anabranching pattern due to the base level changes; (b) during the last 2000 years, the Solimões fluvial system has shown low rates of channels migration due to the resistance offered by the cliffs of the Alter do Chão Formation. Remote sensing analysis of fluvial dynamics in this same reach carried out by Rozo et al. (2012) confirms the stability of the left margin of the confluence, as opposed to the unconsolidated sedimentary deposits on the right margin over a period of more than thirty years. Furthermore, Mertes et al. (1996) report that the Careiro Island has been in its present location for hundreds of years and that the only significant change was the development of a floodplain channel south of it. In the area of the confluence, the geological control is evident on the left margin of the river, where the Northeast lineaments clearly mark the northward limit of the channel (Fig. 3) whose width increases from a minimum of about 2000 m, where it is constrained between Ponta das Lajes and Careiro Island, to more than 5000 m, about 15 km downstream of the junction (Trevethan et al., 2015a).
All the central part of the confluence, just downstream of the junction corner, is characterized by bare bedrock almost bank to bank (Fig. 4), which is evidently a sediment by-pass region where even bed load is re-routed around the scour hole or re-suspended. This might be explained by the high velocities and bed-shear stresses of the flow on the right side of the confluence, which transports the sediment-laden Solimões waters (Gualtieri et al., 2018), while the relatively lower flow velocities and bed-shear stresses on the left side of the confluence carry the sediment-starved Negro waters. In this region are also observed the greater depths of the surveyed region, corresponding to the funnelling of the flow through the “Ponta das Lajes” – Careiro passage.
4.2 Confluence angle
The confluence angle deserves special attention, as it is considered to be one of the main controls on confluence hydrodynamics for it determines the extent of the separation zone and the bed morphology (e.g., Biron and Lane, 2008). At the confluence of the Negro and Solimões, the junction angle is a dynamic variable as shown in the work of Franzinelli (2011) that reports a change from 15° to 90° based on the observation of the depositional bars of the Xiborena Island. According to Passos and Soares (2017), who used an historical series of Landsat satellite images (1991–2009) to estimate the eastward displacement of the western side of the Xiborena Island of about 1.2 km and the final junction angle of 75°. Such analyses are complemented by the underwater observation of the deposition dynamics around the Xiborena Island, as shown in Figs. 3 and 5 (upper panel and Feature B), which confirms the trend of sediment accretion and bank erosion on the western Xiborena Island and eastern Careiro Island boundaries, respectively. As expected, the growth of the Xiborena Island occurs mostly at the right side of the stagnation zone (Fig. 1), due to the larger sediment transport of the Solimões.
4.3 Scour hole
At the confluence, the Negro and the Solimões rivers have a direction of about 55°N and 350°N, respectively, giving a confluence angle of about 65°, while the direction of the maximum scour localized next to the Careiro Island is about 50°N, attaining a depth of more than 70 m (Figs. 3 and 4). According to Best (1988): (a) higher junction angles and higher discharge ratios give origin to greater scour depths; (b) the orientation of the maximum scour is bisecting the confluence angle and gets aligned with tributary channel at increasing discharge ratios. For the Negro/Solimões confluence, given a maximum scour depth of about 80 m and an average upstream depth of 20 m, the relative scour depth is about 4, which compares well with the compilation of data reported by Sambrook Smith et al. (2005). On the other hand, the alignment of the scour does not fit the model, as it is more almost aligned with the Negro direction, despite the much lower discharge of this river. If the relative scour depth value might contribute to confirm the scale invariance hypothesis (Best and Rhoads, 2008; Sambrook Smith et al., 2005), a question could be raised regarding the origin and dynamics of this particular scour, which could be influenced by the evolution of the confluence angle, which, due to the almost unilateral sediment accretion occurring at the stagnation zone around the Xiborena Island, has progressively increased by turning the Solimões channel over time, from 15°N (Franzinelli, 2011) to the actual junction angle, leading, in turn, to a deepening of the scour zone. The peculiar geological settings of this confluence must be considered, as the southern boundary of the scour hole shows a piecewise linear trend with directions 60°N, 10°N, 30°N, compatible with the general structural trend NE–SW (Costa et al., 2001; Silva et al., 2007). This suggests that the shape and depth of the scour hole might be controlled by neo-tectonic fractures in the lithified layers of the Alter do Chão Formation, and that the relatively low channel migration rates (Gonçalves et al., 2016; Rozo et al., 2012), along with the high-flow velocities, might have allowed enough time to erode the bedrock.
Regarding the position of the scour hole in a confluence, Rhoads et al. (2009) investigated the morphodynamics of the channel bed at a small stream confluence and observed the shifting of the scour as a function of discharge ratio. In partial agreement, Szupiany et al. (2009) concluded that the scour position appears to depend on the discharge ratio, being closer to the bank opposite to the tributary with largest discharge; however, it is also observed that in large channels (high width to depth ratio) the cores of maximum velocity might have the greatest influence. For this confluence, the width-to-depth ratio is highly variable, and the observed discharge ratio QN/QS was between 0.3 and 0.40 during FS-CNS1 and FS-CNS2 (Gualtieri et al., 2018). Of the three scours observed in the confluence area, two are closer to the left bank of the Negro and Amazon channel and do not match the high velocity cores observed in the hydrodynamic data collected in this study (Gualtieri et al., 2018; Trevethan et al., 2015a, 2016). Changes in the hydrodynamic conditions and discharge ratio are a possible explanation, along with the combined effects of different confluence morphology and junction angle, as discussed in Franzinelli (2011). However, several other possibilities could be suggested: the possibility of those being palaeo-scour holes formed in higher energy environments, as could occur during sea-level fall, as reported by Best and Ashworth (1997) for the sand-bedded Jamuna and Ganges Rivers confluence, or the possibility that these features are determined by the tectonics, as could be suggested by the normal faults delimiting the scour hole as observed in the seismic profile in Fig. 6; or a combination of both.
4.4 Sedimentary features
The sedimentary features commonly encountered in river confluences are a region of sediment deposition in the stagnation region near the junction corner, tributary-mouth bars sloping into the scour, bank-attached bar in correspondence with the flow separation/recirculation region and mid-channel dune systems in the flow recovery zone (Best and Rhoads, 2008). The peculiarity of the features encountered in this confluence are again related to the different sediment load of the converging rivers, so that:
- a) the possible avalanche faces observed in Fig. 4 seem to be composed of two deposition fans of sediments of the Solimões, being the northernmost related to the stagnation region (low depth–averaged velocity and bed shear stresses) and the other to the train of composite bars that fines up while avalanching into the scour (Fig. 6D) and the region of flow acceleration and high bed-shear stresses (Gualtieri et al., 2017);
- b) a large bank-attached bar-type formation was observed downstream of the flow recirculation and separation zone on the Solimões side of the Amazon channel, which corresponds to lower flow velocities and bed shear stress (Gualtieri et al., 2018). Its growth may accompany the increase in the junction angle, which is responsible for the formation of a larger region of low velocities, or even reverse velocities forming a recirculation region (Best, 1988);
- c) the dune system occurs past an abrupt transition from bedrock to alluvial bed marked by a normal fault (Fig. 6, 4.5 km downstream) and marks the end of a region where the effect of the confluence on the transfer of momentum is observed (Szupiany et al., 2009) and corresponds to the higher mid-channel bed-shear stresses (Gualtieri et al., 2018). Dunes are observed to grow during high-flow and reach their maximum after peak discharge, then increase in length with reduced height during the falling stage, however large dunes can persist during low-flow conditions, as discussed in previous studies (e.g., Chen et al., 2012). Besides discharge, to the downstream reach of the Negro/Solimões confluence, another variable must be taken in account as the width-to-depth ratio of the channel increased by a factor of five between transects A0 and A8 because of the combined increase in channel width and decrease in average depth (Gualtieri et al., 2018). During FS-CNS1, the dunes seem to undergo an evolution with downstream distance from the cliff, from relatively small wavelength compound dunes to large dunes, while during FS-CNS2 the dunes decrease the height and increase the wavelength downstream (Fig. 7). Considering that FS-CNS1 took place during a stable low-flow stage and FS-CNS2 during a raising stage, the two profiles seem to have captured the transient condition of the dune field with flow discharge in this region.
5 Conclusion
The study of the confluence of such two large rivers was a challenge due to the dimensions (i.e., width and depth) of these channels, along with the added complexity of rivers draining two very distinct hydrographic basins, sediment load and geological settings. Three survey campaigns about the confluence of the Negro and Solimões Rivers allowed the description of key morphological features commonly observed in past confluence studies, but also showed some unusual features that seem related to past hydrological conditions and the local neo-tectonics. These observed morphological features include: a stagnation zone observed as the underwater continuation of Xiborena Island, whose growth from sediment deposition over time seems to contribute to modifying the junction angle; the scour hole which is an erosional feature on the Alter do Chão Formation, the outcropping geological basement that characterizes the study area, possibly controlled by neo-tectonics; deposition of sediment is observed within and past the flow separation region on the Solimões side of the confluence bend, forming a large bank-attached bar; and further downstream the abrupt appearance of sedimentary bedforms on the Solimões side of the Amazon River past a sharp bedrock terrace. Conversely, two erosional features were detected close to the left margin of the Amazon River, which cannot be explained by observed hydrological conditions during this investigation and may therefore most likely be related to the past hydrological conditions and configuration of the confluence, or even relict basement features.
One of the main questions addressed herein was the hypothesis of scale invariance, as most of the previous studies were carried out in smaller rivers or in laboratory experiments. The data presented here mostly corroborate the results and models of previous studies, while the observed differences can probably be attributed to the peculiar geologic and hydrologic setting of this confluence, which is one of the first detailed studies conducted about the confluence of two among the largest rivers on the Earth, with one channel bed being alluvial, and the other bedrock. Furthermore, the confluence dynamics are strongly affected by structural control due to the funneling imposed by rocky outcrops exposing the sandstone terraces of the Alter do Chão Formation within the central CHZ. For this reason, the observed difference of scour hole position and orientation with respect to past studies might simply reflect the longer time scale necessary to morph bedrock as compared to alluvium.
Acknowledgements
The research was carried out within the CLIM-AMAZON European Laboratory in Brazil funded by grant FP7 INCO-LAB No. 295091 from the European Commission. The survey campaign FS-CNS3 was carried out thanks to the support of the team of the FAPESP project coordinated by Prof. Renato Pães de Almeida (University of São Paulo). The authors acknowledge the support of the Geological Survey of Brazil, the Federal University of Amazonas, Ruraltech Ltda, as well as of graduate students Daniela Moreira Andrade, and Jonas Pereira Lion. The authors would also like to thank the editor and the two reviewers for the valuable suggestions and comments that led to a significant improvement of the work.