1 Introduction
Silver–lead–zinc veins hosted in clastic sedimentary sequences (CSS Pb–Zn–Ag veins) form a distinct class of mineral deposits with a distinctive mineralogy, associated with crustal-scale faults (Beaudoin and Sangster, 1992). Several examples of this class of deposits have been documented around the world, in the Kokanee Range (Beaudoin and Sangster, 1992), Keno Hill district (Lynch et al., 1990) and Purcell Basin (Paiement et al., 2012) in Canada; the Freiberg district (Baumann, 1994) and Harz Mountains (Lüders and Möller, 1992) in Germany; the Coeur d’Alene district (Fleck et al., 2002; Leach et al., 1998; Lydon, 2007) in the USA; the Pribram district (Zàk and Dobes, 1991) in the Czech Republic; and the Pumahuasi district in Argentina (Segal et al., 1999). Based on a worldwide review, Beaudoin and Sangster (1992) proposed a descriptive model for CSS Pb–Zn–Ag veins and discussed different alternative models for their genesis.
The Paramillos de Uspallata Pb–Zn–Ag vein deposit (32°28′29.6″S; 69°08′46″W) is hosted in the northern part of the Cuyo Basin, which extends along ∼60,000 km2 between 31° and 36° S in the Andean Cordillera (Fig. 1). This basin is the largest of the Triassic basins of Southwest Gondwana in Argentina related to a generalized extension linked to the collapse of the Permian orogen and the beginning of the Mesozoic Gondwana break-up (Legarreta and Uliana, 1996; Tankard et al., 1995; Uliana et al., 1989).

Location of the Cuyo Basin and geological sketch of the Paramillos de Uspallata vein deposit area. Star: study area.
From the 17th century until the early 1980s, the Paramillos de Uspallata Pb–Zn–Ag vein-type deposit was discontinuously mined, producing nearly 414,000 t of ore at ∼2.5% Pb, 3.5% Zn and 475 g/t Ag (Lavandaio and Fusari, 1999). At present, there are only a few intense oxidized outcropping veins, and only ∼1,200 m of the ∼20,000 m of underground documented galleries are currently accessible. It is currently a geopark where it is possible to visit the ruins of the old miner town and access the underground mining works. Although it is the oldest mine in Argentina, there are few previous studies about this deposit. These include the description of the mining works (Rayces, 1949), some specific fluid inclusions and isotope studies (Garrido et al., 2001), and limited geochemical work (Lavandaio and Fusari, 1999). Thus, its genesis still remains controversial.
The aim of this study was to build a new descriptive model of the Paramillos de Uspallata Pb–Zn–Ag vein deposit based on both new and existing metallogenic information, particularly mineralogical, petrographic, and isotopic data, in order to elucidate its genesis and provide insights into a class of mineral deposit whose genesis still remains partly unresolved.
2 Geological setting
2.1 Regional geology
The Cuyo Basin is a passive rift system located in western Argentina (Fig. 1). It is composed of asymmetric half-grabens controlled by preexisting zones of weaknesses in the basement related to the ancient Gondwanan sutures separated by transfer faults or intrabasinal highs that led to different depocenters. The basin infill consists of up to 3700 m of a volcano-sedimentary sequence overlying a basement consisting of Paleozoic sedimentary rocks and Permian–Triassic volcanics (Choiyoi Group). The lower part of this sequence is composed of coarse-grained alluvial, fluvial, lacustrine, deltaic, and volcaniclastic deposits of the Early to Mid-Triassic synrift stage, a stage characterized by tectonic subsidence related to the activity of the main fracture systems along the active flank of the half-grabens (Spalletti, 1999). During this synrift phase, tholeiitic to slightly alkaline basalts with moderately steep rare-earth element patterns and mantle-like isotopic ratios emplaced during the Middle Triassic (∼235 Ma) (Massabie, 1986; Ramos and Kay, 1991; Rocher et al., 2015). The chemistry of these basalts suggests relatively low degrees of melting (4 to 5%), which is consistent with the comparatively narrow width of the Cuyo Basin (Ramos and Kay, 1991). The synrift deposits are overlaid by Middle to early Late Triassic dominant fluvial-lacustrine successions deposited under thermal-tectonic subsidence conditions (sag phase) of the basin. The upper part of these sag deposits is composed of Late Triassic alluvial–fluvial successions related to tectonic subsidence reactivation. The thermal relaxation of the basin finished with the flexural subsidence of the lithosphere triggered by the Andean Orogeny during the Cenozoic (Barredo, 2012; Spalletti, 1999).
By the latest Early Miocene, the collision of the Juan Fernández Ridge resulted in the shallowing of the subducting plate between 28 and 33°S, which was accompanied by a change in the deformation regime from transpressional to compressional, crustal thickening, eastward frontal arc migration, and a change in the geochemical signature of the magmatism (Kay and Mpodozis, 2002; Yáñez et al., 2001). Synchronously with the initiation of the compressional deformation and arc migration, a dominant andesitic magmatism erupted in a broadened arc that extended into the western Precordillera (Carrasquero et al., 2017).
2.2 Deposit-scale geology
The Paramillos de Uspallata vein-system deposit is hosted in the Triassic synrift volcano-sedimentary sequence locally named Uspallata Group (Fig. 1), which includes the Paramillos and Agua de la Zorra Formations. The Paramillos Formation is a volcano-sedimentary unit which hosts an in situ fossil forest of conifers and corystosperms (Brea et al., 2009). It is composed of sandstones, tuffaceous sandstones, shales, mudstones, and tuffs, which were deposited in a meandering fluvial system (Brea et al., 2009) during the upper Middle Triassic (∼239–230 Ma, Spalletti et al., 2008). The overlying Agua de la Zorra Formation consists mainly of bituminous shales and marls with minor interbedded sandstones and mudstones, which were deposited in a fluvial shallow lacustrine environment with episodic eruptive events (Brea et al., 2009; Cortés et al., 1997).
Interbedded with the Paramillos and Agua de la Zorra Formations, there are basaltic lava flows, locally with vesicles filled with carbonate and minor quartz, and subordinate basaltic sills and dykes. The volcanics have porphyritic texture with plagioclase, clinopyroxene (augite) and scarce olivine phenocrysts in an intergranular to intersertal groundmass composed mainly of plagioclase with variable augite and abundant magnetite. These mafic rocks show variable alteration depending on their vesicularity and their primary glass content with their igneous texture widely retained. The alteration paragenesis is dominated by ubiquitous chlorite along with carbonate and titanite, with minor epidote, prehnite and pumpellyite as well as very scarce albite, actinolite, quartz, and zeolites. The alteration minerals occur as a replacement of interstitial areas and primary minerals and infilling of vesicles and veins. This paragenesis corresponds to very low-grade metamorphism in the prehnite–pumpellyite facies in metabasites.
The Triassic sequence is intruded by arc andesitic subvolcanic rocks (Cerro Redondo Formation, Fig. 1) of latest Early Miocene age (∼20–16 Ma, Kay et al., 1991; Massabie, 1986). Cu–Mo porphyry-type and Au-epithermal-type deposits are spatially and genetically associated with these Miocene magmatic rocks (Carrasquero et al., 2011; Navarro, 1972).
3 Zn–Pb–Ag mineralization
The Paramillos de Uspallata deposit includes 33 subvertical veins with predominantly NW and WNW and subordinate WSW strikes hosted in the volcano-sedimentary Triassic sequence (Figs. 1 and 2a). These veins are of up to ∼2 m thick and extend for up to ∼2500 m along strike and consist of sulfides and sulfosalts and siderite as the main gangue mineral, typically displaying crustiform, cockade, and breccia textures (Fig. 2b). The wall-rock alteration surrounding the veins is moderate to intense and includes pervasive sericitization and carbonatization and veinlet-type silicification.
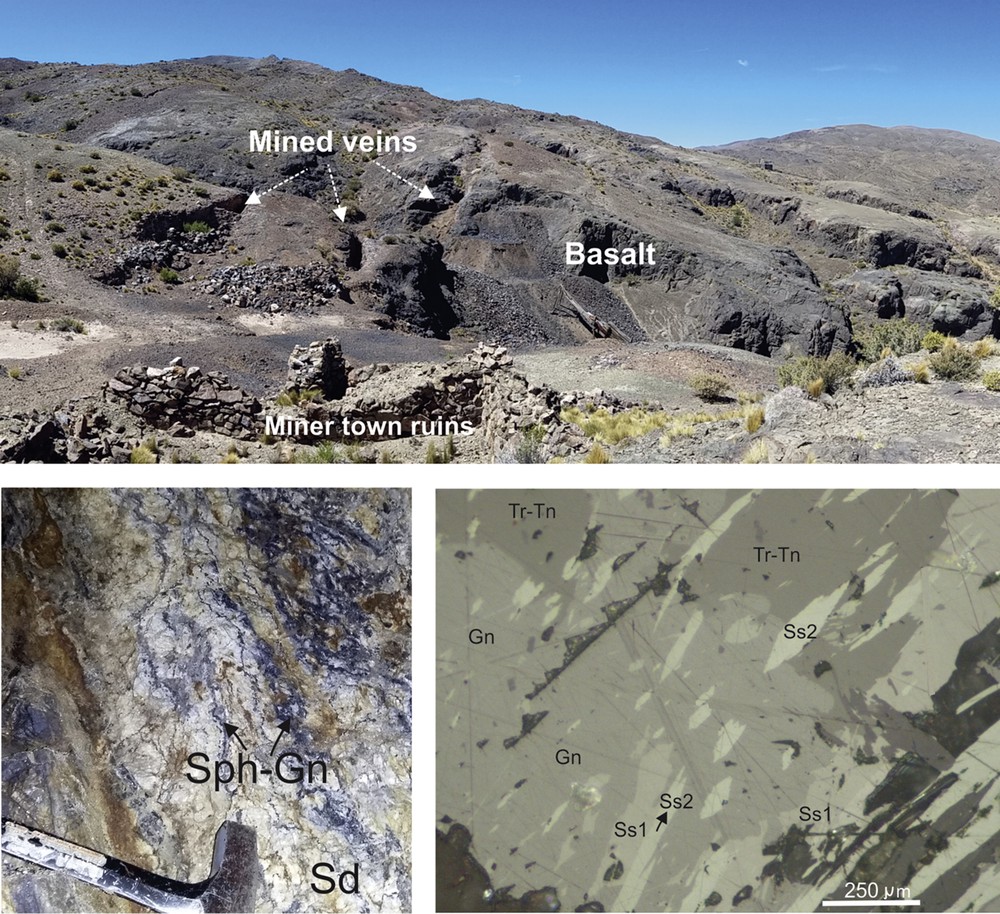
A. Mined veins hosted by the Triassic basalts. B. Vein consisting of siderite (Sd) and sulfides (mainly galena, Gn and sphalerite, Sph) displaying characteristic crustiform texture. C. Galena (Gn) replaced by a mineral of the tetrahedrite–tennantite series (Tt–tn), which is variably replaced by different sulfosalts (Ss1 and Ss2).
Ore petrography studies have shown that the mineralization consists of an intergrowth of galena and sphalerite with chalcopyrite disease, minor chalcopyrite, and scarce pyrite in a siderite with minor quartz gangue. These sulfides are variably replaced by a mineral of the tetrahedrite–tennantite series, which is, in turn, variably replaced by tabular and acicular sulfosalt aggregates (Ss1 and Ss2, see Fig. 2c). Late discontinuous veinlets of arsenopyrite and marcasite in a quartz gangue cut the above-mentioned ore mineral assemblage.
4 Results
4.1 Mineral chemistry and ore paragenetic sequence
The sulfides and sulfosalts of four vein samples were chemically analyzed with a Cameca SX-100 Electron Probe Microanalyzer (EPM) in the Research Centre for Physical and Environmental Science, Open University, UK. The analytical conditions were 20 kV for the accelerating voltage, 20 nA for the beam current and a beam diameter of 2, 5, and 10 μm. The standard reference materials used for calibration were S (Kα/Sp3), Ag (Lα/Sp5), Fe (Kα/Sp1), Mn (Kα/Sp1), Cu (Kα/Sp3), Zn (Kα/Sp1), Cd (Lα/Sp5), Hg (Lα/Sp3), (Pb Mα/Sp5), Sb (Lα/Sp4), As (Kα/Sp1), and Bi (Mα/Sp4). The representative composition and the structural formula of the mineral phases analyzed are presented in Table 1.
Representative analyses (by means of Electron Probe Microanalyzer) of sulfides and sulfosalts from the Paramillos de Uspallata deposit.
Galena | Pyrargyrite | Sphalerite | Arsenopyrite | Chalcopyrite | Marcasite | Boulangerite | Owyheeite | Tetrahedrite-Freibergite | ||||||||
S (wt%) | 14.90 | 13.77 | 16.86 | 32.50 | 32.46 | 20.93 | 34.18 | 33.66 | 52.89 | 52.29 | 18.71 | 19.07 | 19.58 | 20.29 | 20.89 | 22.50 |
Ag | 1.99 | 0.00 | 57.34 | 0.03 | 0.01 | 0.03 | 0.00 | 0.11 | 0.03 | 0.15 | 0.00 | 0.00 | 10.00 | 9.04 | 27.55 | 20.78 |
Fe | 0.12 | 0.09 | 0.00 | 0.85 | 8.40 | 34.93 | 29.15 | 28.53 | 45.32 | 45.42 | 0.01 | 0.04 | 0.10 | 0.08 | 3.77 | 5.54 |
Mn | 0.00 | 0.00 | 0.00 | 0.00 | 0.03 | 0.00 | 0.08 | 0.00 | 0.03 | 0.17 | 0.00 | 0.01 | 0.00 | 0.00 | 0.01 | 0.00 |
Au | 0.00 | 0.00 | 0.00 | 0.43 | 0.44 | 0.00 | 0.00 | 0.00 | 0.00 | 0.19 | 0.00 | 0.00 | 0.00 | 0.00 | 0.00 | 0.08 |
Cu | 0.44 | 0.01 | 0.02 | 0.06 | 0.00 | 0.01 | 33.60 | 33.11 | 0.02 | 0.02 | 0.01 | 0.00 | 0.16 | 0.15 | 18.10 | 22.32 |
Zn | 0.01 | 0.11 | 0.13 | 63.72 | 54.59 | 0.42 | 0.02 | 1.17 | 0.44 | 0.03 | 0.00 | 0.11 | 0.00 | 0.00 | 2.09 | 1.03 |
Cd | 0.00 | 0.00 | 0.00 | 0.37 | 0.01 | 0.00 | 0.00 | 0.00 | 0.00 | 0.00 | 0.00 | 0.00 | 0.00 | 0.00 | 0.00 | 0.00 |
Hg | 0.09 | 0.00 | 0.06 | 0.00 | 0.00 | 0.06 | 0.00 | 0.04 | 0.00 | 0.03 | 0.00 | 0.02 | 0.04 | 0.04 | 0.07 | 0.03 |
Pb | 83.23 | 84.67 | 0.00 | 0.08 | 0.08 | 0.06 | 0.00 | 0.10 | 0.25 | 0.28 | 55.90 | 55.45 | 39.77 | 38.39 | 0.00 | 0.03 |
Sb | 0.01 | 0.23 | 19.72 | 0.11 | 0.01 | 0.49 | 0.00 | 0.00 | 0.00 | 0.03 | 24.13 | 24.75 | 29.74 | 31.37 | 25.95 | 26.96 |
As | 0.00 | 0.00 | 4.41 | 0.59 | 1.08 | 41.96 | 0.10 | 0.24 | 0.00 | 0.00 | 0.00 | 0.00 | 0.00 | 0.00 | 0.27 | 0.36 |
Bi | 0.00 | 1.11 | 0.17 | 0.07 | 0.11 | 0.06 | 0.12 | 0.11 | 0.19 | 0.16 | 0.73 | 0.75 | 0.56 | 0.55 | 0.13 | 0.04 |
Sum | 100.78 | 100.00 | 98.71 | 98.80 | 97.23 | 98.95 | 97.24 | 97.07 | 99.18 | 98.77 | 99.48 | 100.20 | 99.95 | 99.91 | 98.84 | 99.67 |
2 atoms | 7 atoms | 2 atoms | 3 atoms | 4 atoms | 3 atoms | 20 atoms | 52 atoms | 30 atoms | ||||||||
S | 1.04 | 1.01 | 2.87 | 1.00 | 1.00 | 1.06 | 2.01 | 2.00 | 2.00 | 1.99 | 11.06 | 11.10 | 27.69 | 28.21 | 12.95 | 13.26 |
Ag | 0.04 | 0.00 | 2.90 | 0.00 | 0.00 | 0.00 | 0.00 | 0.00 | 0.00 | 0.00 | 0.00 | 0.00 | 4.20 | 3.73 | 5.08 | 3.64 |
Fe | 0.00 | 0.00 | 0.00 | 0.01 | 0.15 | 1.01 | 0.98 | 0.97 | 0.99 | 0.99 | 0.00 | 0.01 | 0.08 | 0.07 | 1.34 | 1.88 |
Mn | 0.00 | 0.00 | 0.00 | 0.00 | 0.00 | 0.00 | 0.00 | 0.00 | 0.00 | 0.00 | 0.00 | 0.00 | 0.00 | 0.00 | 0.00 | 0.00 |
Au | 0.00 | 0.00 | 0.00 | 0.00 | 0.00 | 0.00 | 0.00 | 0.00 | 0.00 | 0.00 | 0.00 | 0.00 | 0.00 | 0.00 | 0.00 | 0.01 |
Cu | 0.02 | 0.00 | 0.00 | 0.00 | 0.00 | 0.00 | 1.00 | 0.99 | 0.00 | 0.00 | 0.00 | 0.00 | 0.12 | 0.11 | 5.66 | 6.64 |
Zn | 0.00 | 0.00 | 0.01 | 0.97 | 0.83 | 0.01 | 0.00 | 0.03 | 0.01 | 0.00 | 0.00 | 0.03 | 0.00 | 0.00 | 0.64 | 0.30 |
Cd | 0.00 | 0.00 | 0.00 | 0.00 | 0.00 | 0.00 | 0.00 | 0.00 | 0.00 | 0.00 | 0.00 | 0.00 | 0.00 | 0.00 | 0.00 | 0.00 |
Hg | 0.00 | 0.00 | 0.00 | 0.00 | 0.00 | 0.00 | 0.00 | 0.00 | 0.00 | 0.00 | 0.00 | 0.00 | 0.01 | 0.01 | 0.01 | 0.00 |
Pb | 0.90 | 0.96 | 0.00 | 0.00 | 0.00 | 0.00 | 0.00 | 0.00 | 0.00 | 0.00 | 5.40 | 5.35 | 8.70 | 8.26 | 0.00 | 0.00 |
Sb | 0.00 | 0.00 | 0.01 | 0.00 | 0.00 | 0.01 | 0.00 | 0.00 | 0.00 | 0.00 | 3.96 | 4.07 | 11.08 | 11.49 | 4.24 | 4.18 |
As | 0.00 | 0.00 | 0.32 | 0.01 | 0.01 | 0.91 | 0.00 | 0.01 | 0.00 | 0.00 | 0.00 | 0.00 | 0.00 | 0.00 | 0.07 | 0.09 |
Bi | 0.00 | 0.01 | 0.00 | 0.00 | 0.00 | 0.00 | 0.00 | 0.00 | 0.00 | 0.00 | 0.07 | 0.07 | 0.12 | 0.12 | 0.01 | 0.00 |
The EPM analyses and back-scattered electron images (Fig. 3a) of galena showed very small pyrargyrite inclusions. In addition, they showed low and erratic contents of Cu (up to ∼0.4%), Bi (∼1%), Sb (up to ∼0.23%), and Ag (up to 2%), and traces of Fe, Hg, and Zn. The contents of Ag could be due to the presence of sub-microscopic inclusions of pyrargyrite. Sphalerite showed variable Fe (∼0.8–8%) and low Au (∼0.4%) contents, whereas chalcopyrite showed a homogeneous composition with low and erratic contents of Zn (up to ∼1%) and traces of Ag, Pb, As, and Bi.

Back-scattered electron images. A. Pyrargyrite (Pyr) inclusions in galena. B. Mineral of the freibergite–tetrahedrite series (Frei-Ttr) with different shades of gray due to the variable contents of Ag along the crystal that replaces galena (Gn) and chalcopyrite (Ccp). C. Boulangerite (Boul) and owyheeite (Owy) replacing galena. D Marcasite (Mrc) in fine aggregates with banded texture and arsenopyrite (Apy) in quartz gangue.
The structural formula of the mineral identified by ore petrography as tetrahedrite–tennantite (Table 1) revealed that it is a member of the freibergite–tetrahedrite series (see Riley, 1974). This mineral displayed variable contents of Ag (∼20 to 27%) heterogeneously distributed in the crystals (Fig. 3b) and very low contents of As (< 0.4%). The EPM analyses also showed that the tabular and acicular sulfosalt aggregates that replace the freibergite–tetrahedrite are composed of boulangerite and owyheeite (Fig. 3c). Boulangerite is typically stoichiometric and has low contents of Bi (∼0.7%), whereas owyheeite has a chemical composition that fits with that obtained by Moëlo et al. (1984) for owyheeites from different ore deposits around the world, but with higher Ag (∼9–10% instead of ∼5–7%), similar to that reported for Rivertree, New South Wales (Lawrence, 1962). The images show that marcasite occurs as fine aggregates with banded texture (probable after melnikovite, see Fig. 3d). Finally, arsenopyrite displays a homogeneous composition with traces of Ag, Mn, Au, Pb, and Bi. Ore petrographic studies plus mineral chemistry results allowed establishing the paragenetic sequence shown in Fig. 4.

Paragenetic sequence of the Paramillos de Uspallata deposit. The width of the bars indicates the relative abundance of the ore minerals.
4.2 Oxygen and carbon isotopes
The results of oxygen isotope analyses performed on quartz and oxygen and those of carbon isotope analyses performed on siderite are reported in Table 2 along with data from Garrido et al. (2001).
Oxygen and carbon isotope compositions of quartz and siderite from different veins of the Paramillos de Uspallata deposit. The isotope fractionation in fluids was calculated according to Sharp et al. (2016), Zheng (1999) and Golyshev et al. (1981) at 190 °C and 210 °C respectively (statistical mode temperatures from Garrido et al., 2001).
Vein | δ18O SMOW quartz |
δ18O SMOW fluid (quartz) |
δ18O SMOW siderite |
δ18O SMOW fluid (siderite) |
δ13C PDB siderite |
δ13C PDB fluid (siderite) |
Belén | 14.21 | 1.711; 3.015 | ||||
Mendoza | 15.25 | 2.739; 4.043 | ||||
San Lorenzo | 16.52 | 3.993; 5.299 | ||||
San Miguel | 16.16 | 3.637; 4.943 | ||||
Santa Rita | 16.2 | 3.677; 4.983 | ||||
San Pedro | 20.84 | 9.067; 10.278 | –2.97 | –6.255; –5.559 | ||
Tajo (1)a | 13.2 | 0.714; 2.016 | 19.1 | 7.347; 8.556 | ||
Tajo (2)a | 12.3 | –0.175; 1.126 | 16.3 | 4.579; 5.785 | ||
Tajo (3)a | 11.5 | –0,965; 0,334 | 18.4 | 6.655; 7.863 | ||
Tajo (4)a | 13.8 | 1.306; 2.609 | 17.4 | 5.666; 6.873 |
a Data from Garrido et al. (2001).
The quartz concentrates were reacted with BrF5 according to the method of Clayton and Mayeda (1963) at the Stable Isotope Laboratory of Université Laval, Québec, Canada, and the CO2 was analyzed by Isotope-ratio mass spectrometry (IRMS) at the Geological Survey of Canada, Québec. The accuracy and precision of the 18O values were verified by analysis of NBS-28. Isotope ratios are reported in the δ-notation relative to V-SMOW with a precision better than 0.2‰.
Siderite was reacted with 100% phosphoric acid at 25 °C and the CO2 obtained was analyzed by IRMS, using an internal standard periodically calibrated with the International NBS-19 (calcite) standard at the ACTLABS Laboratories, Canada. Isotope ratios are reported in the δ-notation relative to V-SMOW for oxygen and PDB for carbon, with a precision better than 0.2‰ for 13C and 18O.
In general, the δ18O values of the hydrothermal fluid equilibrated with siderite (from 4.579 to 10.278‰, see Table 2) were within the oxygen isotopic composition range of magmatic and deep-seated (metamorphic) waters (∼5.5 to 9‰ and ∼5 to 25‰ respectively, see Taylor, 1974). This is consistent with the δ13C values obtained (Table 2), which were also in the range of those of magmatic and deep-seated fluids (∼–9 to –4‰, see Zheng and Hoefs, 1993). Besides, the δ18O values of the fluid equilibrated with quartz were lighter than those of siderite and broadly scattered to the left (from ∼5.3 to –1‰, see Table 2), suggesting a variable involvement of meteoric waters characteristically with δ18O < 0 (see Taylor, 1974).
4.3 Lead isotopes
Galena from three different veins and two samples of the Mesozoic basaltic host rocks were analyzed for Pb isotopes at the Department of Mineralogy, University of Geneva, Switzerland, using a ThermoTRITON mass spectrometer. Lead isotope ratios were corrected for instrumental fractionation by a factor of 0.07% per a.m.u. based on more than 90 measurements of the SRM981 standard and using the standard values of Todt et al. (1984). The external reproducibility of the standard ratios was 0.11% for 206Pb/204Pb, 0.12% for 207Pb/204Pb, and 0.20% for 208Pb/204Pb. Additionally, one galena sample from another vein was analyzed for Pb isotopes at the ACTLABS Laboratories, Canada, with a Finnigan MAT–261 multicollector mass spectrometer. Lead isotope ratios were corrected for mass fractionation calculated from replicate measurements of Pb isotope composition in NBS SRM–982 standards. The external reproducibility of the standard ratios was 0.1% for 206Pb/204Pb and 207Pb/204Pb and 0.2% for 208Pb/204Pb.
The lead isotope composition of galena was found to be variable in 206Pb/204Pb (18.4239 to 18.5012) and 208Pb/204Pb (38.2470 to 38.3192) and different from that of the basaltic host rock (Table 3). In the plumbotectonic diagrams (Fig. 5a and b), the lead from the galena plots very close to the orogen curve.
Lead isotope compositions of galena from different Paramillos de Uspallata veins and the Triassic host rock basalts (whole rock) corrected to 235 Ma.
Vein | 206Pb/204Pb | 207Pb/204Pb | 208Pb/204Pb |
Vallejos | 18.497 | 15.587 | 38.303 |
San Miguel | 18.424 | 15.581 | 38.247 |
Florida | 18.501 | 15.587 | 38.319 |
Tajo | 18.485 | 15.564 | 38.247 |
Basalt | 206Pb/204Pb | 207Pb/204Pb | 208Pb/204Pb |
1 | 18.6859 | 15.599 | 38.5119 |
2 | 18.6229 | 15.6029 | 38.4630 |
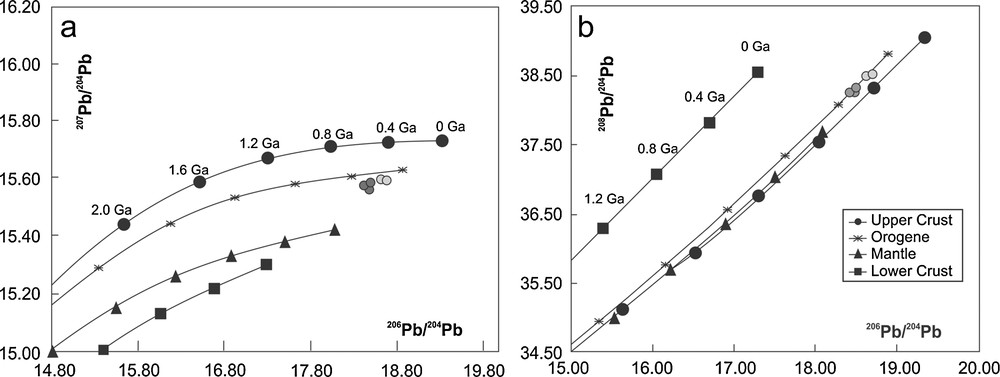
Uranogenic (a) and thorogenic (b) Pb isotope diagrams showing the plumbotectonic curves of Zartman and Doe (1981) and the Paramillos de Uspallata galena composition (gray circles).
5 Discussion
5.1 The descriptive model
The features of the Paramillos de Uspallata vein-type deposit described above plus information provided by previous work were used to outline a new descriptive model (see below).
5.1.1 Age of the mineralization and tectonic setting
Since there are no geochronological data of the Paramillos de Uspallata deposit, the relative age of the mineralization is constrained from field relationships. Mineralized vein strikes are mainly coincident with the NW and WNW normal and sinistral strike-slip faults of Triassic age recognized in the north-central part of the Cuyo Basin (Giambiagi et al., 2011). No crosscutting relations between the Paramillos de Uspallata veins and the Miocene volcanics of the area have been reported, and the veins are cut by north–south thrusts linked to the Andean deformation, which, at that latitude, started by the middle Miocene (Ramos et al., 2002). Based on the age of the host rocks and the structural controls, the deposit could be considered approximately as Middle to Late Triassic and thus contemporaneous with rifting development. In this extensional tectonic setting, the volcanics of the synrift sequence attained sub-greenschist metamorphism (prehnite–pumpellyite facies, ∼250–350 °C) as a result of crustal thinning and raised thermal gradients (diastathermal metamorphism), as proposed for the Mesozoic Neuquén Rift Basin located further south (Rubinstein et al., 2007).
5.1.2 Ore paragenesis and metal ratios
Three stages of mineralization can be recognized at the Paramillos de Uspallata vein deposit (Fig. 4). The early stage consists mainly of Pb–Zn–(Cu) sulfides and has very low Ag contents. The main mineralization stage is composed of Ag–Cu and Pb–(Ag) Sb-bearing sulfosalts and is followed by a late barren stage.
Paragenesis consisting of galena and sphalerite with minor pyrite and chalcopyrite along with diverse sulfosalts, including (Ag)-tetrahedrite and silver minerals in a dominantly siderite and/or quartz gangue, typically occurs in CSS Pb–Zn–Ag veins (see Beaudoin and Sangster, 1992) and particularly in those associated with rift tectonic settings, e.g., base metal veins of the Purcell Basin (Paiement et al., 2012), High Atlas Range (Bouabdellah et al., 2009) and Mesozoic Aulus basin (Munoz et al., 2016). Although the Paramillos de Uspallata deposit has Ag/Ag + Pb (∼0.65) similar to that of CSS Pb–Zn–Ag veins (0.22–0.63), it has a lower Pb/Pb + Zn (∼0.42) than this type of deposits (0.51–0.72) (see Beaudoin and Sangster, 1992).
5.1.3 Physico-chemical conditions and origin of the hydrothermal fluids
According to Garrido et al. (2001), the early-stage mineralizing fluids had temperatures between 160° and 250 °C and salinities that ranged from ∼4 to 19 wt% NaCl eq., and probably carried Mg and Ca. Moreover, the 1.47–14.54 mole percent FeS in sphalerite (see sphalerite composition in Table 1) indicates an intermediate (although variable) sulfidation state for the mineralizing fluids (see Einaudi et al., 2003, and references therein). Besides, the oxygen and carbon isotope composition of the Paramillos de Uspallata gangue minerals is consistent with a magmatic or metamorphic origin for the early stage mineralizing fluids which were mixed with varying proportions of meteoric fluids during the late hydrothermal barren stage.
Around the world, CSS Pb–Zn–Ag veins derive from mixed deep-seated (metamorphic) and meteoric fluids that have a wide range of temperatures (170 to 450 °C) and variable (up to 26%), but characteristically moderate salinities (see Beaudoin and Sangster, 1992). The mineralizing fluids of the Paramillos de Uspallata deposit are within the range of temperature and salinity of the CSS Pb–Zn–Ag deposit. However, although a metamorphic origin of the fluids is consistent with the geodynamic scenario of diastathermal metamorphism associated with the formation of the Cuyo rift basin, the involvement of magmatic fluids in their genesis cannot be ruled out.
5.1.4 Sources of the sulfur and lead
The values for reduced sulfur calculated from the isotopic composition of sphalerite and galena obtained by Garrido et al. (2001) using the equations of Li and Liu (2006) in the sphalerite homogenization temperature range (180 °C–225 °C, see Garrido et al., 2001) vary between 2.2‰ and 11.1‰. This range, which is close to that of the magmatic field (generally between –5 and 7‰, see Seal II, 2006) suggests the involvement of magmatic sulfur, either derived from magmatic fluids and/or leached from the host volcano-sedimentary sequence. The sulfur isotopic composition in different CSS Pb–Zn–Ag veins suggests that it derived from the leaching of the local country rocks or the thermochemical reduction of marine sulfate (e.g., Beaudoin and Sangster, 1992; Bouabdellah et al., 2009; Paiement et al., 2012).
The Pb isotope ratios obtained for galena from different veins of the Paramillos de Uspallata deposit are distinctly lower than those of the host basalts, indicating the involvement of an older (and less radiogenic) source for this metal. In the plumbotectonic diagrams, the samples plot in a range between 320 and 260 Ma (see Fig. 4), which allows suggesting that the arc Permian volcanics of the Choiyoi Group and the underlying Carboniferous marine sedimentary rocks that form the Paleozoic basement of the Cuyo Basin (Cortés et al., 1997; Spalletti, 1999) are possible sources of lead. Moreover, the samples plot between the mantle and the orogen evolution curve, but very close to the latter, as it happens with the Choiyoi volcanics and the genetically related ore deposits (Rubinstein et al., 2004). This is consistent with the Pb isotope mixture signature of the CSS Pb–Zn–Ag veins in which Pb derives from a mixture of rocks that have been stored for a long time in the upper crust and rocks deformed during different orogenic cycles and also with a contribution of Pb from the lower crust and the mantle (Beaudoin and Sangster, 1992; Paiement et al., 2012). Moreover, the lead isotopic composition of galena from the Paramillos de Uspallata plots in the field of the Mesozoic deposits from the Purcell Basin and Coeur d’Alene deposits (Fig. 6), which defines an array resulting from several lead-mixing events during the Mesozoic–Cenozoic tectonics (Paiement et al., 2012).
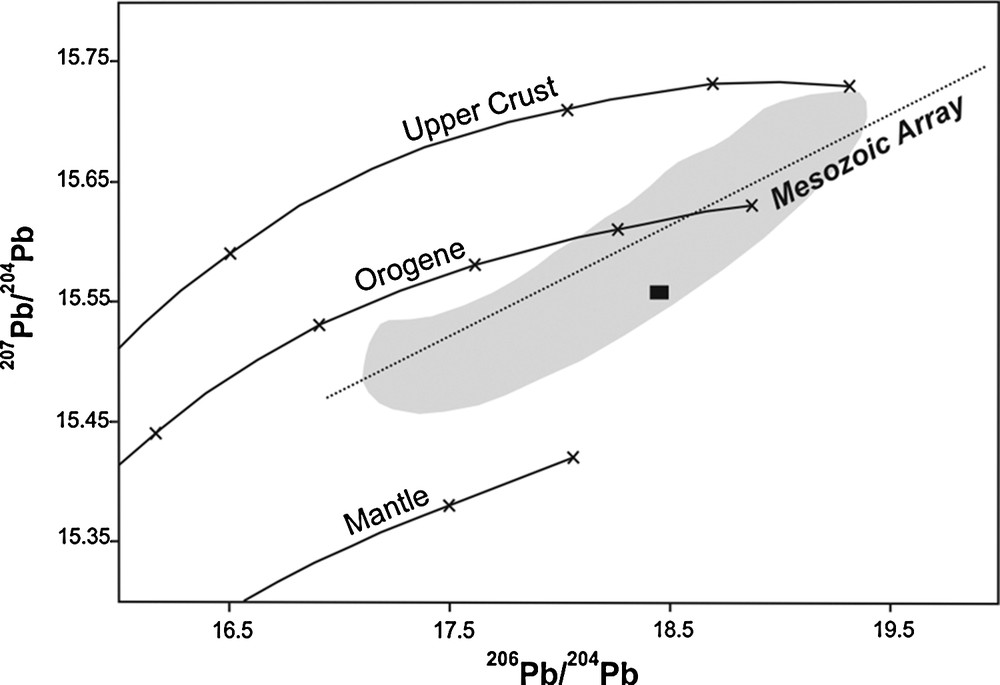
207Pb/204Pb vs. 206Pb/204Pb diagram showing the Mesozoic deposits from the Purcell Basin and Coeur d’Alene district (gray field) and the Paramillos de Uspallata deposit (black field). Modified from Paiement et al. (2012).
5.1.5 The genetic model
Based on the ore and gangue mineralogy and particularly the proximity to the Cu–Mo porphyry and Au-epithermal deposits, Garrido et al. (2001) classified the Paramillos de Uspallata Pb–Zn–Ag veins as a carbonate-base metal and gold system associated with the Miocene porphyry-type deposits of the area (i.e. Cordilleran polymetallic mineralization). However, the stratigraphic relationships and the structural controls clearly contradict this hypothesis. Moreover, the Paramillos de Uspallata Pb–Zn–Ag veins do not display the characteristic base metal zonation (Cu → Zn; Pb → Ag) typical of the Cordilleran veins (Catchpole et al., 2015).
Bearing in mind its tectonic scenario, the Paramillos de Uspallata deposit could be considered as a “detachment-related mineralization”. This deposit model, which was defined by Long (1992) and later expanded by Zappettini et al. (2017), includes Mn bedded and vein deposits, Ba–F veins, Cu–Fe–Pb–Ag–Au replacement and veins, five-element veins, Se-rich polymetallic veins, and Pb–Ag–Zn simple veins. In this model, detachment faults in extensional settings separate an upper cold block with listric and flat normal faults from a lower hot block affected by a metamorphism process. This promotes the circulation of basin brines along the detachment faults toward the normal faults, where they mix with meteoric waters and, under favorable physico-chemical conditions, lead to the deposition of the metals formerly leached from the basin sequence.
The Paramillos de Uspallata deposit is linked to the extensional tectonics that followed the collapse of the Permian orogen and resulted in a rift formation and the consequent rise of the asthenospheric mantle and diastathermal metamorphism that would have triggered the hydrothermal system. Although the results of stable isotopes are consistent with a mixture source of metamorphic and meteoric origin for the mineralizing fluids as in the detachment-related deposits (Zappettini et al., 2017), the involvement of magmatic fluids (and sulfur) cannot be ruled out.
6 Conclusions
The metallogenic analysis of the Paramillos de Uspallata vein deposit shows that this deposit is the result of an early Pb–Zn mineralization stage followed by a Ag–Cu–Pb stage and a late barren stage. The hydrothermal fluids had low temperature, low to moderate salinity, and intermediate sulfidation conditions, and probably resulted from a mix between fluids of metamorphic and meteoric origin. However, the contribution of magmatic fluids cannot be precluded. The most suitable source of lead is the upper Paleozoic basement of the Cuyo Basin, whereas the sulfur derived from magmatic fluids and/or leached from the host volcano-sedimentary sequence.
These characteristics allow describing Paramillos de Uspallata as a CSS Pb–Zn–Ag vein deposit genetically linked to the Cuyo rift basin and therefore redefining it as a detachment-related mineralization. Although this genetic model proposes a mixture of metamorphic and meteoric sources for the mineralizing fluids, the involvement of magmatic fluids cannot be ruled out.
Acknowledgements
We gratefully acknowledge Dr. Massimo Chiaradia (University of Geneva, Switzerland) for his support in the Pb isotope analyses and Dr. Georges Beaudoin (Université Laval, Canada) for his support in the O isotope analyses. Constructive comments by Dr. F. Tornos and Dr. E. Zappettini helped to eliminate inconsistencies and improve the clarity of this paper.
This work was supported by the National Scientific and Technological Promotion Agency, Argentina (PICT ANPCyT 1280-2014), the National Sciences and Technology Council, Argentina (PIP CONICET 112 201301 00107) and University of Buenos Aires (UBACyT 20020130300029BA).