1 Introduction
Relative sea-level fluctuations, climate change and human impacts are well recorded in the sediments of coastal marshes and wetlands (e.g., Edwards, 2007; Giraudi, 2011; Kemp et al., 2011). Coastal marshes and lagoons are particularly sensitive to local and regional palaeoenvironmental changes and have proven to be powerful archives for studying coastal changes during the Holocene (e.g., Anthony, 2008; Clave et al., 2001; Cole and Liu, 1994; Ejarque et al., 2016; Sacchi et al., 2014; Scott and Medioli, 1978; Stanley and Warne, 1994; Vella and Provansal, 2000). Many palaeoenvironmental studies in the Aegean Sea are based on sedimentary archives extracted from coastal marshes and lagoons (e.g., Evelpidou et al., 2012a; Pavlopoulos et al., 2010; Triantaphyllou et al., 2010; Vouvalidis et al., 2010). Using a multi-proxy approach based on sedimentology, micro- and macrofauna identification and dating, it is possible to reconstruct the coastal evolution and relative sea level changes (e.g., Ghilardi et al., 2014; Marriner et al., 2014; Nixon et al., 2009; Primavera et al., 2011; Vacchi et al., 2017).
Our study area, Paros Island (Cyclades), has rich evidence for human occupation; however, there is a dearth of data with regards to the evolution of the island's seaboard palaeoenvironments. Palaeogeographical reconstructions are fundamental to better investigate coastal archaeological sites and to provide the basis for archaeologists regarding past human – environment interactions. In this context, the primary aim of this study is to probe the evolution of the coastal zone in Paroikia bay, Paros Island (Cyclades), and to reconstruct the palaeoshoreline changes during the late Holocene.
2 Study area
2.1 Physical setting
Paros Island is part of the Cyclades Islands complex, in the central Aegean Sea. It is the third largest island of the Cyclades (Fig. 1). The Cycladic region is presumed to be under the influence of an extensional tectonic regime behind the modern volcanic arc at the centre of the Aegean plate and possesses a relatively thin continental crust about 25–26 km in thickness (e.g., Tirel et al., 2004; Zhu et al., 2006). The central Aegean is characterized by relatively low seismicity and the absence of large earthquakes (e.g., Papazachos, 1990). According to Sakellariou and Galanidou (2016), vertical tectonic movements have been considered of minor significance for the palaeogeographical evolution of this area during the late Pleistocene-Holocene.
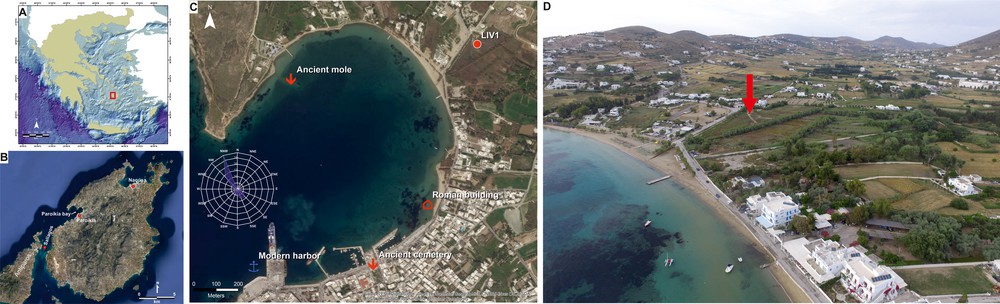
Location of the study area and drilling site: a) Location of Paros Island within the Aegean; b) aerial photograph of Paros Island (Google Earth); c) location of the core along with the main submerged archaeological remains in Paroikia Bay (wind rose from windfinder.com, Image Google Earth); d) aerial view of the coring site in Livadia (the red arrow shows the coring location).
Several geomorphological and geoarchaeological investigations have been conducted in the Cycladic region to evaluate the RSL changes in the area (e.g., Baika, 2008; Desruelles et al., 2009; Evelpidou et al., 2012a, 2012b; Evelpidou et al., 2014; Kapsimalis et al., 2009; Karkani et al., 2017; Pavlopoulos et al., 2011; Poulos et al., 2008). For Paros Island, Evelpidou et al. (2014) have reported the presence of submerged tidal notches and suggested three palaeoshorelines at −35 cm, −170 cm and −230 ± 20 cm. The occurrence of tidal notches at various depths reveals the occurrence of rapid subsidence events, potentially of seismic origin (Evelpidou et al., 2014).
Lithologically, Paros Island is characterized by a variety of metamorphic rocks (with the dominance of marble, gneiss, and schists) along with some Neogene and Quaternary formations covering only a small part of its coastal zone. The coastal zone of Paros mainly consists of Quaternary deposits, marbles, gneiss schists, and some minor outcrops of igneous rocks. It is mainly rocky and steep, particularly in the northeastern and northwestern part, and the beaches form a smaller part. The studied site, Livadia, is located in the northwestern part of Paros Island, at an alluvial plain bounded by two faults of NNE–SSW and east-west direction, respectively (Figs. 1 and 2).
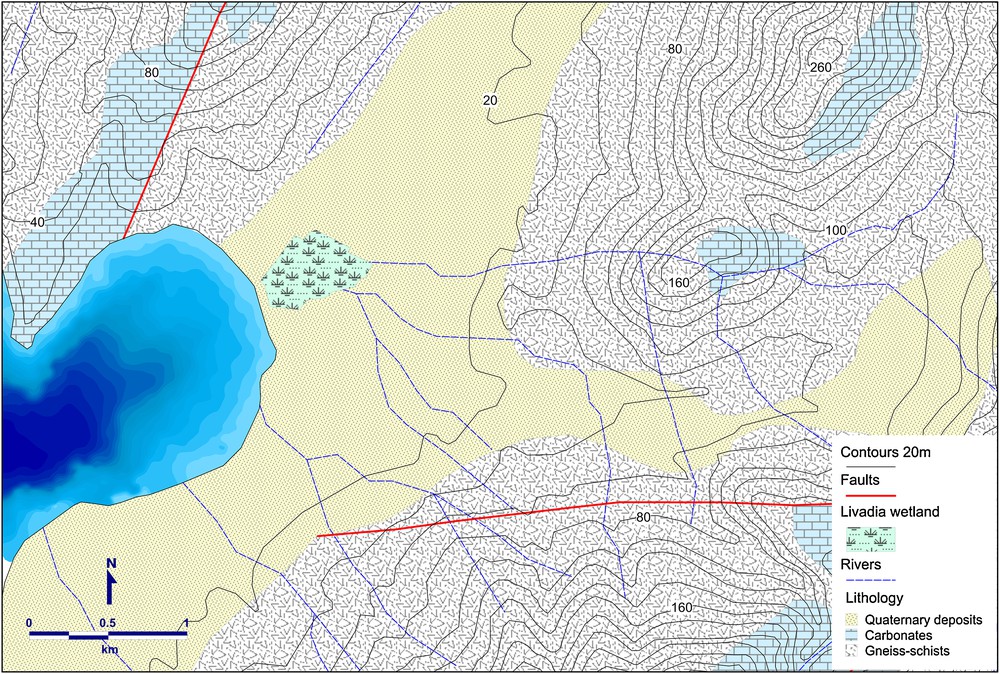
Simplified geomorphological map of the study area. Bathymetry is based on the bathymetric map of the Paroikia harbour, scale 1:5000 (Hellenic Navy Hydrographic Service, 1989), whereas geological data are based on the geological map of Paros (IGME, 1996).
2.2 Archaeological context
Paros Island is of great geoarchaeological significance, with the earliest evidence of occupation dating back to the 5th millennium BC (Schilardi, 2002), while the nearby island of Saliagos has yielded a Neolithic settlement (Evans and Renfrew, 1968). In antiquity, Paros had two renowned harbours, one of which according to Scylax (Periplus 58: "... Πάρος λιμένας ἔχουσα δύο, ὧν τὸν ἕνα κλειστόν") was ‘closed’, i.e. situated within the city walls (Papathanassopoulos and Schilardi, 1981). Due to its sheltered harbours and the island's position in the Aegean, along with the marble trade, Paros became a rich maritime power.
The submerged antiquities of Paros include cemeteries of various time periods, harbour installations and buildings. The bay of Paroikia, in particular, is characterized by the presence of coastal and submerged archaeological remains (Fig. 1c), some of which remain undated (Papathanassopoulos and Schilardi, 1981). In particular, remains of a submerged Roman building have been reported by Rubensohn (1901, 1949) and later by Papathanassopoulos and Schilardi (1981). During an underwater survey by Papathanassopoulos and Schilardi (1981), an ancient mole was found between −2 and −3 m, with a length of ∼100 m. However, no chronological indices were found. The ancient cemetery in the bay of Paroikia was in use from the end of the 8th c. BC until the 3rd–4th c. AD (Zapheiropoulou, 2000). Although the island has been the object of geomorphological and archaeological research, no late Holocene palaeogeographical reconstructions have been undertaken.
3 Materials and methods
3.1 Fieldwork
For the purposes of this study, a multiproxy analysis was undertaken, which included sedimentological and biostratigraphic analyses of the core. The borehole was drilled with a portable drilling sampler, 35 mm in diameter. Here, we present the results from Livadia coastal zone (LIV1), which reached a depth of 4.46 m b.s.l. (below sea level).
Core LIV1 was drilled on a coastal plain (Figs. 1d and 2), 1.2 km to the north-northeast of Paroikia. The plain is composed of Quaternary alluvial deposits, surrounded mainly by gneiss and schists. The site of the coring is, nowadays, a coastal marsh of brackish water (water salinity brackish: 5.0–18.0 g/l; WWF, 2013). It is supplied with fresh water by an aquifer and through an intermittent stream.
3.2 Sedimentological analyses
The analyses took place at the CNRS laboratory facilities of the “Chrono-environnement” lab (université de Franche-Comté, Besançon, France). Before sampling for grain size analysis, the core was studied and photographed in detail in order to record the general stratigraphy. Samples were oven-dried at 50 °C, and dry sediment aggregates were weighed and washed through two mesh sizes, 2 mm and 50 μm, to separate out the gravel (> 2 mm), sand (2 mm to 50 μm) and silt/clay (< 50 μm) fractions.
3.3 Biostratigraphy
The gravel fraction of the sediments was examined to identify mollusc shells and determine their ecology. The identification and classification was accomplished based on d’Angelo and Gargiullo (1978) and Doneddu and Trainito (2005). The species were assigned to ecological groups defined by Pérès and Picard (1964) and Pérès (1982).
Ostracods were extracted from the dry sand fraction (> 150 μm). A minimum of 100 valves was identified and the identified taxa were assigned to five assemblages based on their ecological preferences: fresh water, lagoonal, marine lagoonal, coastal and marine (Lachenal, 1989; Marriner et al., 2012; Nachite et al., 2010; Salel et al., 2016).
3.4 Radiocarbon dating
The chronostratigraphy of the cores was determined by a series of five AMS radiocarbon datings on marine shells, performed at the Poznan Radiocarbon Laboratory (Poland). The samples were corrected for the local marine reservoir effect according to Reimer and McCormac (2002), using a mean ΔR value of 154 ± 52 for the Aegean Sea (Reimer and McCormac, 2002). The radiocarbon ages of the samples were calibrated by using Calib 7.10 (Stuiver et al., 2016) with the Marine13 curve (Reimer et al., 2013). The radiocarbon ages are expressed in calibrated years BP and BC at 95% probability (2σ).
4 Results
4.1 Dating results
Table 1 presents the results of the radiocarbon dating. Two samples (Poz-81150 and Poz-81151) provided ages that appear inconsistent with the stratigraphic order and the ages of the other samples.
Radiocarbon ages for dated samples from Paros cores.
Sample code |
Lab code | Depth below sea level (cm) |
Material | δ13C | 14C BP | Age cal. BP | Cal. BC/AD (2σ) |
LIV1-1 | Poz-81150 | 150–160 | Cerithium vulgatum | 4.3 | 3630 ± 30 | 3193–3508 | 1559–1244 BC |
LIV1-2 | Poz-81151 | 235–245 | Bittium reticulatum | −1.4 | 3470 ± 30 | 2985–3330 | 1381–1036 BC |
LIV1-3 | Poz-81156 | 284–296 | Pirinella conica | −7 | 2985 ± 35 | 2385–2729 | 780–436 BC |
LIV1-4 | Poz-81157 | 360–370 | Pirinella conica | 2.7 | 3110 ± 30 | 2559–2872 | 923–610 BC |
LIV1-5 | Poz-81192 | 420–430 | Hydrobia | −5.9 | 4670 ± 50 | 4500–4864 | 2915–2551 BC |
4.2 Sedimentological and biostratigraphic results
LIV1 core reached a depth of 4.46 m b.s.l. Based on the sedimentological and faunal analysis, four units were distinguished.
4.2.1 Unit A1: semi-enclosed lagoon under fluvial influence
This unit was observed from the base of the core up to 2.84 m b.s.l., dominated by grey silts (Figs. 3 and 4). The silt/clay fraction represents almost 77% of the total sediment texture up to 3.85 cm, and between 3.75 and 2.84 m it is reduced to 45%. Lagoonal (Cyprideis torosa) and freshwater ostracods (Illyocypris gibba, Heterocypris salina, Darwinula stevensoni, Candona lactea) dominate this unit. Lagoonal species increase towards the upper part from 33.4 to 54% of the total assemblages. Marine lagoonal (Loxoconcha rhomboidea) and coastal species (Urocythereis oblonga, Pontocythere elongata, Aurila convexa, Aurila woodwardii) are only present in the upper part of the unit, representing 2.5 and 7.8% of the total assemblages, respectively (Fig. 3).
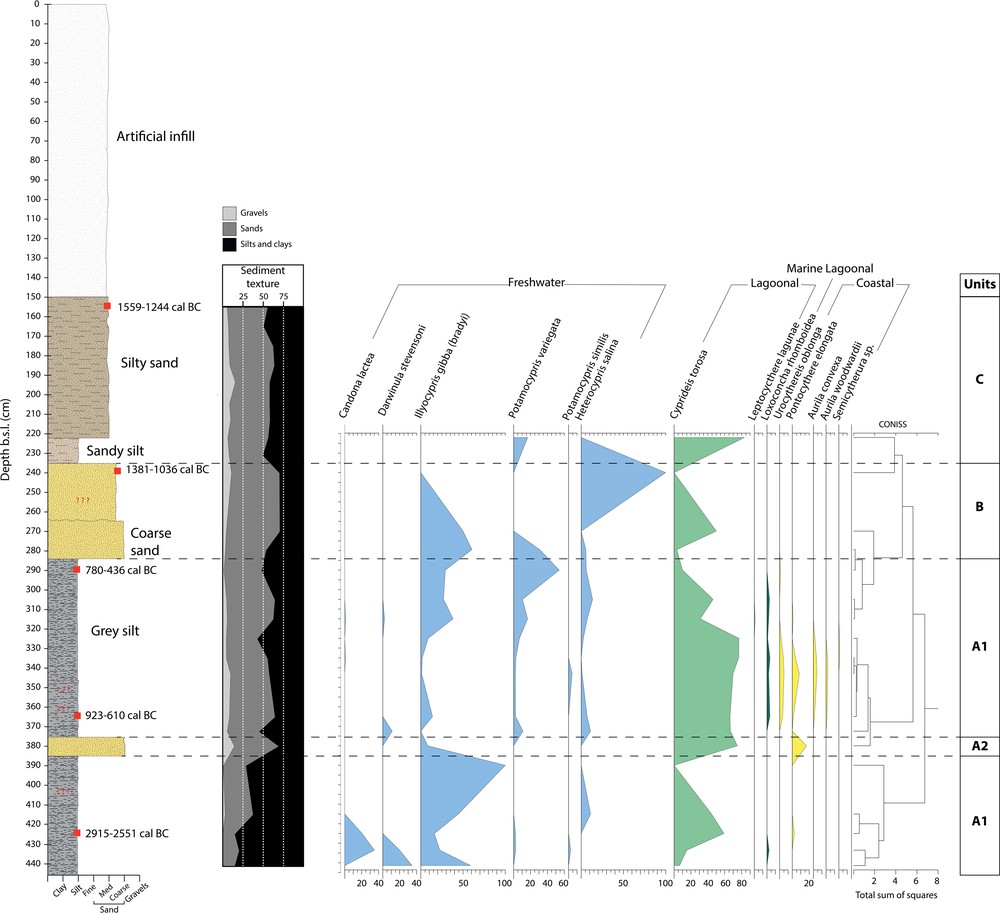
Core LIV1 stratigraphy along with ostracod species and assemblages.
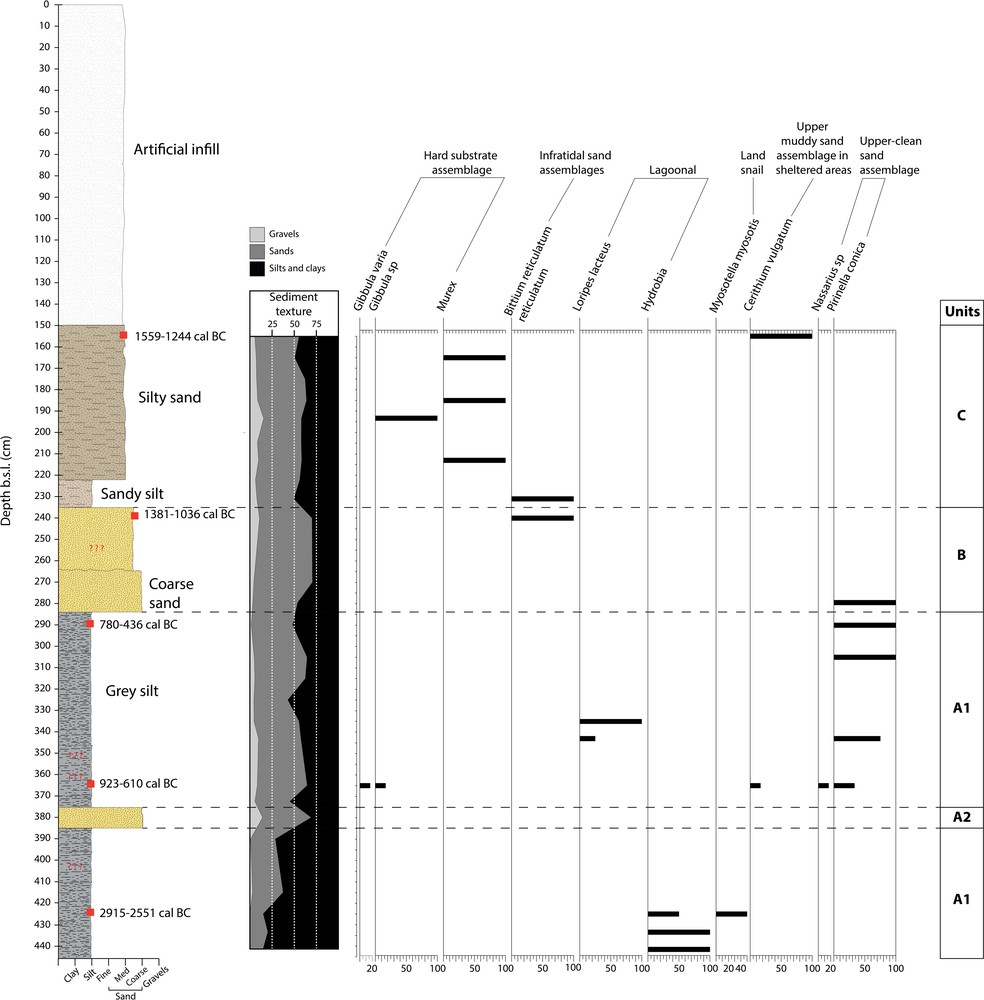
Mollusc species and assemblages in Core LIV1.
The molluscan fauna is poor, with only a few individuals of the lagoonal species Hydrobia sp., upper clean sand assemblage species (Pirinella conica, Nassarius sp.), and a few hard substrate species (Gibbula varia, Gibbula sp.) and upper muddy sand assemblage in sheltered areas species, represented by Cerithium vulgatum, in the upper part of the unit (Fig. 4). The age of unit A1 was determined using three radiocarbon dates of marine shells. They indicated an age of 2915–2551 cal BC (4500–4864 cal BP) for the lowermost part, and 780–436 cal BC (2385–2729 cal BP) for the uppermost part. The middle part was dated to 923–610 cal BC (2559–2872 cal BP). The low species diversity along with the presence of typical lagoonal species suggest this unit is representative of a semi-enclosed lagoon. The presence of freshwater ostracods further suggests a freshwater influence from a nearby stream.
4.2.2 Unit A2: input of coarser sediments – high energy event?
This unit consists of a 10-cm layer of coarse sand with some gravels, between 3.85–3.75 m b.s.l. (Figs. 3 and 4). The sand fraction represents 54.8% of the total sediment texture, gravels 14.4% and silt/clays 30.8%. This unit is barren of any molluscan fauna, while the ostraco-fauna shows low densities. They are characterized by a peak in the coastal Pontocythere elongata (17%), a relative decrease in freshwater species (8%) and an increase in Cyprideis torosa (75%). Considering the chronostratigraphy of Unit A1, this unit is older than ∼923–610 cal BC (2559–2872 cal BP).
4.2.3 Unit B: freshwater influenced lagoon
The unit, found between 2.84 m and 2.35 m b.s.l., consists of coarse-medium sands (Figs. 3, 4). Sands represent 58.7% and silt/clays 35% of the total sediment texture. It is barren of molluscan fauna, except at the top of the unit, where a few individuals of an infratidal sand species (Bittium reticulatum) are identified. The ostracods are dominated by freshwater (Illyocypris gibba, Heterocypris salina, Potamocypris variegata) (82.2%) and lagoonal species (Cyprideis torosa) (17.8%), with the absence of any marine lagoonal or coastal species. Species richness generally decreased towards the top of the unit. The top of Unit B was dated to 1381–1036 cal BC (2985–3330 cal BP). However, this date is older than the ages obtained in Unit A1 and appears inconsistent with the stratigraphic order (maybe reworked by fluvial processes). The low species diversity, the abundance in freshwater ostracods, along with the lagoonal species Cyprideis torosa is probably indicative of a local stream/river influence in the lagoon.
4.2.4 Unit C: infilling of the lagoon
Unit C occurred between 2.35 and 1.5 m b.s.l. (Figs. 3 and 4). The base of the unit is dominated by sandy silts with charcoal and the upper part by silty sands with charcoal and traces of oxidation. Ostracods are only present in the sandy silt layer, represented by a few individuals. The lagoonal species Cyprideis torosa represents 83% of the total assemblage while freshwater species comprise Potamocypris variegata (17%). Molluscan faunas are poor in unit C, mainly characterized by broken pieces of hard substrate assemblage species (Murex, Gibbula sp.). The top of the unit was dated to 1559–1244 BC (3193–3508 cal BP). This date, however, is significantly older than expected, and furthermore is not in stratigraphic order in relation to deeper samples. This unit is probably indicative of the gradual infilling of the lagoon.
5 Discussion
The drilling site at Livadia is located a few hundred meters from the remains of a submerged Roman building, a submerged mole (Papathanassopoulos and Schilardi, 1981; Rubensohn, 1901) and the ancient cemetery of Paroikia (Zapheiropoulou, 2000) (Fig. 1c). In 1979, the remains of the Roman building were found 1 m offshore at a depth of 0.5 m below sea level (Papathanassopoulos and Schilardi, 1981). The submerged ancient mole was found between −2 and −3 m, but, unfortunately, remains undated. For the ancient cemetery, no information is available concerning the precise elevation of the excavation; however, it is located below the level of the sea and unless the water is constantly drained, the excavation area turns into a swamp within a few hours (Zapheiropoulou, 1990, 2000). The only available datum, at present, is the depth of the Late Roman cemetery, which extends to the west of the Archaic and Classic one (Zapheiropoulou, 1990). The graves in the Late Roman cemetery were marble sarcophagi placed on high marble pedestals, which are dated to the 3rd c. AD; according to archaeologists, they were found at a depth of −1.50 m (below modern ground level) (Zapheiropoulou, 1990). Taking into consideration the elevation of the ground level (Topographic map of Paroikia Port, 1999) and the depth of the sarcophagi, the current elevation of the ancient cemetery may be estimated at about ∼30–40 cm below sea level.
Overall, the archaeological remains in Paroikia Bay suggest a subsidence regime for the study area. This observation is further supported by the presence of submerged tidal notches, which indicates that at least part of this observed subsidence is related to vertical seismic displacements (Evelpidou et al., 2014). Three submerged palaeoshorelines have been reported for Paros Island by Evelpidou et al. (2014), related to palaeoearthquakes that have taken place since ∼3300 BP, and shorelines at −35 and −170 cm have been noted near the Livadia coring site to the southwest. No differential tectonic movements have been noted by Evelpidou et al. (2014) for the island of Paros based on the layout of the observed notches.
The results from the Livadia core offer new elements to reconstruct the main phases of shoreline development in the northern part of Paroikia (Fig. 5a, b). From at least 2915–2551 BC (4500–4864 cal BP) until ∼780–436 BC (2385–2729 cal BP), the area was a semi-closed lagoon with fresh water input, as attested by the presence of freshwater microfauna (∼51% on average). This lagoon must have been almost continuously affected by the presence of a local stream. In fact, a closer examination of the river network of the area shows that two third-class streams discharge into the bay of Paroikia.
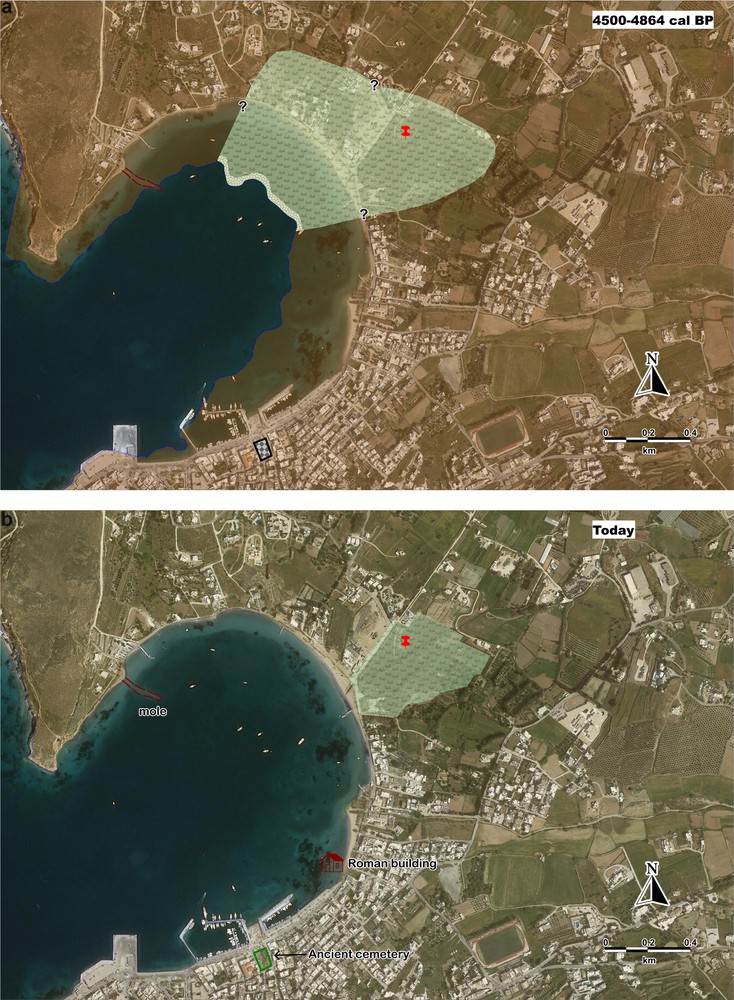
Palaeogeographical evolution of Livadia since 2915–2551 BC in relation to present-day geography (the red pin shows the LIV1 core location): a) The coring site used to be a semi-enclosed lagoon from at least 2915–2551 BC until ∼780–436 BC, occasionally influenced by a local stream; b) the present-day coastal landscape (background from Google Earth).
This environment appears to be interrupted by an input of coarser sediments, which is attested to by the presence of a 10-cm-thick layer of coarse sand with gravels. This event is probably dated to before 923–610 BC (2559–2872 cal BP). The coarse sand layer bears no evidence of molluscan fauna. However, ostracod faunas are characterized by a peak in the coastal Pontocythere elongate, and freshwater ostracods are poorly represented in comparison to Unit A1. The transition from fine (unit A1) to coarser deposits (unit A2) correlated with the increase in the proportion of coastal ostracods in A2 may indicate reworking during a possible storm episode. The input of sand along with gravels, considering the contemporary beach sediments, may have originated from the coast. Sedimentological and faunal evidence indicates a high-energy event, i.e. a storm. However, in many occasions, the sedimentological characteristics of these high-energy events are similar, and it is not always possible to distinguish them (e.g., Kortekaas and Dawson, 2007; Morton et al., 2007). Following this event, the lagoon barrier appears to be more frequently breached, as attested to by some coastal macrofauna along with marine lagoonal and coastal ostracods.
After ∼780–436 BC (2385–2729 cal BP), the absence of any coastal-marine molluscs and the dominant presence of freshwater ostracods suggest that the lagoon is primarily affected by fluvial processes. These processes are not evidenced in the last unit of the core (Unit C), and sedimentological and faunal observations probably reflect the gradual infilling of the lagoon. The presence of a few ostracod species, limited only to the lower part of unit C, along with the presence of only broken pieces of hard substrate assemblages, may reflect the gradual infilling of the lagoon. Charcoal fragments probably indicate burning, while pedogenesis may be suggested by traces of oxidation in the unit. Although it is not possible to chronologically constrain the timing of this change, it may be relatively young, testifying to anthropogenic impacts.
In order to provide insights into the RSL changes in the study area, RSL index points were produced from the dated samples of this study (Table 1). To produce RSL index points, we considered an indicative range from 0 to −1 m for samples deposited in semi-enclosed lagoons (e.g., Vacchi et al., 2016). The archaeological data from Paroikia bay were used as terrestrial limiting points, as their relationship to sea level cannot be accurately defined. We compared our results with the RSL reconstruction by Desruelles et al. (2009) from the wider region (Fig. 6). For Paros Island, Papathanassopoulos and Schilardi (1981) estimated an RSL of 2–3 m at about 2500–2900 BP, while Morrison (1968) estimated a RSL rise of ∼5.5 m since 5500 BP.
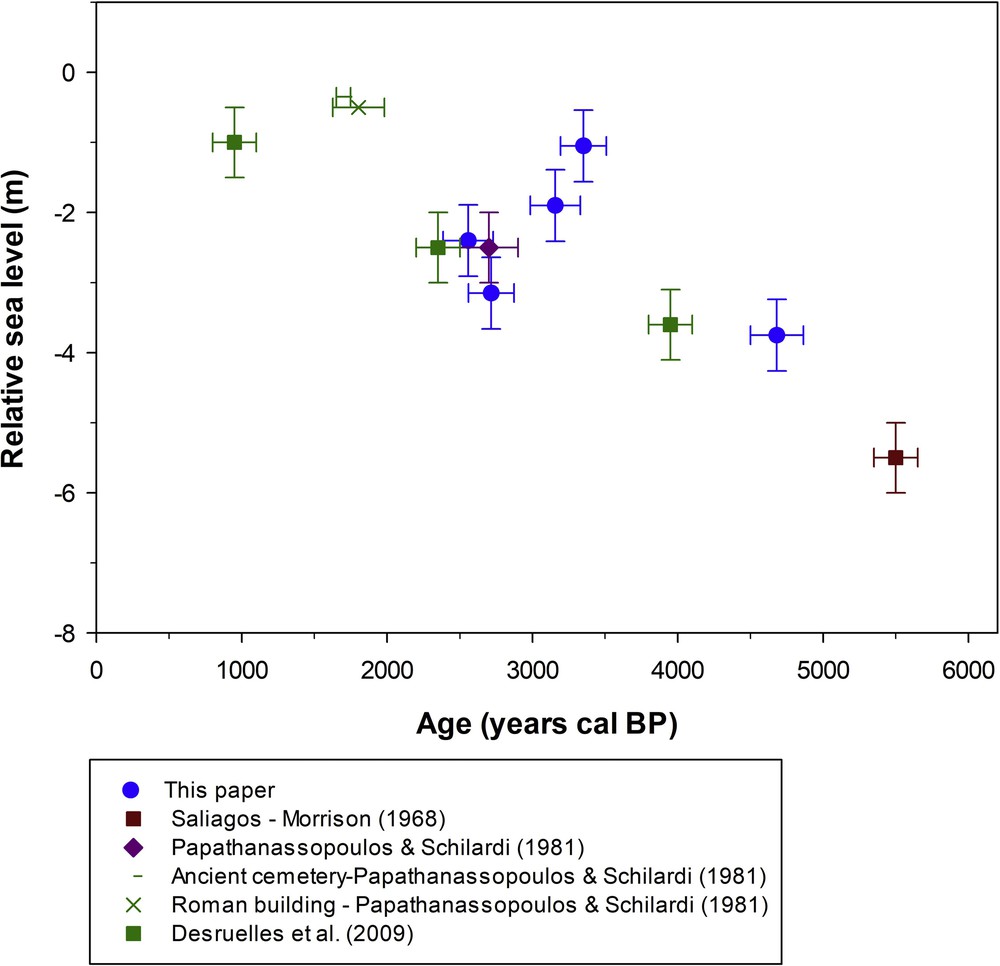
Relative sea-level changes in Paros Island. The produced RSL index points are plotted in relation to the RSL curve by Lambeck and Purcell (2005).
According to Lykousis (2009), a continuous subsidence rate has dominated during the last 400 ka, quantified to 0.34–0.60 mm/a for the Cycladic plateau, with a gradual decrease in the magnitude of the extensional tectonic regime. At the same time, the presence of submerged tidal notches at various depths in Paros and neighbouring islands (Naxos, Sifnos, Iraklia, Keros) indicates that at least part of this observed subsidence is related to vertical seismic displacements (Evelpidou et al., 2014, Fig. 3).
As already noted, Paros was a rich maritime power, because of its sheltered harbours, and the island's position in the Aegean, along with the marble trade. However, its harbours remain to be found. Understanding the evolution of coastal landscapes and palaeoshoreline reconstructions may provide the basis for further geoarchaeological studies.
6 Conclusions
Multiproxy analysis of a coastal core allowed us to reconstruct the coastal landscape evolution of Paroikia Bay (Paros Island, Greece) during the late Holocene. Based on sedimentological and palaeontological data, a semi-enclosed lagoon existed in the northeastern part of Paroikia Bay from at least 2915–2551 BC. The lagoon appears to have been gradually infilled after 780–436 BC, possibly influenced by anthropogenic factors. The reconstructions of RSL changes in correlation with archaeological and geomorphological data from Paros Island suggest a subsidence regime, probably composed of long-term factors in combination with vertical seismic displacements. Our study further highlights the significance of understanding the evolution of coastal landscapes and palaeoshoreline reconstructions as the basis for further geoarchaeological studies.
Acknowledgements
The authors would like to thank Alexandros Petropoulos, Giannis Saitis, Theophilos Valsamidis, Matina Seferli, and Electra Kotopoulou for their help during fieldwork in Paros. We also thank Manolis Lykouropoulos for the aerial photography. We would also like to thank also Dr. Friederike Stock and Dr. Dorit Sivan, whose constructive comments improved an earlier version of this paper.
This work was co-funded by the General Secretariat for Research and Technology (GSRT) and the European Regional Development Fund, in the framework of the Bilateral project Greece–France entitled: ‘Sea-level changes in Cyclades’.
This work has been partially carried out thanks to the support of the Labex OT-Med (ANR-11-LABX-0061) and of the A*MIDEX project (No. ANR-11-IDEX-0001-02), funded by the “Investissements d’Avenir” program of the French Government, managed by the French National Research Agency (ANR).