1 Introduction
Natural aluminium (Al) is a well-known refractory element (the third most abundant element in the earth crust, just behind oxygen and silicon elements) not easily labile (Belhadj et al., 2017). However, anthropological activities also release aluminium in the environment. For instance, water treatments use aluminium salts that are subsequently found in natural waters. The use of aluminium sacrificial anodes is another source of anthropogenic aluminium, mainly in marine environments. The anodes enable the protection of the immersed steel structure by cathodic protection. This process involves an electrical connection between the submerged or buried metallic steel structures to protect and a block of aluminium, which behaves as an anode, so that the steel behaves as a cathode and the corrosion is strongly delayed (Leleyter et al., 2007).
As aluminium is more stable in solid phase than in aqueous phase, aluminium ions poured in natural waters tend to be sorbed onto surrounding sediments (Gabelle et al., 2012; Pineau et al., 2008), which then could act as a sink of aluminium to biota. Many studies have proved that the growth of plants and aquatic biota in acidic ecosystems is largely affected in Al-contaminated environments (Leleyter et al., 2016; Lu et al., 2011; Meiri et al., 1993; Platt et al., 2001).
Contamination by heavy metals in sediments is often assessed by the determination of their total contents and comparison with either established national guidelines or some background reference values (Baraud et al., 2017; Chand and Prasad, 2013; Covelli and Fantolan, 1997; Dubrulle et al., 2007; Giancoli Barreto et al., 2004; Leleyter and Baraud, 2005; Meybeck et al., 2007). However, metal concentrations derived from these total digests do not necessarily provide biologically meaningful data. The alternative is to use a partial extraction that only targets the labile mineral phases, as these are most likely to exert an influence on biota (Scouller et al., 2006; Tam et al., 1989). Many chemical extractions procedures have been proposed in the literature, to estimate the mobility of metals in soil and sediments, or their bioavailability defined as the capacity of an element to be transferred from a soil fraction to a living organism, regardless of the mechanism.
Thus, some studies focused on the potential mobility of the heavy metals in sediments, estimated by either simple (HCl or EDTA) or sequential extractions (several steps) procedures (Alvarenga et al., 2014; Baraud and Leleyter, 2012; Devesa-Rey et al., 2010; El Azzi et al., 2013; Hamdoun et al., 2015a, b; Islam et al., 2015; Leleyter and Probst, 1999; Leleyter et al., 2012; Rousseau et al., 2009; Roussiez et al., 2013; Sutherland, 2002; Tessier et al., 1979). These chemical extraction procedures should be efficient (to solubilize all the elements in sediments which could be naturally solubilized due to changes in the physicochemical properties of the aquatic environment) and selective (the residual metals, that are associated with very stable fractions of sediments, should not be mineralized during the extractions).
For marine sediments, HCl (1 M) extraction is recommended by many authors (Burton et al., 2005; Doherty et al., 2000; Hamdoun et al., 2015a, b; Larner et al., 2008; Leleyter et al., 2012; Peña-Icart et al., 2014; Scouller et al., 2006; Snape et al., 2004), as HCl is assumed to extract heavy metals thanks to its acidic properties combined with the chelatant property of Cl−. Moreover, sequential extraction procedures, which present the advantage of characterizing the different labile fractions and thus to be more selective and specific, are recommended by many authors too (Leleyter and Baraud, 2006; Leleyter and Probst, 1999; Shuman, 1985; Tessier et al., 1979; Ure et al., 1995).
For a comprehensive environmental risk assessment, both approaches (total and labile contents) are generally complementary to fully characterize the degree of metallic contamination of sediments. However, detecting anthropogenic aluminium contamination in sediments could be especially difficult. Indeed, the usual trace metal contaminations studies (using background references comparisons) could not be easily transposed to the study of a major element such as aluminium, because anthropological quantities are often negligible compared to the natural amount. First tests (Gabelle et al., 2012; Leleyter et al., 2012) on natural sediments exhibited some differences in the mineralogical partitioning of natural and anthropological aluminium: partial extractions appear as the only chemical procedures to use to highlight aluminium contamination.
To study aluminium fractionation speciation in sediment, a natural marine sediment (noted SL) was collected. Some aliquots of SL sediments were contaminated by aluminium salts or by aluminium sacrificial anode. Then the total contents of aluminium in the contaminated or natural sediments were determined as well as the Al mobility, estimated by single (HCl) and sequential (Leleyter and Probst, 1999) extractions.
2 Materials and methods
2.1 Sampled sediments
The natural sediment (noted SL) is collected on a weakly urbanized zone (Luc-sur-Mer, Calvados, western France). Two cans of 10 L of sediments are taken to the marine research station (380 m from the sea coast at Luc-sur-Mer), air-dried and sieved on a < 1 mm mesh. Approximately 8 kg of the obtained sediments are boorishly crushed and homogenized. Then, a small part (200 g) of the sediments is crushed in a mortar (agate), homogenized, and stored at 4 °C. This sediment was characterized by a high percentage of calcium carbonate (evaluated at 33% in the dried sediments, due to the erosion of marine shells collected with sediments) and the presence of different clays: kaolinite (44% of the clay fraction), smectites (31%), illite (19%), chlorite (4%), and interstratified layers of illite and chlorite (2%) (for details, see Gouali, 2013).
2.2 Al total concentration
The total concentrations of Al were determined by ICP–AES (Inductively Coupled Plasma–Atomic Emission Spectrometry, Varian, Vista MPX) following sample dissolution using alkaline fusion (procedure NF ISO 14869-2, AFNOR, 2002). 0.2 g of dried sediment was thoroughly mixed with 0.8 g of lithium metaborate and 0.2 g of lithium tetraborate in a Pt crucible. The mixture was heated at 1000 °C for 45 min, and then the fusion product was immediately put in 60 mL of 1 mol·L−1 HNO3 solution until total dissolution of the residue occurred. The recovered solutions were made up to a volume of 100 mL with 1 mol·L−1 HNO3 solution and analysed by ICP–AES.
To check the efficiency of the process, a standard harbour sediment reference (HR1) and a standard marine sediment reference (PACS2) were analysed (three replicates), and have proved the efficiency of the mineralisation process and the accuracy of the chemical analyses (Gabelle et al., 2012).
2.3 Single and sequential extraction techniques
1 mol·L−1 HCl single extraction and one sequential extraction procedure (Leleyter and Probst, 1999) are performed.
1 mol·L−1 HCl single extraction is commonly recommended to estimate the content of labile metals in marine sediments (Burton et al., 2005; Larner et al., 2008; Leleyter et al., 2012; Scouller et al., 2006; Snape et al., 2004; Townsend et al., 2009). 1 g of dry sediment is mixed with 10 mL of a 1 mol·L−1 HCl solution under agitation at room temperature for 1 h. The resulting mixture is centrifuged (3000 rpm; 2 min), filtered at 0.45 μm, and the filtrate is analysed using ICP–AES.
Leleyter and Probst's (1999) sequential extraction method was chosen (for details, see Leleyter and Baraud, 2006; Leleyter and Probst, 1999; Leleyter-Reinert, 2017) among several procedures, as this procedure was checked for selectivity, reproducibility, and repeatability of the different steps, and was commonly used in the literature (Bur et al., 2009; Cecchi et al., 2008; N’guessan et al., 2009; Salvarredy-Aranguren et al., 2008). This chemical extraction dissolves, in selective and efficient ways, all the chemical constituents of sediments in the following order: F1, water soluble fraction (released by ultrapure water); F2, exchangeable fraction (extracted with a magnesium nitrate solution); F3, acid-soluble fraction (leached by an acid/acetate buffer); F4, reducible fraction (extracted with hydroxyl ammonium, oxalic acid and ascorbic acid); F5, oxidisable fraction (released by hydrogen peroxide-ammonium acetate extraction). F6 is the sum of all labile fractions (F6 = ΣF1 to F5).
2.4 Laboratory contamination tests
2.4.1 Salts contamination
As the most frequently salts used in treatments of drinkable waters in the coagulation/flocculation process are aluminium polychloride and aluminium sulphate (Takaoka et al., 2015), contamination tests were run with these two common aluminium salts according to the following protocol. Aluminium sulphate (Al2(SO4)3·18 H2O) or aluminium chloride (AlCl3·6 H2O) are added to an artificial sea water solution, to get an aluminium concentration of 200 μg·L−1 (which is the reference for drinking waters according to the French “arrêté” of 11 January 2007). Then 10 g of sediment were mixed with 30 mL of z saline solution (aluminium sulphate or chloride) under agitation at room temperature for 24 h. The resulting mixture is centrifuged (3000 rpm; 2 min) and residual are dried (40 °C), and noted SLsulfate or SLchloride (respectively SL contaminated by aluminium sulphate or aluminium chloride solutions). The pHs of the solutions are measured. The obtained values for the initial and final solutions remain stable and equal to 8.0.
2.4.2 Sacrificial anode contamination
Contamination tests by an aluminium sacrificial anode (details in Gouali, 2013) are performed in a 1-L thermostatic cell (20 °C) by means of a classic electrochemical experiment with three electrodes–aluminium alloy working electrode (5.2 cm2), reference saturated calomel electrode, and platinum counter-electrode (46.1 cm2). In the cell, 100 g of sediments and 700 mL of artificial sea water (ASTM) are placed. A positive current of 0.1 A is imposed for 24 h. At the end of the experiment, we collect the superficial layer of the sediment (0 to 0.5 cm; noted SLanode), which is finally dried at 40 °C. As for salt contamination tests, the initial and final pHs of the solutions are measured: the pH drops from 8.0 to 4.0. This pH variation is probably due to the precipitation of solubilised Al, as aluminium hydroxysulphates form (Gouali, 2013).
3 Results and discussion
3.1 Aluminium mineralogical speciation in natural sediment
The total content of Al in the natural sediment (SL) is equal to 28 ± 1 g·kg−1 (see Table 1). This value remains in the ranges reported by various studies (Gabelle et al., 2012 ; Hamdoun et al., 2015a). As already observed in our previous studies in non-contaminated sediments (Gabelle et al., 2012), Al in the natural sediment SL is mainly scavenged in the residual fraction. Indeed, the two extraction procedures tested (HCl and sequential extractions, Table 1) solubilize less than 9% of the total aluminium, suggesting a poor mobility of this element. The applied sequential extraction (Leleyter et al., 2012) is more aggressive (9% mobilized) than HCl extraction (only 2% of Al mobilized). Such a result is usual for most of the current studied elements, except for lead or zinc (Hamdoun et al., 2015a ; Leleyter et al., 2012).
Al total concentration (g·kg−1) (alkaline fusion: FA) in contaminated (salts or anode) natural marine sediments (SL).
FA (g·kg−1) | HCl (%) | F6 (%) | |
SL | 28 | 2 | 9 |
Ec SL | 1 | 0 | 1 |
SL sulphate | 33 | 2 | 18 |
Ec SL sulphate | 1 | 0 | 2 |
SL chloride | 33 | 2 | 11 |
Ec SL chloride | 0 | 0 | 1 |
SL anode | 29 | 15 | 32 |
Ec SL anode | 1 | 0 | 1 |
Fig. 1 displays aluminium fractionation, according to the sequential procedure applied: the percentage of the total aluminium mobilized in each fraction is reported. The natural sediment SL is characterized by leachable Al almost exclusively present in the reducible fraction (95%) and only 5% in the oxidable fraction.
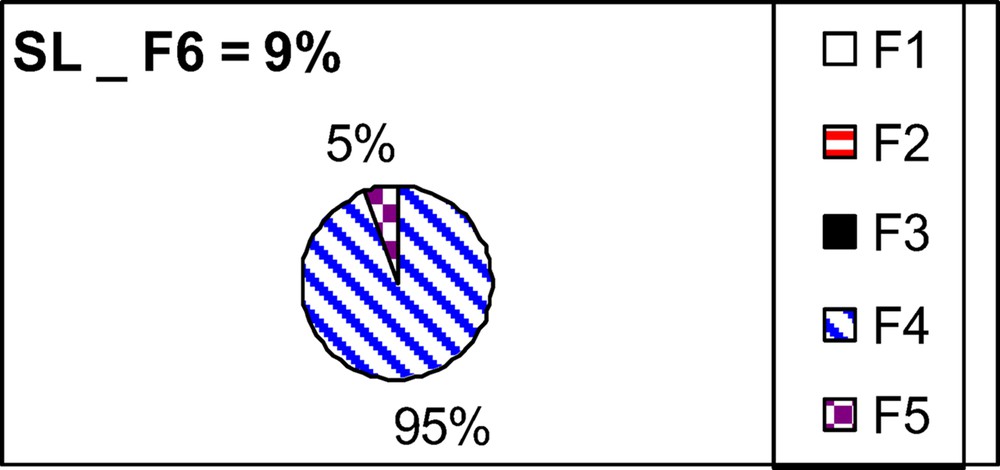
Leachable aluminium fractionation according to the sequential extraction applied.
3.2 Impact of aluminium contamination on aluminium mobility
The total contents of Al in contaminated sediments are respectively 29 and 33 g·kg−1 after the aluminium sacrificial anode contamination and salt contamination tests (see Table 1). Compared to the initial sediment Al content, the increase in total aluminium content is low (5% for the case of the sacrificial anode) to moderate (18% for the contamination by salts). Thus, analysis of total content cannot discriminate the aluminium from anthropogenic source, as anthropological quantities are often negligible compared to the natural amount of this major element.
Focusing on the salts contamination experiments, it seems that the nature of the used salts (sulphate or chloride) affects neither the rate of Al contamination (33 g.kg−1, Table 1), nor the HCl-leachable percentage (2%, Table 1), nor the mineralogical speciation (reducible fraction > 94% with the two salts, Fig. 2). On the other hand, the F6-leachable percentage differs according to the salt nature: Al from sulphate salts is more F6-leachable than Al from chloride salts.

Aluminium partitioning in different mineralogical fractions.
Compared to the natural sediment (SL), the HCl-leachable percentage remains unchanged for the sediments contaminated (2%) with the two salts (despite the moderate increase of the aluminium total content). Whereas an increase in F6-leachable percentage is observed, with values up to 18 or 11% (respectively in case of aluminium sulphate or chloride contamination), compared to 9% for SL. This suggests that the aluminium from salt contamination is scavenged in a mobile fraction that HCl is not able to solubilise.
A previous study, conducted in tidal artificial conditions, with natural sediments artificially contaminated with aluminium salts, highlighted that the Al-contamination had a strong effect on diatom communities growing at the sediments surface, and that 1 mol·L−1 HCl-extraction probably underestimates aluminium bioavailability for phytobenthic communities (Leleyter et al., 2016).
The sediment contaminated by the sacrificial anode stands out by the important HCl-leachable percentage (15%, Table 1) measured, compared to the low HCl leachability (2%) observed for the other sediments studied. Thus, anode contamination induces a rise in the HCl-labile content, which could not exclusively be correlated with the low additional quantity of Al introduced (5%). These results show that cathodic protection by an Al sacrificial anode does not only discharge anthropological aluminium, but also modifies the mineralogical speciation of the initial natural aluminium, and therefore its environmental availability, which is increased. This phenomenon could be explained by the fact that cathodic protection induces changes in redox potential and pH conditions, which are known as environmental factors influencing the speciation and availability of the elements (Leleyter and Probst, 1999).
This increase in the HCl-leachable percentage in the case of sacrificial anode contamination is fairly consistent with our previous study (Gabelle et al., 2012) in an open-air field of the Port of Le Havre (western France), where Al sacrificial anodes are used to protect steel structures against corrosion. Indeed, we observed an important increase in HCl mobility (11%) in the sediment collected near the Al sacrificial anodes, with regard to HCl mobility (4 and 3% respectively) in less or non-contaminated sediments sampled downstream and upstream from the port. The strong HCl-lability associated with the dissolution of the sacrificial anode, confirmed by the present study, is worrying, as sediments characterised by aluminium HCl mobility superior to 4% induced important negative effects for benthic diatoms growing at the surface of the sediments, reported in Leleyter et al. (2016).
Moreover, this difference of HCl-mobility according to the nature of the contamination source (salt or anode dissolution) could be explained by the mineralogical speciation of labile aluminium. Fig. 2 shows that labile Al is almost exclusively bound to the reducible fraction (> 94%) for the sediments contaminated by the aluminium salts. In contrast, for the sediment contaminated by Al anodes, even if the reducible fraction remains the prevailing one (69%), a significant percentage of labile Al is also found in the oxidable (3%) and acid-soluble (28%) fractions. This acid-soluble fraction is mainly composed of carbonates, phosphates, and sulphates (Leleyter and Baraud, 2006); the use of sacrificial anodes in seawater induces the formation of a calcareous deposit, mainly constituted of calcium carbonate (Rousseau et al., 2010). Indeed, cathodic currents induce dissolved oxygen reduction – Eq. (1) –, which generates hydroxyl ions at the very near polarized surface, which increase the interfacial pH and result in enhanced carbonate ion concentration – Eq. (2) – and in the precipitation of an inorganic layer whose principal component is calcium carbonate, even containing some magnesium – Eq. (3). This mixed deposit is generally called “calcareous deposit”.
(1) |
(2) |
(3) |
Then, it is possible that the Al3+ ions, resulting from anode dissolution, co-precipitate with the calcareous deposit. The incorporation of foreign metal ions in this deposit has already been observed in a previous work (Carré et al., 2017). Parts of this deposit can fall apart and get mixed up with the sediment. In the same way, the likely formed precipitates of aluminium hydroxysulphates can be finally incorporated within the sediment. These compounds then enrich the acid-soluble fraction. Similar results have been obtained in the analysis of natural sediments collected near to sacrificial anodes installed in the Port of Le Havre (Gabelle et al., 2012). This presence of aluminium in the acid-soluble fraction could have an important environmental impact, as this fraction is generally considered as really easily available.
4 Conclusion
The Al total content in a natural sediment (28 ± 1 g·kg−1) was poorly solubilized, either by single HCl or by sequential (Leleyter and Probst, 1999) extractions, suggesting a poor mobility of this element. Moreover, leachable aluminium is scavenged mostly exclusively (95%) in this reducible fraction of this natural sediment (5% in the oxidable fraction).
Aluminium contamination tests (with aluminium salts or aluminium from sacrificial anode) induce a low increase in the Al total concentration with regard to the non-contaminated sediment. Thus, anthropogenic aluminium in sediments could not be detected by total mineralization methods, as anthropological quantities are often negligible compared to the natural amount of this major element.
For the sediments contaminated by Al salts, we notice an unchanged value for the HCl Al-leachable percentage (2%), but a strong increase in the F6-leachable percentage (SL: 9%, SLsulphate: 18% and SLchloride: 11%). This suggests that Al coming from salt contamination is scavenged in a mobile fraction of the sediment that HCl extraction is not able to solubilise. A previous study (Leleyter et al., 2016), which highlighted that Al-contaminated sediments had a strong effect on the diatom communities growing at the surface of the sediments, proved that 1 mol·L−1 HCl extraction (widely used in the literature for metal mobility evaluation in marine sediments) underestimated the aluminium bioavailability for phytobenthic communities. Thus, we do not recommend 1 mol·L−1 HCl single extraction to study aluminium mobility in sediments.
Moreover, we note that cathodic protection by Al sacrificial anodes does not only discharge anthropological aluminium in the environment, but also modifies the mineralogical speciation of the initial natural aluminium, and therefore its environmental availability, which is increased (F6-leachable: 32%). Thus, labile aluminium is mainly bound to the reducible fraction (> 94%) in the sediments contaminated with salts, whereas it is also bound to the acid-soluble (28%) and oxidable fractions (3%) for the sediment contaminated by the sacrificial anode.
This rise of Al-lability could be explained by:
- • a modification of the mineralogical speciation of the natural aluminium induced by the changes in redox potential and pH conditions;
- • and/or by the use of sacrificial anodes in seawater, which induces the formation of a calcareous deposit; the Al3+ ions resulting from anodes dissolution co-precipitate with the calcareous deposit and enrich (as also probably aluminium hydroxysulphate precipitates) the acid-soluble fraction of the sediments.
Then, it seems that the origin of the anthropological contamination (aluminium salts or aluminium sacrificial anode) has a considerable importance on the form of aluminium sorption on the sediments, which has a direct impact on aluminium speciation and distribution in the sediments fractions. The presence of aluminium in the acid-soluble fraction of the sediments could have an important environmental impact, as this fraction is generally considered as really easily available. Thus, we do not recommend the use of aluminium sacrificial anodes in natural environments, especially if the installations to be protected are close to the coast, facilitating metal accumulation in the sedimentary phase.