1. Introduction
Models for painters, poets, and writers, scenario of many myths and legends, often mysterious, mountains also provide a wealth of ecosystem services. Mountains are characterised by a rugged topography and higher elevation than the surrounding landscape, therefore exhibiting strong elevation-dependent gradients in terms of temperature and precipitation. Cryospheric components are part of many mountain ranges, with seasonal snow lasting up to several months at intermediate and high elevations, and the presence of permafrost (ground frozen for at least two consecutive years) and glaciers at the highest elevations. The European Alps cover 1.8% of the continent (190,000 km2), and are located in the mid-latitudes (43–47 °N), hosting a diversity of ecosystems, landscapes, cultures and socio-economic activities. The Europeans Alps spans a large range of elevations culminating at the top of Mont Blanc, above 4800 m a.s.l. (Figure 1). Mountains of the European Alps provide water to the largest European rivers, and contribute key natural resources such as hydropower and timber. The European Alps are also the scenes of many natural hazards such as rockfalls, floods, avalanches, landslides to which human societies have long been exposed.
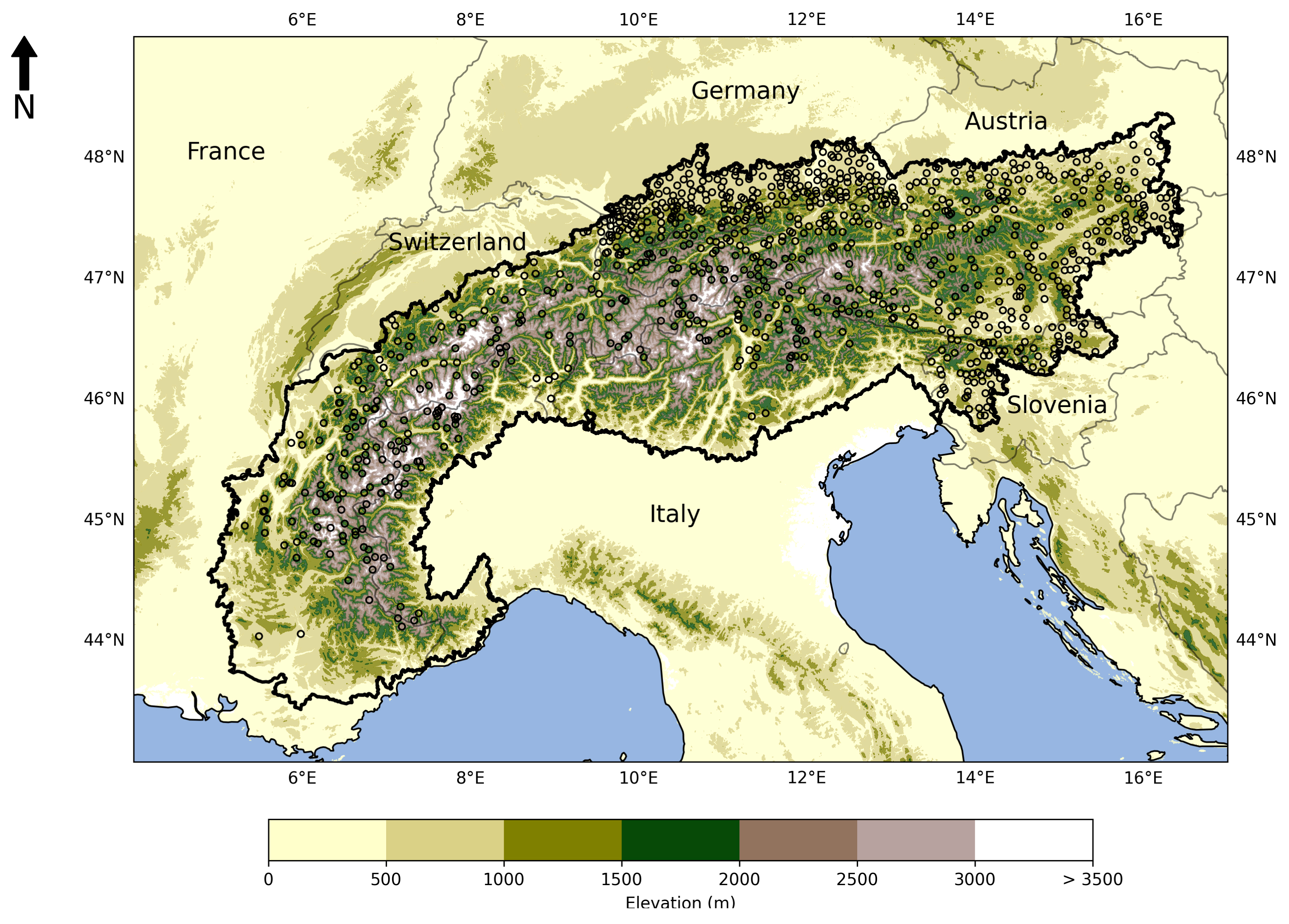
The European Alps as defined by the Alpine convention perimeter in Piva (2020). The circles represent snow depth measurements stations where the time serie of measurement is longer than 10 years (Matiu, Crespi, et al., 2021; Monteiro and Morin, 2023).
Climate change affects the entire planet. Anthropogenic greenhouse gases emission has led to a global near-surface air temperature increase that has reached +1.1 °C in 2011–2020 above the reference period 1850–1900 (IPCC, 2021). As the rest of the planet, mountains areas are affected by climate change with a broad range of impacts for ecosystems, human societies and geomorphological processes. The Copernicus Climate Change Service reports on the European State of the Climate highlights that Europe is the fastest warming continent in the world, with a warming occurring at a rate twice that of the rest of the world (C3S, 2024). Due to climate change, the European Alps landscapes and their surrounding are being affected by changes in precipitation regime and higher temperatures, raising the average rainfall-snowfall elevation limit, melting snow and glaciers, and thawing permafrost. Implications to changes of the cryosphere are far-reaching as well as the consequences for mountain ecosystems and societies.
Several studies have reviewed changes in one specific component of the mountain environment, such as the cryosphere in Beniston et al. (2018). In 2019, the Special Report on the Ocean and Cryosphere in a Changing Climate (SROCC) (Hock et al., 2019) assessed the knowledge on climate change and its impacts in mountains regions for all the different components of the cryosphere. The present paper builds on SROCC and brings together more recent studies to review the observed and projected changes in meteorological conditions in the European Alps and the impacts of these changes on all the main components of the mountain system, with a focus on the French and Swiss Alps. Otherwise explicitly mentioned in the text, European Alps refer to the area contains within the boundaries of the Alpine Convention (Piva, 2020) depicted in Figure 1.
2. Past and projected changes in meteorological variables
Climate change induces profound modifications of the Earth System. The first, and most known manifestation of climate change, is near surface air temperature rise. Changes in temperature triggers numerous feedbacks that in turn modify other meteorological variables. Here we review the changes in temperature and precipitation affecting the European Alps, the two main drivers of the cryosphere changes.
2.1. Air temperature
Quantifying accurately the magnitude of the temperature increase in mountainous areas is a difficult task. Firstly, it varies in space due to the complex topography. Secondly, mountains are remote places, experiencing harsh meteorological conditions and exposed to natural hazards. These factors make it difficult to access and maintain monitoring stations over the long term, leading to geophysical records including air temperature usually scarce and shorter than in surrounding lowlands. Nevertheless, a number of studies assessed the change in temperature in the European Alps (Auer et al., 2007; Hock et al., 2019; Isotta et al., 2019; Beaumet et al., 2021; Pepin, Arnone, et al., 2022; Philipona et al., 2023). These studies used available in situ measurements, statistical methods to reconstructed and homogenized the time series of measurements as well as global and regional reanalysis evaluated against the in situ measurements. These studies estimate that, since 1970, the rate of temperature increase has been 0.3 ± 0.2 °C per decade. The current increase in temperature in the European Alps since 1850–1900 is therefore approximately 2.0 ± 0.3 °C (see Figure 2, methods and references therein). This increase is higher than for the rest of the planet since air temperature over land surfaces increases faster than over oceans (IPCC, 2021) but is in line with the average warming in Europe (2.3 ± 0.4 °C over the past 5 years, C3S (2024)) and a little higher than the average warming over metropolitan France (1.7 °C, Ribes et al. (2022)).
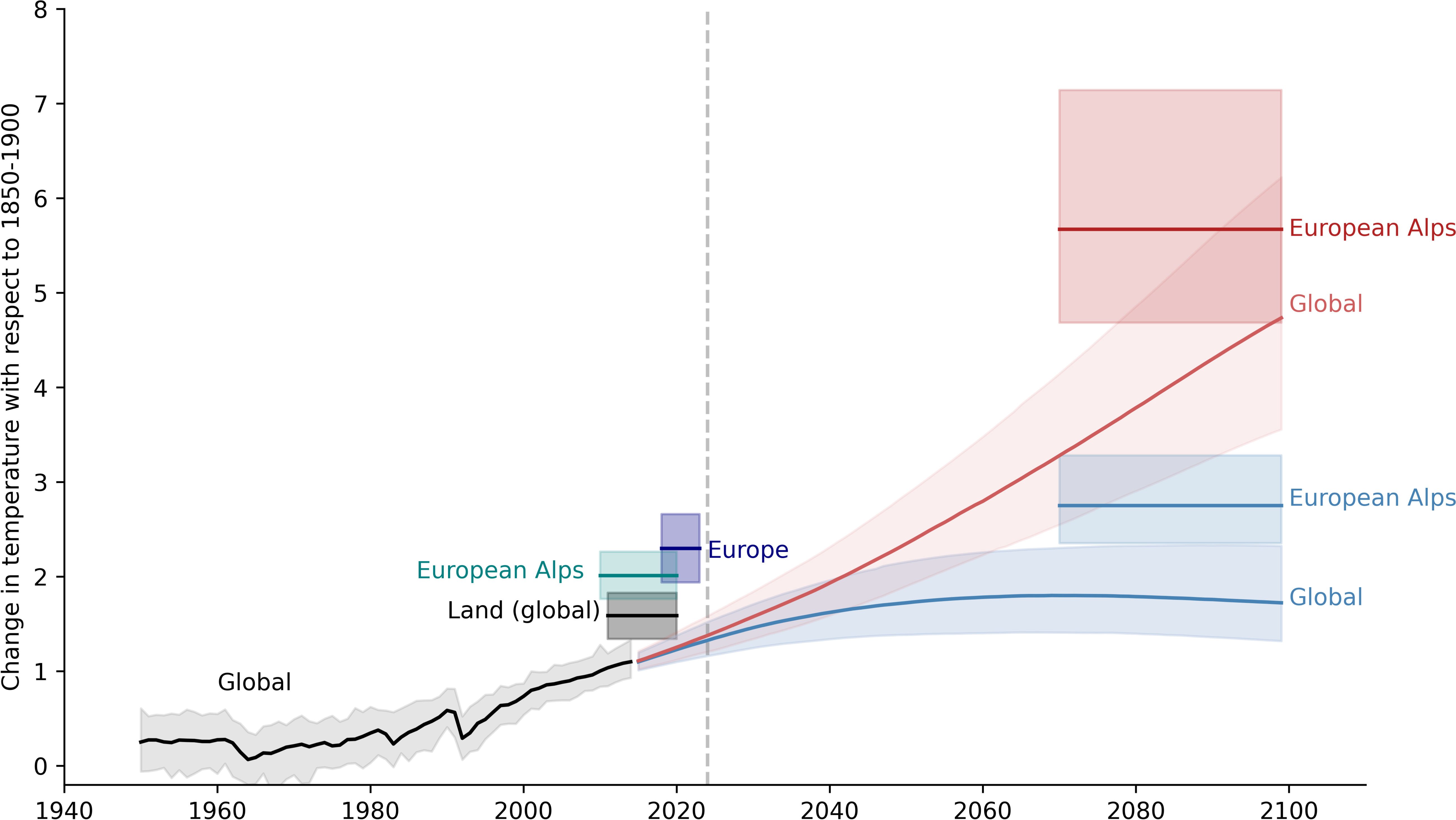
Change in temperature with respect to 1850–1900. The black lines and the global projected temperature change are taken from IPCC (2021). The temperature change for the European Alps (green box) was calculated from 1970 until today based on the values from Monteiro and Morin (2023), assuming that prior changes followed the average change for mainland France with data from Ribes et al. (2022). Projections for the European Alps are calculated from Table S2 in Kotlarski et al. (2023). The blue projections are for a low emmission scenario (RCP2.6 for the European Alps, SSP1-2.6 for global). The red projections are for very high emission scenario (RCP8.5 for the European Alps, SSP5-8.5 for global) (more in IPCC (2021)). Masquer
Change in temperature with respect to 1850–1900. The black lines and the global projected temperature change are taken from IPCC (2021). The temperature change for the European Alps (green box) was calculated from 1970 until today based on the ... Lire la suite
The rate of temperature increase depends on season and elevation. It exhibits a seasonal variations with stronger warming in spring and summer than in autumn and winter, similarly to the situation prevailing in central and southeastern Europe and around the Mediterranean (Simmons, 2022). Warming in the Alps also depends on elevation, but the magnitude and sign of this influence varies with the the season. In the Swiss and the French Alps, winters experience a pronounced warming at lowest elevations, while summers exhibit a pronounced warming at elevations higher than 2000 m a.s.l. (Pepin, Arnone, et al., 2022; Scherrer, 2020; Isotta et al., 2019; Rottler et al., 2019; Beaumet et al., 2021; Monteiro and Morin, 2023). These conclusions were drawn thanks to available in situ measurements, statistical methods and regional reanalysis over the French and the Swiss Alps. Several physical processes can explain this elevation dependant warming: decrease in the aerosols content of the atmosphere, increase and decrease in cloud cover as well as a darkening of the surface in spring due to earlier snow retreat (a.k.a. the snow albedo feedback, see Section 3.1) (Pepin, Bradley, et al., 2015). Locally, the contribution of these processes may vary, which makes it difficult to derive common simple rules.
Climate projections indicate that warming in the European Alps will continue at almost the same rate (0.3 ± 0.2 °C) per decade until about 2050 for as long as net greenhouse gases emission, i.e. releases of greenhouse gases from anthropogenic sources minus removals by anthropogenic sinks, is above zero (Hock et al., 2019) (Figure 2). By the end of the 21st century, the increase in temperature is highly dependant on the emission scenario (Figure 2) (Kotlarski et al., 2023). The projected increase in temperature in 2070–2100 compared to the pre-industrial period ranges between 3 to 6 °C in the European Alps (against 1.8 to 5 °C for the global temperature increase in IPCC (2021)). In most cases, the scenarios indicate a more pronounced increase in temperature during the summer and autumn months compared to the winter and spring seasons (Kotlarski et al., 2023). As highlighted in the later reference, projected temperature are subject to considerable model uncertainty, the largest uncertainties being found for the highest projected warming (see Figure 2).
2.2. Precipitations
Precipitations, i.e. snowfall and rainfall, are affected by climate change. Precipitation measurements in mountain regions are even scarcer than temperature measurements and subject to greater uncertainties (Hock et al., 2019; Kochendorfer et al., 2018). Several conclusions can however be obtained using available in situ measurements, statistical methods for homogenization of measurements, as well as regional reanalysis (Masson and Frei, 2016; Ménégoz et al., 2020; MeteoSwiss, 2025; Bozzoli et al., 2024). On an annual scale, no clear trends are found on the total annual amount of precipitation over the European Alps, but seasonal changes are detected, with an increase in winter precipitation over the northwestern Alps for the period 1903–2010, with changes typically reaching up to 20% per century (Masson and Frei, 2016; Ménégoz et al., 2020; MeteoSwiss, 2025; Bozzoli et al., 2024). In summer, a decrease in total precipitation is found in the Southern Alps, remaining small (<10% per century) at elevation higher than 1500 m a.s.l., and is due to a decrease in the number of rainfall days. An increase in extreme precipitations at the annual timescale and also during the four seasons is observed (Scherrer et al., 2016; Ménégoz et al., 2020). Based on regional reanalysis over the French Alps, Le Roux et al. (2021) have shown that the magnitude of extreme snowfall is increasing at high elevation and decreasing at low elevation in favour of extreme rainfall due to the shift from snowfall to rainfall in a warmer climate.
Projections of precipitation are subject to large uncertainties concerning the magnitude of changes by the end of the 21st century. They show an intensification of the seasonal changes already observed over the last century, with wetter winters and drier summers. These contrasting future seasonal changes are explained by large-scale atmospheric circulation (Rajczak and Schäer, 2017; Blanc et al., 2022). Indeed, the European Alps are in a transition zone between the Mediterranean basin, on a drying trend, and the central and northern Europe, likely on a wetting trend (Rajczak and Schäer, 2017). The location of this transition zone varies depending on the season and its future evolution will likely determine the regional sign of precipitation changes. While the respective trends do not vary with the scenario, the intensity of the changes scales with the temperature rise and is more pronounced after 2050 (Kotlarski et al., 2023). Despite these seasonal variations concerning the amount of precipitation, extreme precipitation events are expected to intensify in all seasons (Rajczak and Schäer, 2017; Gobiet and Kotlarski, 2020).
Other meteorological variables, such as cloud cover, aerosols content and deposition, longwave and shortwave radiations and winds may also be affected by climate change, although their changes both from a model and observation standpoint is more difficult to detect and less documented (Hock et al., 2019; Philipona et al., 2023).
3. Past and future changes in the mountain cryosphere
Consistent and recurrent below-freezing temperatures in the higher elevations enable the presence of the solid phase of water, ice, and therefore the existence of many cryospheric processes. The mountain cryosphere includes several components, in particular snow cover, glacier and permafrost, that are extremely sensitive to the rise in temperatures (Beniston et al., 2018; Hock et al., 2019). This section reviews past and future changes of the mountain cryosphere in the European Alps.
3.1. Snow cover
The snow cover is a major component of the mountain environment especially in high and mid elevation mountain ranges such as the European Alps. The snow cover results from the accumulation of snowfalls on the ground during winter, and gradually melts in spring or transforms to firn, and ultimately to glacier ice, at high elevation. In the Alps, snow covers the ground for at least 6.5 months per year above 2000 m elevation (Hüsler et al., 2014; Marty, Schlögl, et al., 2017).
The presence of snow on the ground is very important for many aspects due to its unique properties. First, snow has an albedo, i.e. fraction of reflected solar radiation, close to 1 and thus limits the absorption of solar radiation (Warren, 1982). Second, snow is a reservoir for water in mountain regions and the surrounding lowland, buffering river discharge from winter to spring and summer, providing high flow at the time of melt (Fayad et al., 2017). Finally, as the snow cover has a high air content (e.g. 50–87% of its volume), snow acts as an insulator, protecting the ground from air temperature variations, buffering cold temperatures during winter months to reach the ground, and preventing warm temperatures during springtime (Bender et al., 2020) to heat the ground. Changes in the snow cover due to climate change thus have important consequences for several components of the mountain system that are detailed in Section 4.
As a consequence of sustained warming, the snow cover is shrinking worldwide (Pulliainen et al., 2020). Overall since 1950, the duration of snow cover in the European Alps has shortened by roughly one month below 2000 m elevation (Matiu, Crespi, et al., 2021). Several snow observation sites have been maintained in the Alps for a few decades, providing a unique insight on the signal of climate change in mountain regions (Lejeune et al., 2019; Guyomarc’h et al., 2019; Marty and Meister, 2012). For instance, at the Col de Porte site, located at medium elevation (1325 m a.s.l.) in the French Alps, the mean winter air temperature (December 1st to April 30th) has increased by 1.05 °C between the two 30-year periods of 1960–1990 and 1990–2020, while the total amount of winter precipitation has not changed significantly (Figure 3 upper and lower panel). As a result, the mean snow depth decreased by 40% between the two periods due to (i) a temperature-induced change to more rainfall on the cost of snowfall at this mid-elevation site where the winter temperature is close to 0 °C (0.55 °C from December to April) (Serquet et al., 2011), and (ii) stronger melting in a warmer climate, i.e. a melting that starts earlier in the season. The increase of the rain vs. snow precipitation elevation limit is the first cause of an increasing snow scarcity at low and mid-elevation (Hock et al., 2019). At higher elevation, as exemplified by the Weissfluhjoch site, 2536 m a.s.l. in the Swiss Alps (Figure 3 in light blue), temperatures have increased roughly the same for the same periods than at Col de Porte (Marty and Meister, 2012), i.e. 1.16 °C between the two 30-year periods of 1960–1990 and 1990–2020. However, the average snow depth from December to April did not change significantly and no major change in total precipitation is detected (Figure 3). The reasons for this are that (i) the average winter temperature at this elevation is still well below freezing (−7.35 °C at Weissfluhjoch) and (ii) the increase in absolute temperature due to climate change is not sufficient to convert snowfall to rainfall for the period December to April. Nevertheless, the snow cover duration even at 2536 m a.s.l. has shortened in late Spring. Our analysis in Figure 3 shows how the snow melt-out date occurs 15 days earlier on average between the two 30-year mean. The increase in spring temperature leads to faster melt explaining this earlier melt-out date. In fall, the snow onset date has however not significantly changed for higher elevations with continuous snow cover during winter (Klein et al., 2016; Essery et al., 2020; Matiu, Crespi, et al., 2021; Vorkauf et al., 2021). Thus, the shorter snow cover duration is the second cause of snow disappearance in the Alps due to climate change. As illustrated in Figure 3, the snow depth exhibits a very strong interannual variability. The impact of climate change can thus not be evaluated on a limited number of years but require average over long time series (typically 30 years). This also implies that temperature rise causes a decrease in the frequency of snowy winters in favour of the frequency of winters with very shallow snowpacks. To summarise, the snow cover is retreating on average as a response to air temperature increase, occurring faster at low and mid-elevation due to the change in the precipitation phase (i.e. rain instead of snow) but also at higher elevation due to earlier melt.
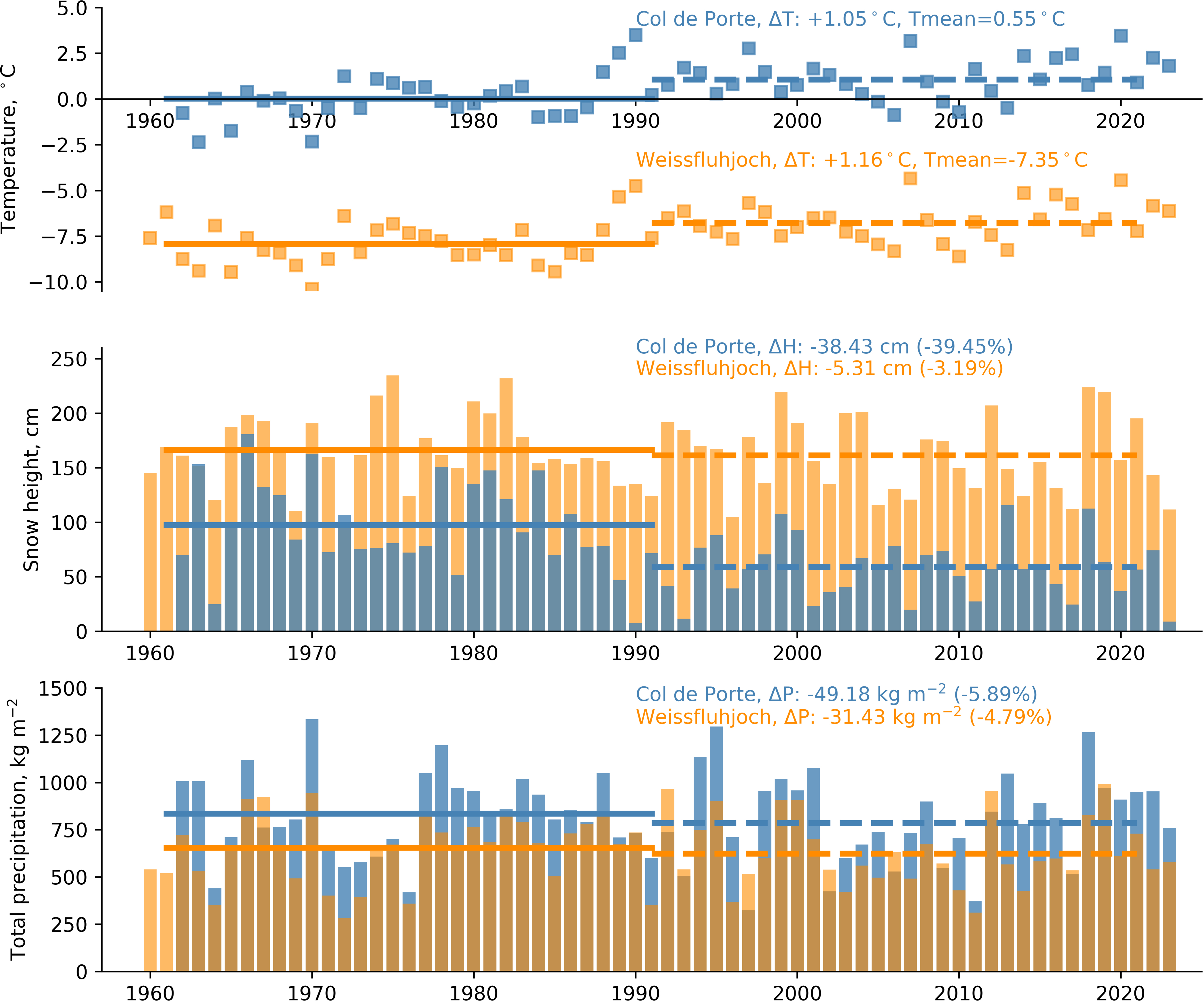
Evolution of air temperature (upper panel), mean snow depth (middle panel) and total precipitation (rain+snow, lower panel) since 1960 at two observations sites. All data are averaged over the same period (December 1st of year n − 1 to April 30th of year n). The Col de Porte site is located in the French Alps at 1325 m elevation (deep blue marker, Lejeune et al. (2019)). Precipitation data are corrected from undercatch and other artifacts (Lejeune et al., 2019). The Weissfluhjoch observation site is located in the Eastern Swiss Alps at 2536 m elevation (light blue markers). Precipitation data for Weissfluhjoch measurements are taken from snow board only since the rain contribution is negligible over the considered months. For data and site description, see Marty and Meister (2012). The horizontal lines corresponds to the 30-years mean (solid line 1961–1990 and dashed line 1991–2020). The absolute and relative differences between the two 30-year means are indicated on each panel. Masquer
Evolution of air temperature (upper panel), mean snow depth (middle panel) and total precipitation (rain+snow, lower panel) since 1960 at two observations sites. All data are averaged over the same period (December 1st of year n − 1 to ... Lire la suite
The projected changes in snow cover show a decline for all scenarios considered, with a response of the snow cover directly modulated by the temperature rise (Hock et al., 2019; Marty, Schlögl, et al., 2017) (Figure 4). Hock et al. (2019) and Kotlarski et al. (2023) estimate that snow depth at low and mid-elevation (i.e. below 2000 m a.s.l.) will decrease by 25% (±15%) in 2050 for every emission scenario, associated with a decrease in snow cover duration. Under high emission scenario (RCP 8.5) the decrease in snow depth could reach 80–90% in 2100 for these elevations. This decrease is explained, as for the observed decrease, by a change in precipitation phase, especially pronounced early and late in the snow season (Piazza et al., 2014), and a more intense melt in a warmer climate. The projected increase in winter precipitation will not compensate for the loss of the snow season duration (Kotlarski et al., 2023). At higher elevations, i.e. above 2000 m a.s.l., the decrease will be less pronounced. Though, the snow duration will decrease due to an earlier disappearance in springtime. At higher elevations (typically above 3000 m a.s.l.), where this not many observation available (Figure 1), uncertainties in projections are still quite high with models having difficulties to apprehend the sharp and rugged topography due to too coarse resolution (Terzago et al., 2017; Monteiro, Caillaud, et al., 2022; Matiu, Napoli, et al., 2024). All in all, the decrease in snow cover will continue as long as global temperature will continue to rise, and the seasonal snow cover will only be able to stabilise once global temperatures will do so.
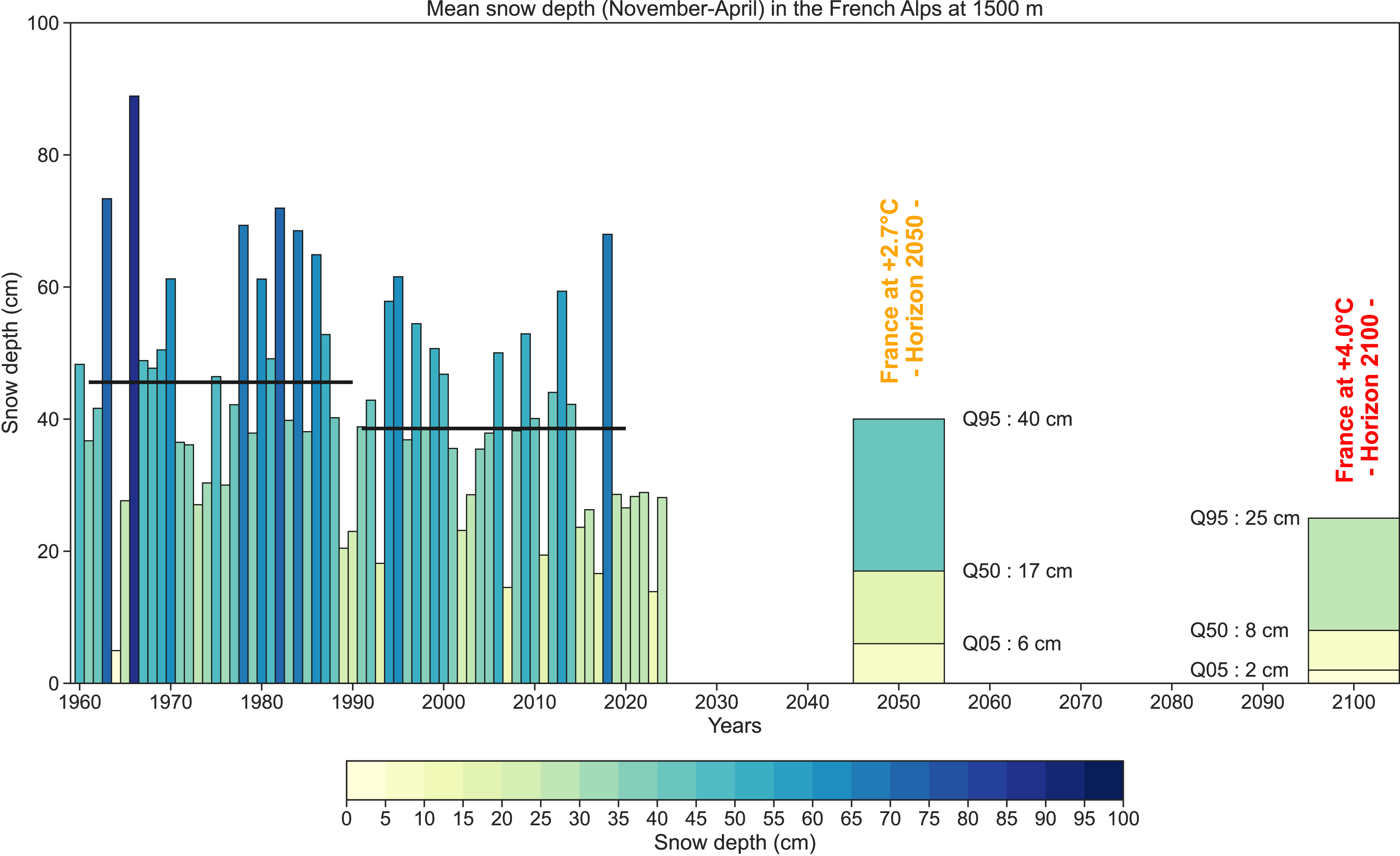
Past and projected evolution of mean snow depth during the period November to April in the Mont-Blanc massif at 1500 m elevation. The colours indicates the snow depth. The horizontal black lines corresponds to the average snow depth from the periods 1961–1990 and 1991–2020 (respectively 45.6 and 38.6 cm). Projected snow depth for 2050 and 2100 are reported with the quantile. Q05, Q50 and Q95 respectively represent quantile 5%, 50% and 95% of the ensemble. Data from Samacoits et al. (2022). The emission scenario corresponds to the French national pathway for adaptation to climate change, +3 °C in 2100 for global temperature with respect to 1850–1900 (SSP2-4.5). Masquer
Past and projected evolution of mean snow depth during the period November to April in the Mont-Blanc massif at 1500 m elevation. The colours indicates the snow depth. The horizontal black lines corresponds to the average snow depth from the periods ... Lire la suite
Deposition of light absorbing impurities such as black carbon, originating from fuel combustion, mineral dust (mostly originating from the Saharan desert in the European Alps) or also algae accelerate snow melt by decreasing the snow albedo (Dumont, Tuzet, et al., 2020; Réveillet et al., 2022; Dumont, Gascoin, et al., 2023; Roussel et al., 2024). The deposition of such particles modulates the response of mountain snow cover under climate change. While the amount of black carbon deposited on snow has decreased since 1990, there is no trend on the amount of mineral dust deposition in the last 40 years over the French Alps (Réveillet et al., 2022). In the future, black carbon deposition are projected to decrease while there is no consensus yet on the sign of the trend for mineral dust (IPCC, 2021; Mirzabaev et al., 2019). The future evolution of these depositions will thus participate in the future evolution of the snow cover in the European Alps.
The reduction in snow cover has many consequences. In the United States, snow was estimated to contribute significantly to the western US economy (Sturm et al., 2017). A lot of parallels could be drawn in between the Alps and the western US in terms of socio-economic activities (agriculture, hydropower and winter tourism), the European Alps being even more densely populated. The reduction of snow cover will thus profoundly change society and the economy. The main impacts of a retreating snow cover are discussed in Section 4.
3.2. Glaciers
Glaciers are often seen as sentinels of climate change. They are one of the important symbols of mountain regions, imprinting their presence on the shape of mountains. Glaciers cover 2092 km2 in central Europe, most of them found in the European Alps (Hock et al., 2019). If their entire volume of ice was to melt, glaciers of the European Alps would contribute to 0.3 ± 0.1 mm of global sea level rise, a small contribution compared to, for example, the melting of the Greenland ice sheet corresponding to more than 7 m in equivalent sea level rise (ibid.). In the European Alps, glaciers have been retreating since the little ice Age (1850) in response to changes in temperature and precipitation. For the past 50 years, alpine glaciers have lost on average 40% of their surface area (Sommer et al., 2020), as exemplified by La Mer de Glace glacier on Figure 5. On average, glaciers of central Europe have lost 1.02 ± 0.21 m water equivalent per year for the period 2000–2019 (Hugonnet et al., 2021).
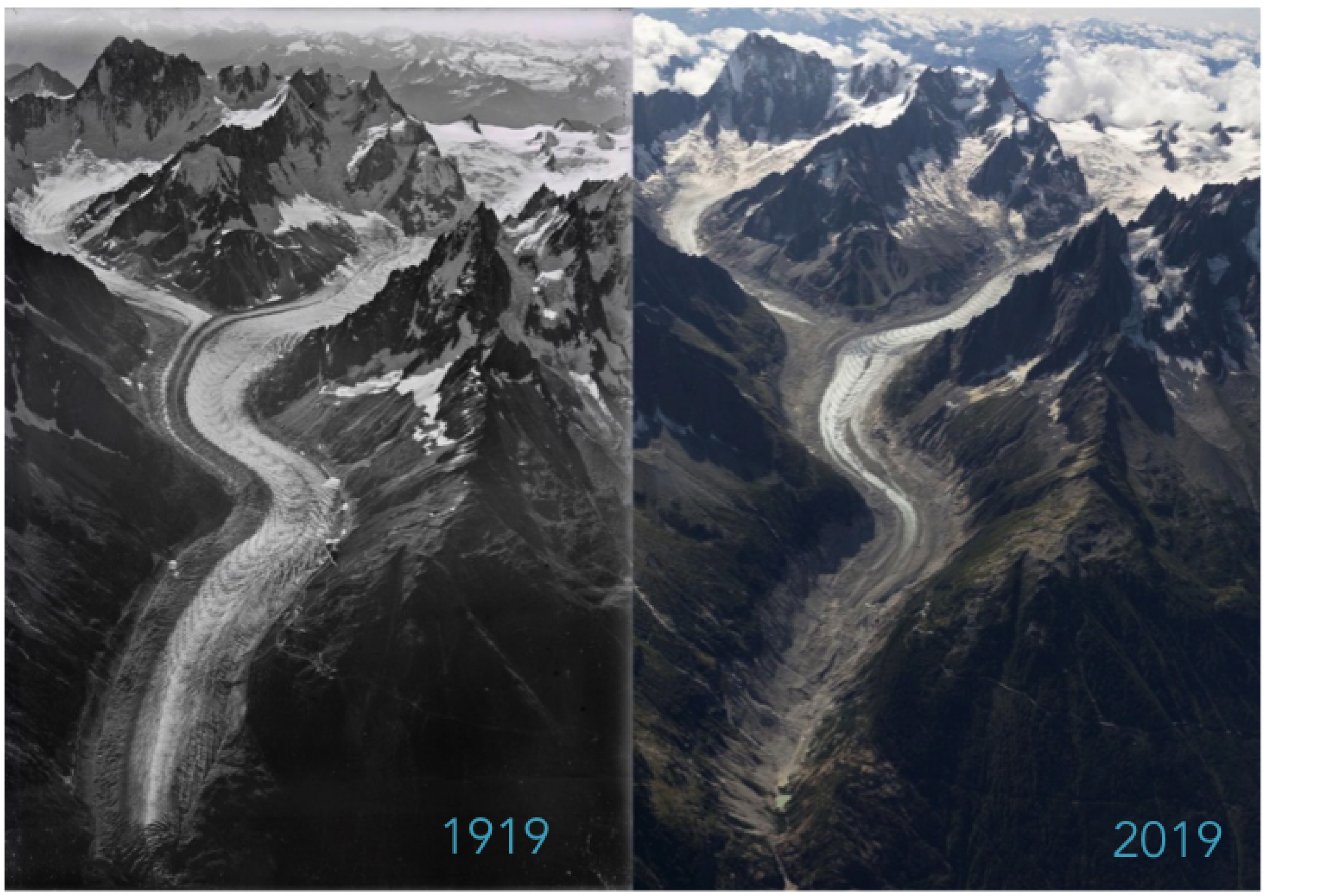
The Mer de Glace glacier (Massif du Mont Blanc in 1919 (left panel) and 2019 (right panel). Source: http://ige-vis.univ-grenoble-alpes.fr/glacioclim/glacioclim.html.
Projections are showing that, regardless of the emission scenario, glaciers of the European Alps below 3500 m a.s.l. will very likely disappear before the end of the century (Compagno et al., 2021). Small glaciers with accumulation areas below 3400 m a.s.l. such as Saint Sorlin glacier, in the French Alps, should even disappear by 2050, regardless of the climatic scenario. Such small glaciers account for 75% of the glaciers in the French Alps (Vincent, Six, et al., 2023). For larger glaciers such as the Mer de Glace (Figure 5), their state at the end of the century depends on the emission scenario. The Mer de glace would loose 80% of its surface area in 2100 under the intermediate emission scenario (RCP 4.5), while it would disappear before 2100 under the high emission scenario (RCP 8.5) (Vincent, Peyaud, et al., 2019). In contrast to the seasonal snow cover that mostly evolves in response to the meteorological conditions of the current year, the time evolution of glaciers results from a cumulative process by which glaciers gain or lose mass on an annual scale—most often lose, in the past decades, because of their negative annual mass balance under current climate conditions. This explains why the future of most low- and medium-elevation glaciers is sealed, regardless of the emission scenario, towards total disappearance by 2050. In contrast, the number of glaciers that will disappear and the evolution of larger glaciers with high accumulation areas largely depends on the emission scenario. This is why every increase in temperature matters (Rounce et al., 2023).
Beyond global sea level rise, the shrinkage of glaciers has important local and regional impacts on human societies, including hydrology, geohazards, and mountain recreational activities and tourism (Mourey, Perrin-Malterre, et al., 2020; Salim et al., 2023; Weissbrodt et al., 2024) (see Section 4). In glacierized catchments, glaciers act as seasonal and long-term reservoirs, releasing water in rivers from the melt of snow accumulated during winters, and the melt of ice accumulated in past decades and centuries. Therefore, the retreat and disappearance of alpine glaciers will lead to important changes in the water cycle (see Section 4.1) especially important to downstream communities and ecosystems close to the glaciers. Moreover, when glaciers retreat in a valley there are a number of geomorphological cascading and compound effects: (i) the buttressing effect glaciers can have on the surrounding slopes is removed, which increases the instability of steep slopes (McColl, 2012), (ii) unconsolidated debris generated by glacial erosion are exposed to the surface, leading potentially to debris flow during high snow melt or intense rain events (Ballantyne and Benn, 1994), (iii) the basal topography of glaciers may reveal local depressions leading to the formation of lakes below unstable slopes (Vincent, Six, et al., 2023; Haeberli, Schaub, et al., 2017) and (iv) the increasing chance of the formation of glacial lakes and therefore of glacial lake outburst and debris flow (e.g. GLOF, see e.g. Emmer (2017)) (see Section 4.4).
3.3. Permafrost
Permafrost, or “permanently” frozen ground, is a less visible component of the mountain cryosphere than snow and glaciers nonetheless important. Permafrost is defined as ground continuously remaining below 0 °C for at least two consecutive years. It can be found in soft materials (e.g. soil, talus slope, rock glaciers) or in bedrock. Permafrost is a subsurface phenomenon, less accessible to observational means than snow and glaciers and thus generally less monitored and understood (Hock et al., 2019). Permafrost covers roughly 6220 km2 in the whole European Alps, almost 3 times the glacier area (Boeckli et al., 2012; Marcer, Bodin, et al., 2017).
Permafrost temperature is driven by (i) the surface energy balance and therefore by air temperature, solar and longwave net radiation, snow cover, vegetation, and (ii) by the ground thermal properties (i.e. soil moisture, soil stratigraphy). Because of the soil thermal inertia, permafrost may build up over centuries and is often a remnant of previous climatic conditions at depths. As a consequence, mountain permafrost distribution is heterogeneous and responds to local conditions in energy fluxes (Magnin, Ravanel, et al., 2024). It is found, for the most part, at the upper elevations of alpine mountains (Boeckli et al., 2012) and anecdotally in places at lower elevation where local effects allow for sustained freezing conditions (Lambiel and Pieracci, 2008). Snow plays a critical role in permafrost dynamic (Luetschg and Haeberli, 2005; Gisnås et al., 2016; Noetzli et al., 2024). We know that snow acts as an insulator for permafrost and prevail warmer conditions than areas bare of snow. The timing of arrival of snow in fall is also crucial, as for instance a late snowpack arrival will cool the ground. Snow, in steep permafrost areas was identified to be a significant contributor to liquid water infiltration during snowmelt, a mechanism known to trigger rockfalls and advect energy in the ground (Ben-Asher et al., 2023). Yet these feedback mechanisms are still poorly characterised for steep slope permafrost, most common across the Alps (e.g. rock cliffs).
Different than boreal and Arctic permafrost which holds large amount of carbon (Schuur et al., 2015), mountain permafrost in the Alps is mainly affecting slope stability of steep rock slopes emblematic of the highest alpine peaks. Thawing permafrost has important consequences for infrastructures located in the vicinity of unstable slopes or downstream of alpine valleys affected by permafrost, and therefore for the safety of human society in mountains regions (see Section 4.4). Permafrost in the European Alps has warmed up and thawed for the last decades (Hock et al., 2019; Haberkorn et al., 2021; Magnin, Ravanel, et al., 2024). The rate of warming exhibits high local variability. Ice-poor bedrock warmed by up to 3 times faster than the average values (Etzelmuller et al., 2020; Magnin, Ravanel, et al., 2024). Latest estimates based on borehole observations located across the Alps shows 0.36 °C per decade warming rate at 10 m depth for the last decade (Noetzli et al., 2024). The active layer thickness, corresponding to the depth of seasonal thawing, has also been increasing (Hock et al., 2019; Magnin, Ravanel, et al., 2024). Subsurface liquid water content is increasing too, indicating ice loss (Hock et al., 2019; Bodin, Thibert, et al., 2009).
Rock glaciers, a permafrost landform made of a mixture of rock and ice, experience more stable temperature benefiting from an insulating effect of the upper layer of debris but exhibit a variety of responses to rising temperature. Marcer, Cicoira, et al. (2021) showed that some rock glaciers can enter a phase of destabilisation during which their speed increases. 5% of the rock glaciers in the French Alps were found to be in this phase, for which the mean annual temperature explains 55% of the displacement rate variance. Similar behaviour were found across the Alps for a subset of rock glaciers (Kellerer-Pirklbauer et al., 2024). Most rock glaciers, however show no acceleration (potentially inactive) or even decelerating flow speeds (Kellerer-Pirklbauer et al., 2024; Manchado et al., 2024). The longest time series of observed permafrost temperature from the rock glacier of Murtèl-Corvastch in Switzerland shows that since 1987, temperatures between 15 and 25 m depth as increased by 1 °C in the last 30 years (Haeberli, Noetzli, et al., 2023).
Projections indicates that mountain permafrost will likely undergo increasing thaw and degradation during the 21st century, with consequences scaling with the increase in temperatures (Hock et al., 2019). Peaks below 3850 m a.s.l. may lose permafrost entirely under high emission scenario (RCP 8.5) (Magnin, Josnin, et al., 2017). The local response of permafrost as well as a detailed process understanding is however required to refine these projections.
4. Impacts
4.1. Mountain hydrology
Changes in snow cover due to climate change induce changes in mountain hydrology, as conceptually illustrated in Figure 6 (Blöschl et al., 2017). In a warmer climate, the liquid fraction of precipitation increases, causing an increase in winter runoff with an associated larger risk of floods (Bocchiola, 2014; Hock et al., 2019; Marty, Rohrer, et al., 2023). The lower accumulated snow mass at the end of winter leads to a lower and earlier peak of meltwater runoff in spring (Bocchiola, 2014; Hock et al., 2019). Another consequence is the increased severity of low discharge in summer leading to an increase in the risk of drought. The summer drought of 2022 in the European Alps due to the compound effect of the snow droughts and heatwaves provided a glimpse into what could happen more regularly in the coming decades (Gascoin, 2024; Soubeyroux, 2023; Montanari et al., 2023). More intense snowfalls are also expected at high elevations with climate change, increasing the risk of rain-on-snow events in the European Alps at high elevation (Hock et al., 2019). Changes in meltwater from snow often dominate the runoff response at high elevations, while at lower elevations, changes in precipitation and evapotranspiration due to climate change become increasingly important (Bocchiola, 2014; Hock et al., 2019). The shift from snow- to rain-dominated hydrological regime in alpine catchments will increasingly disrupt our ability to predict the availability of water resources for various usage including hydropower generation, irrigation and fluvial navigation, consequently causing profound impacts on the operational management of reservoirs (Hock et al., 2019). It also triggers changes for natural hazards and ecosystems (see following sections). Projections show that these hydrological changes will continue and intensify as long as the air temperature will continue to increase (ibid.).
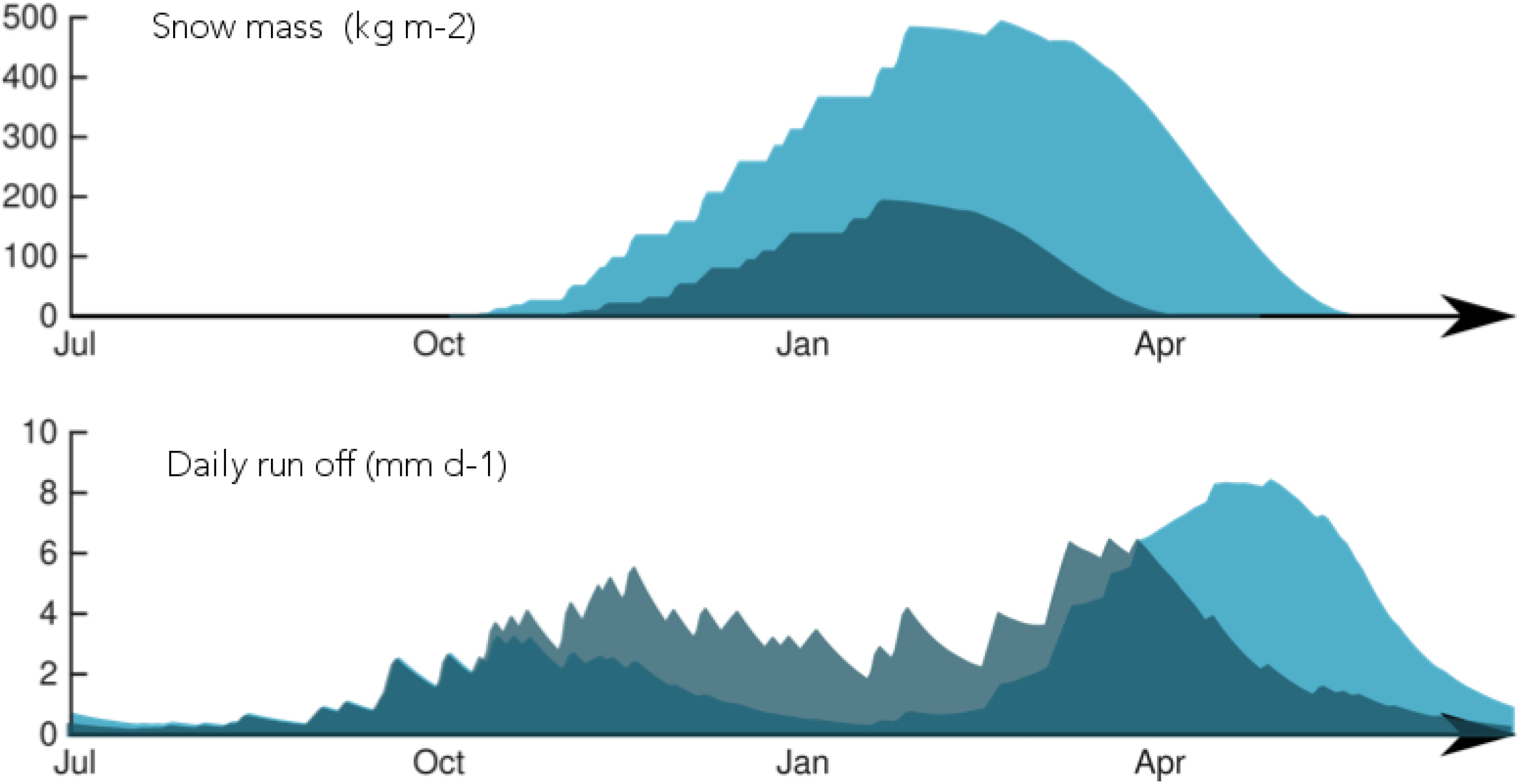
Simulated snow mass (upper panel, kg⋅m−2) and daily run-off (lower panel, mm⋅d−1) for an idealised snow dominated mountain basin (light blue). In the dark blue simulation, the mean annual temperature was increased by +5 °C while the precipitation remained unchanged. Details about the hydrological model and its forcing are given in Gascoin (2021).
In glacierized catchments, glacier retreat also contributes to changes in water availability (ibid.). When a glacier is in equilibrium with climate, its contribution to the water cycle is not very different from seasonal snow, except that it is shifted later in the melt season (Radić and Hock, 2014). However, when the glacier is in a warming climate, the amount of melt water provided in summer increases, reaching a maximum, which is named “peak water” state of the glacier. Beyond that point, the glacier surface becomes too low to provide substantial melt water from ice melt. The glacial contribution to runoff decreases to eventually stop once the glacier has fully disappeared, leading to water shortage in summer. The FAQ 2.1 in Hock et al. (2019) provides a schematic of this evolution. In the European Alps, the peak water date passed for more than half of the glaciers and will be reached before 2100 for all glaciers (Huss and Hock, 2018). Summer runoff contribution from glaciers will therefore be strongly reduced, intensifying issues of water shortages in glacierized catchments such as the Rhone, Rhine, Po and Danube rivers (Huss, 2011). Indeed during periods of droughts, glaciers can represent a non negligible source of water (ibid.).
4.2. Winter tourism
Changes in snow cover affect human societies in mountain areas and beyond. One example is the impact of snow cover changes on winter tourism recently studied at the scale of the European continent (François et al., 2023). Winter tourism in the European mountain ranges is an important economic and societal component (total turnover on the order of 30 billion euros). If the global temperature change reaches +2 °C compared to the pre-industrial era (see Figure 2), 53% of the European ski resorts would be affected by a high level risk of snow scarcity without snowmaking capabilities. A high level risk of snow scarce winters is defined as snow scarce conditions encountered at least once every two years on average. The level of risk varies from 33% of the ski resorts in the French Alps being at high level risk to more than 80% of the ski resorts in the mid-elevation ranges of France and Switzerland (including Jura, Vosges, Massif Central) reaching high level risks. Under a global temperature change of +4 °C, 98% of the European ski resorts would reach high level risk. Snowmaking, when applied to 50% of the surface of ski slopes, could reduce by 7% to 9% the amount of ski resorts at high level risk for +2 °C scenario in the European Alps, while for mid-elevation mountain range the amount of ski resorts at high level risk reduces from 80% to by 56%. However, snowmaking is also impacted by temperature rise as it requires sufficiently low temperature. Climate change impacts ski tourism, but ski tourism contributes to greenhouse gas emissions. Transport and accommodation are the main contributors to the carbon footprint of winter tourism and ski tourism is a good example where adaptation and mitigation needs to be jointly analysed for planning the future of this industry and its relationship with the mountain environment (ibid.).
4.3. Ecosystems
Climate change is significantly impacting European mountain ecosystems, leading to observable changes such as “greening”, species redistribution, community structure alterations, and shifts in ecosystem services (e.g. Brambilla et al., 2024). The European Alps have shown clear signs of “greening”, where vegetation growth increases due to rising temperatures and longer growing seasons. Choler et al. (2021) discussed how these changes are altering the spatial distribution of vegetation, affecting ecosystem services such as water regulation. Additionally, Vitasse et al. (2021) note that phenological shifts in plant species are closely tied to the timing of snowmelt and the subsequent rapid greening of the landscape (also in Kosolapova and Altshuler, 2024). The redistribution of species, driven by climate change, is another significant impact on mountain ecosystems. Species compositions are shifting upwards in elevation, which may lead to changes in community dynamics and the potential loss of species unable to migrate (Hufnagel and Garamvölgyi, 2013). This shifts in species distributions, particularly in the Alps, can further exacerbate the loss of specialized alpine species not able to sustain competition from lower-land species (Rogora et al., 2018). Both greening and species redistribution in response to climate change induce strong structural changes within communities, with shifts in species composition leading to new community dynamics (Bektaş et al., 2021). These community shifts are not only altering biodiversity but also the ecosystem functions these communities provide and the associated ecosystem services (Reed et al., 2013). In this context, an outstanding question is whether the current protected area network will mitigate and safeguard the last pieces of mountain ecosystems. Using semi-mechanistic species distribution models together with scenarios of climate and land use changes, a recent study showed that significant modification of the network will be needed, as it does not adequately represent biodiversity patterns (Chauvier-Mendes et al., 2024). To be efficient, a substantial shift in conservation priorities over time will be needed, moving from lower to higher elevations and across latitudes, as plants migrate upslope and their distributions contract.
4.4. Natural hazards
The mountain cryosphere is directly involved in several natural hazards. As described above, the increase in temperature causes profound changes in the mountain cryosphere, modifying consequently the magnitude, frequency, and types of these cryosphere related natural hazards (e.g. Jacquemart et al., 2024).
The disruption of the water cycle due to an increase in snow cover scarcity and glacier retreat (see Section 4.1) induces changes in rain-on-snow related floods that may become more frequent in winter at high elevation (and scarcer at low elevation) (Stoffel and Corona, 2018). Spring floods are happening earlier due to earlier snow melts (Blöschl et al., 2017). Climate change also induces an increased risk of droughts and water shortages in summer due to the increased lack of water stored as snow in upper elevation (Montanari et al., 2023).
Snow cover is also responsible for snow avalanches, responsible of approximately 100 death per year on average (mainly mountain practitioners in uncontrolled terrain) over the Alps (Techel et al., 2016) as well as disturbances to transportation and threats to mountain infrastructures. Snow avalanches number, types and the related risks are also impacted by climate change (Eckert, Corona, et al., 2024). In the French Alps, the number of large natural avalanches as well as their run-out distance decreased where snow depth decreased (Eckert, Keylock, et al., 2013). Wet snow avalanches also tend to happen earlier (Hock et al., 2019). In general, avalanche natural activity decreased at low elevation (Giacona et al., 2021). At higher elevations more mixed changes are observed with an increase in wet snow activity and these changes will be exacerbated in the future (Hock et al., 2019; Castebrunet et al., 2014).
The retreat of glaciers leads to several glacier related hazards. For cold glaciers, where the ice is below 0 °C, the observed increase in ice temperature can be higher than the increase in air temperature (Vincent, Gilbert, et al., 2020) and could lead to ice gliding and ice avalanches that can endanger population (Faillettaz et al., 2015; Francese et al., 2024). For temperate glaciers (ice at 0 °C), an increase in glacier lake formation due to accelerated melt is observed and can be related to climate change (Hock et al., 2019). However, it is not clear if the frequency of glacier lake outburst floods (GLOF) has evolved and how it will evolve in the future (ibid.). For polythermal glaciers, accelerated melt may favour the formation of aquifers inside the glacier that can lead to the collapse of part of the glacier (Gilbert et al., 2012; Bondesan and Francese, 2023).
Permafrost thawing and degradation induce an increase in the observed frequency and magnitude of rockfalls and rock avalanches (Ravanel et al., 2017; Walter et al., 2020). In combination with heavy precipitation and increase soft soil exposure, it can increase the risk for debris flow (Ballantyne and Benn, 1994). Other damages such as sudden drainage of lakes and destabilisation of infrastructure are also observed (Haeberli, Buetler, et al., 2016; Duvillard et al., 2021; Magnin, Ravanel, et al., 2024; Bodin, Schoeneich, et al., 2015).
For all these risks, cascading and combined hazards can occur. While there is a limited number of observed direct chain of events to project future trends, it seems reasonable to assume that such chain of events combining some of the natural hazards described in this section could increase in frequency and magnitude. Adaptation strategies are thus required for the safety of mountain population as well as for mountain practices (Mourey, Marcuzzi, et al., 2019, Mourey, Perrin-Malterre, et al., 2020; Salim et al., 2023).
5. Concluding remarks
Climate change already strongly influences the meteorological conditions in the European Alps, with an air temperature increase of 2 °C compared to the pre-industrial period (end of 19st century). While the annual amount of precipitation is remaining stable, there is a slight seasonal shift towards wetter winters and drier summers in some regions of the European Alps. All components of the mountain cryosphere, glaciers, snow cover and permafrost, are declining and retreating as a consequence of this temperature increase. Mountain landscapes will be permanently altered, for instance due to the loss of glaciers. These changes trigger profound disruptions for mountain ecosystems and human societies. They will continue to intensify as long as the temperature continues to rise.
This paper reviews recent studies on the trends and impact of climate change in the European Alps, with a focus on the French and the Swiss Alps, based on existing knowledge and highlighting most recent advances in this field. It however does not claim to be exhaustive, calling for a wider report genuinely spanning the entire European Alps domain in a changing climate, involving a larger and more diverse team of authors and including further advances from the research and practitioners communities, and updated climate projections from latest international coordinated climate modelling experiments.
Dedication
The manuscript was written by M. Dumont through contributions of all authors. All authors have given approval to the final version of the manuscript.
Declaration of interests
The authors do not work for, advise, own shares in, or receive funds from any organization that could benefit from this article, and have declared no affiliations other than their research organizations.
Funding
MD has received funding from the European Research Council (ERC) under the European Union’s Horizon 2020 research and innovation program (IVORI; grant no. 949516).
Acknowledgements
The authors are grateful to Aurélien Ribes (CNRM) and Martin Menegoz (IGE) for useful discussions. The authors are grateful to national services (MeteoSwiss, WSL/SLF, Météo-France, GLACIOCLIM and GLAMOS) for maintaining their long-term mountain observatories. IGE, LECA and CNRM/CEN are part of Labex OSUG@2020.