1 Introduction
In the late 1980s, in preparation for the US–French TOPEX/Poseidon mission [20], the French ‘Centre national d’études spatiales' (CNES) considered the opportunity of a new independent geodetic system for precise orbit determination. In close cooperation with the ‘Institut géographique national’ (IGN) and the ‘Groupe de recherche en géodésie spatiale’ (GRGS), CNES developed such an operational system called DORIS (Doppler Orbit Determination and Radiopositioning Integrated on Satellite) [16,29]. Truly optimized for orbit determination, DORIS is an up-link Doppler system. Radio signals are generated from a ground-tracking network, and Doppler measurements are performed on-board the satellite. Orbit determination can be done in real-time on-board or in a post-processed mode after downloading data to the ground. The development of this new technology was possible thanks to recent enhancement in accuracy of quartz time standards [13,35]. In particular, the goals for on-board oscillators (including mechanical, thermal, magnetic and radiative effects) are as follows, and were developed by the C-MAC Company:
- – for short-term stability: variance,
- – for medium-term stability: variance,
- – for long-term stability: the frequency should stay equal to the nominal value () with a maximum discrepancy of ±2.0 Hz during the whole life of the oscillator.
Since the launch of the SPOT-2 French remote sensing satellite on January 22, 1990 [3], carrying on-board a first DORIS receiver, the technology has changed and its applications have gradually and logically expanded from orbit determination to gravity-field determination, terrestrial reference frame maintenance and geodynamics. In particular, the International DORIS Service (IDS) [45] has been created in 2003 and is currently the DORIS counterpart of the International GNSS Service (IGS) [4] for Global Navigation Satellite Systems (GNSS), such as the Global Positioning System (GPS). Those scientific services (with ILRS, IVS and IERS) are now considered as the backbone of the newly created project of the International Association of Geodesy (IAG): Global Geodetic Observing System (GGOS) [38,52].
The goal of this paper is to present the current scientific applications of the DORIS system, to discuss their present accuracy and to present future evolutions.
2 The DORIS tracking network
Besides the stability of the on-board clocks and the precision of the Doppler measurements, the tracking network is a key factor in the success of the DORIS system. This network was deployed by the ‘Institut géographique national’ and is still maintained by its SIMB group (‘Service d'installation et de maintenance des balises’) [17,54]. The DORIS network was operational before the launch of the first DORIS satellite and has maintained since then a dense and geographically good distribution of tracking stations. Fig. 1 shows the current status of the DORIS tracking network as of January 1, 2005.
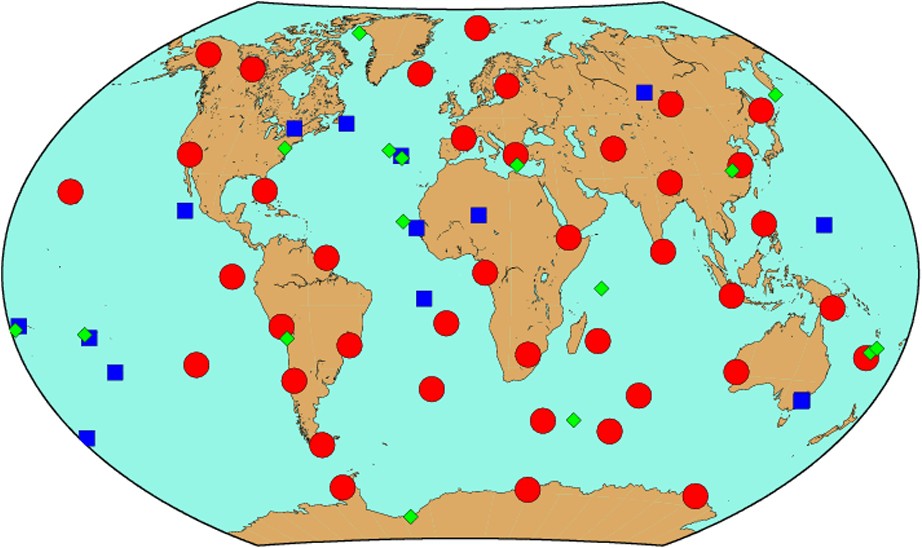
The DORIS tracking network (January 2005) (squares = more than 10 years of observations, circles = between 5 and 10 years of observations, diamonds = less than 5 years of observations).
Le réseau orbitographique DORIS (janvier 2005) (carrés = plus de 10 ans d'observations, cercles = entre 5 et 10 ans d'observations, losanges = moins de 5 ans d'observations).
A significant number of sites (40) have continuously observed for more than ten years. These sites are also geographically well distributed all over the Earth. Only 15 sites have observed between five to ten years, while 16 stations have been installed on new sites during the last five years. Fig. 2 represents a histogram of the distribution of the observations data span per station, showing how stable this network has been with time. Fig. 2 shows in more detail how stable the DORIS network has been since 1993.
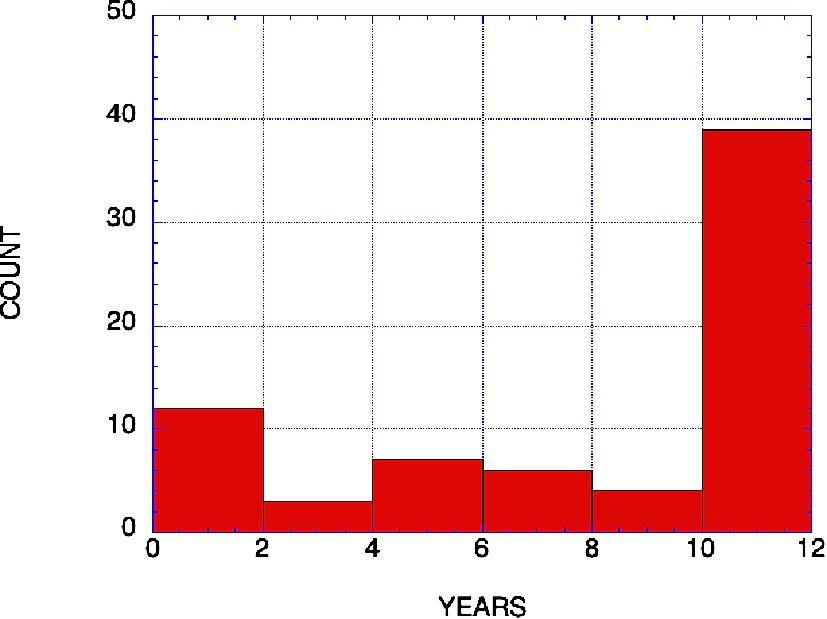
Histogram of DORIS observation data spans per station (January 2005).
Histogramme des durées d'observation DORIS par station (janvier 2005).
When selecting a new DORIS site and especially at the very beginning of the system, the availability of other space techniques to obtain precise a priori geodetic coordinates and velocities for the site was an important criterion. It is also a very important criterion for Terrestrial Reference Frame maintenance [1]. Fig. 3 provides the current geographical distribution of the geodetic collocations between DORIS and other space geodetic systems (VLBI, SLR, GPS). It can be seen that the DORIS–GPS collocations are the more numerous by far and are very important in the realization of the Terrestrial Reference Frame (TRF) [1,54]. The VLBI collocations are mostly in North America, for economic reasons. Several VLBI and SLR collocations were realized only temporarily through campaigns of mobile equipments and are no longer active collocations.
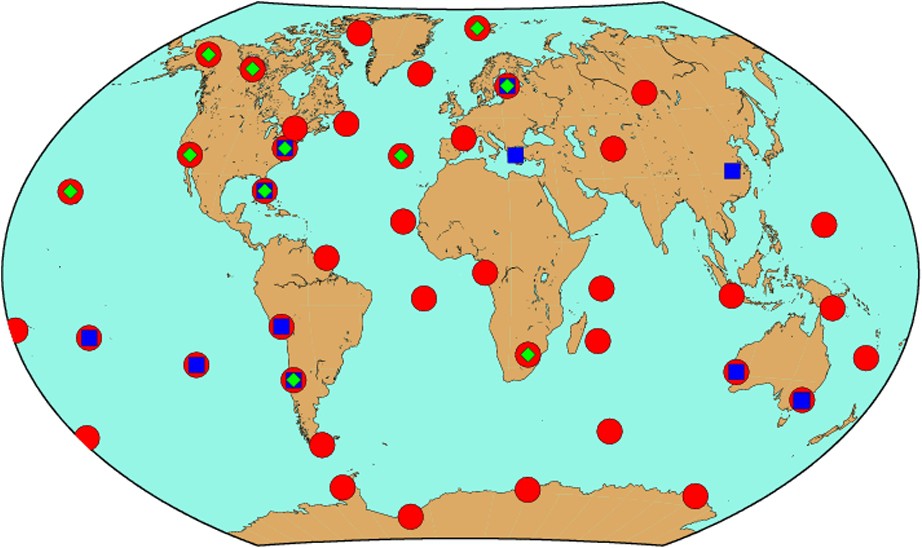
Geodetic collocations at DORIS tracking sites (January 2005) (squares = GPS, circles = SLR, diamonds = VLBI).
Colocations géodésiques sur les sites de poursuite DORIS (janvier 2005) (carrés = GPS, cercles = SLR, losanges = VLBI).
The local surveys between DORIS were performed or at least collected by the IGN using strict specifications. Table 1 displays a summary of the estimated precisions of these local tie surveys. A large number of them have been done with a sufficient care to be used for global geodetic techniques combinations or intercomparisons. It must also be noted that the DORIS stations are often located on remote islands, sometimes with only a few ships traveling there per year. This of course complicates the task of the geodetic survey teams. The few collocated sites for which local tie could not be performed or was performed with lesser accuracy correspond mostly to mobile VLBI or SLR stations that are no longer in existence.
Accuracy of the DORIS geodetic local ties toward other geodetic techniques (January 2005)
Précision des rattachements géodésiques des stations DORIS par rapport aux autres techniques (janvier 2005)
Excellent (⩽3 mm) | Poor (>3 mm) | No local tie | |
SLR | 7 | 1 | 4 |
VLBI | 7 | 0 | 4 |
GPS | 32 | 5 | 4 |
Total | 46 | 6 | 12 |
3 Precise orbit determination
DORIS is a globally distributed, all-weather system providing nearly continuous precise Doppler tracking of SPOT2, TOPEX/Poseidon, SPOT4, SPOT5, ENVISAT and Jason-1 satellites [34]. Precise Orbit Ephemeris (POE) orbits computed either by the Precise Orbit Determination and Production System (PODPS) at GSFC, or by the POD team at CNES, with different orbit determination software, have a radial accuracy of 2–3 cm for TOPEX/Poseidon, Jason-1 and ENVISAT, based on a combination of DORIS and Satellite Laser Ranging (SLR) tracking.
Orbits computed using only DORIS compare to the POEs to within 2 cm on the radial component [36].
The dense DORIS tracking allows a reduced-dynamic approach for POD [59]. In a dynamic approach, all forces applying on the satellite are supposed to be known or at least a limited number of additional parameters (e.g., atmospheric drag or solar pressure coefficients) are estimated during the orbit determination process, but the satellite position and velocity at the epoch of reference (start of the considered arc of data) are considered to be constant. In a reduced-dynamic approach, the satellite accelerations are estimated more frequently to give more freedom to the dynamic model itself, but with appropriate constraints [59]. The reduced-dynamic approach requires more data and also a continuous tracking is more appropriate, as more flexibility is introduced in the estimation process. However, when the data are of excellent quality, a reduced-dynamic is less affected by error models and the orbit will be more accurate. In the case of TOPEX, orbit overlap consistency and SLR data fits suggest that the preliminary ‘enhanced parameter’ POE offers an improvement over the ‘dynamic’ POE orbits. However, evaluation of the 2–3-cm orbits is also quite a challenge and requires new types of improved tests. Recently, such tests were done by comparing the results of different oceanographic missions, enabling the detection of previously unnoticed tiny geographically correlated errors.
DORIS offers a powerful satellite tracking capability, whose full potential for POD has yet to be realized. For example, at CNES, the DORIS-modeled observation is weakened by the current POD strategy of unconstrained adjustment of pass-by-pass measurement and troposphere biases. The application of crude constraints dramatically increases the level of signal in the DORIS residuals, and in the case of the troposphere, improves the orbit [48] as well as the geodetic derived results [54]. The investigation conducted at CNES, aiming at strengthening the modeled observation, continues to try to improve operational POD accuracy.
On a more general point of view, POD results could be improved by adopting better models or by using a more refined data analysis strategy. The gravity field models are now much more accurate since the use of the GRACE data, and improvements are foreseen in the surface force models (atmospheric drag, solar pressure, albedo) as well as in the measurement model (use of quaternions for the spacecraft attitude, better phase centre determination and monitoring [56]). In particular, better force models could improve the centering of the derived orbits [9], which is of critical importance for oceanographic studies. It could also improve geocenter monitoring derived from time series of station coordinates as discussed later. Other aspects still under discussion are proper weighting between tracking techniques [9] and parameter time constraints [55].
The mission objective for TOPEX/Poseidon and ENVISAT has been largely fulfilled with 2–3-cm POE orbits routinely produced. Further improvements are under study in order to take greater advantage of the dense tracking DORIS has to offer, possibly leading to more accurate POEs. For example, there is presently some discussions between POD groups using multi-technique analysis (DORIS/SLR/GPS) as they all need to estimate an additional parameter in their estimation that is equivalent to a DORIS time tagging offset, whose effect can be seen in the along-track component of the satellite if not taken into account (Zelensky, personal communication).
Table 2 summarizes the different POD results obtained for DORIS satellites. Major improvements were done after the adoption of a new generation of gravity fields [5,42] based on data from the GRAvity and Climate Experiment (GRACE) mission [43].
Past and current satellite missions carrying an on-board DORIS receiver and precise orbit determination performances (1G = first generation, 2G = second generation, 2GM = second generation miniaturized)
Missions spatiales passées et présentes comportant un récepteur DORIS embarqué et performances de la détermination précise d'orbite associée (1G = première génération, 2G = deuxième génération, 2GM = deuxième génération miniaturisée)
Satellite | Launch | Receiver generation | Other on-board tracking system | Altitude (km) | Orbit precision (radial RMS) (cm) | References |
SPOT-2 | Jan. 1990 | 1G | – | 830 | 3 | [16,18] |
TOPEX/Poseidon | Aug. 1992 | 1G | SLR + GPS | 1330 | 2 | [36,59] |
SPOT-3 | 1994 | 1G | – | 830 | 3 | |
SPOT-4 | 1998 | 1G | – | 830 | 3 | |
Jason | Dec. 2001 | 2GM | SLR + GPS | 1330 | 1 | [9,23,32] |
ENVISAT | Mar. 2002 | 2G | SLR | 800 | 2 | [30,60] |
SPOT-5 | 2002 | 2GM | – | 830 | 3 |
4 Real-time orbits
4.1 General purposes
DIODE (Doris Immediate On-board orbit DEtermination) is a series of on-board orbit determination software integrated into the DORIS receivers. DIODE processes the DORIS measurements on-board as soon as they are performed in order to estimate the position of the carrier satellite and to synchronize the DORIS receiver with TAI atomic time.
The first goal of DIODE was to allow the DORIS receiver to provide a real-time estimate of the satellite position and velocity to the Attitude and Orbit Control System (AOCS), and also to other instruments in the payload, or even to the ground system (after inserting this information in the telemetry).
But DIODE is also used to increase the onboard autonomy of the DORIS system. Knowing the orbit allows the on-board software to predict the next beacon(s) to be visible to the satellite, then to operate an optimal selection and program the receiver loops. DIODE has allowed the complete removal of the daily beacon mission uploads generated by the ground system.
Last but not least, DIODE estimates the synchronization of the DORIS receiver with respect to TAI time allowing the DORIS receiver to accurately time-tag an external signal sent by the satellite AOCS. DORIS returns the TAI date of this signal, with an accuracy of a few microseconds. The DORIS receivers may thus be considered as an on-board clock.
4.2 The SPOT-4 mission
The first DORIS receivers (SPOT-2, SPOT-3, TOPEX/Poseidon – hereafter called T/P) were not fitted with a navigation function. DIODE was first flown on SPOT-4 (launched on March 24, 1998). The SPOT-4 experiment was successful [10,25,26]: DIODE/SPOT-4 is still operating more than six years after its launch, in a preliminary version, used as proof-of-concepts and already performing at a few meters accuracy on the position determination. Its results are daily used for SPOT image rectification and for POAM3 pointing (the Polar Ozone and Aerosol Measurement instrument is a US Naval Research Laboratory payload dedicated to atmosphere monitoring [31]).
4.3 The Jason-1 mission (SPOT-5 and ENVISAT)
Within the Jason-1 mission, DIODE navigation bulletins are used:
- – to geolocate altimeter and radiometer measurements in real time,
- – to give a real-time estimate of the radial component of the satellite orbit.
The specified accuracy was 30 cm RMS on the radial component, and 1 m RMS in three dimensions (3-D), and the observed accuracy is better than the initial specifications. During routine phases, the real-time orbit is used on the ground to produce Operational Science Data Records [14] generated and distributed within 3 h to operational oceanography and meteorological centers throughout the world.
The Time-Tagging Bulletins are used to time tag the Jason-1 altimeter measurements. A level of accuracy of 100 μs (with a goal of 10 μs) was specified [37], and the result is below 5 μs. DIODE/Jason-1 has been operating for more than 30 months without interruption (except a planned interruption to upload a software update), and its observed availability is 99.8%. It is still operating today, and very good results have also been demonstrated during exceptional events (maneuvers, satellite safe-holds modes) [28]. Similar results are obtained from DIODE/SPOT-5 and DIODE/ENVISAT missions [27].
4.4 Future missions (CRYOSAT, Pléiades and Jason-2)
A new DORIS 2GXX generation is being developed for Jason-2, Pléiades and later satellites, with important enhancements in the DORIS system [44] and in the navigation software [28].
The most significant improvements are:
- – adoption of the J2000 reference system as the inertial frame in which the numerical integration is performed (instead of Veis quasi-inertial frame);
- – adoption of the GRIM5-C1 Earth gravitational field to model Earth attraction forces [22];
- – addition of the contribution of Earth tides to the calculation of the beacon coordinates;
- – modeling of DORIS measurements has been re-written in order to avoid unnecessary simplifications.
In terms of processing control, editing of spurious measurements and failure detection and recovery has been reinforced.
In terms of output products, a new J2000 inertial bulletin (estimated position and velocity in the inertial frame) is generated to help AOCS systems in their operations. Even if the specified accuracy is 500 m, a few meter accuracy is expected on-board the ESA/CRYOSAT mission1.
As usual, the future DIODE flight version has first been validated on-ground. To do so, we use real DORIS measurements gathered from the different satellites carrying the DORIS tracking system. Below are displayed the results for a TOPEX twelve-day arc (September 2004) (Fig. 4).
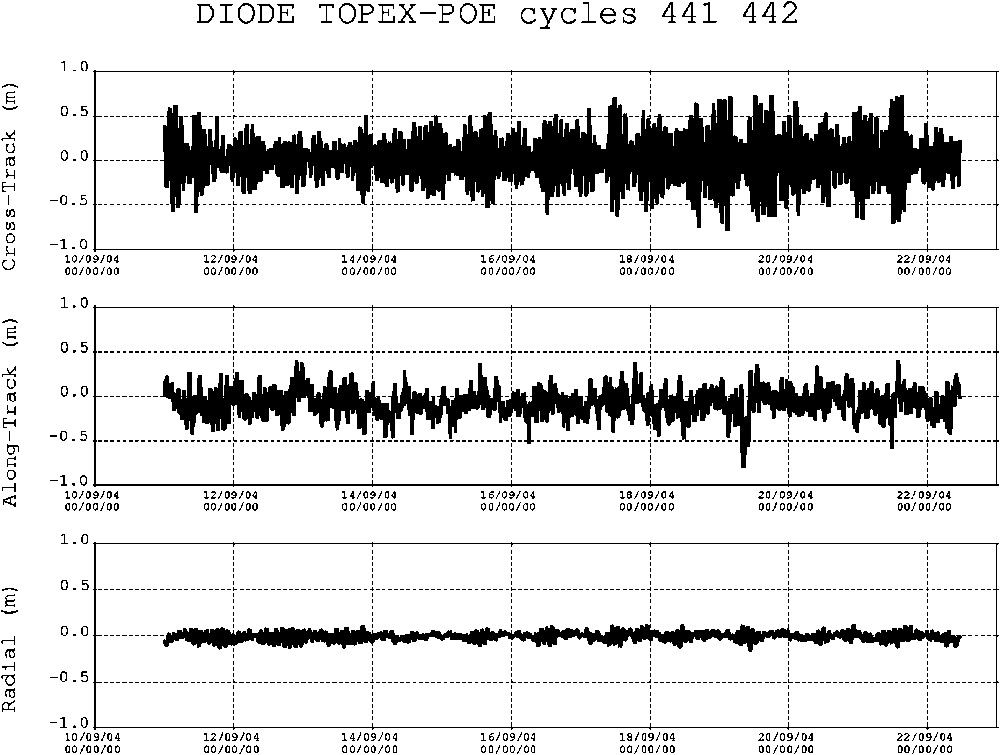
Expected position accuracy for the coming DIODE version using actual TOPEX/DORIS data in a post-processing mode (September 11 to September 22, 2004).
Exactitude de position attendue de la version actuelle du logiciel DIODE en utilisant des données réelles DORIS/TOPEX en mode post-traitement (du 11 au 22 septembre 2004).
The statistical results of the comparison are listed in Tables 3 and 4. As numerical issues have been solved with the new ERC32 processor, it should then be possible to produce better than 5-cm orbits on-board Jason-2 with an expected 100% availability of the products. However, these results may be worse in the case of actual Jason-1 data due to possible sensitivity of the on-board clock to radiation over the South Atlantic Anomaly (SAA) [57,58].
Overall comparison statistics (DIODE–POE) from DORIS/TOPEX data. September 11 to September 22, 2004. All units are in mm for position and mm s−1 for velocity
Statistiques globales de comparaison (DIODE–POE) en utilisant des mesures DORIS/TOPEX. Du 11 au 22 septembre 2004. Toutes les unités sont en mm pour les positions et en mm s−1 pour les vitesses
Minimum | Maximum | Mean | Standard deviation | |
Radial | −154 | 122 | −6 | 44 |
Tangential | −801 | 406 | −73 | 137 |
Normal | −783 | 734 | 11 | 252 |
3-D error | 8 | 832 | 266 | 138 |
Radial velocity | −0.34 | 0.67 | 0.07 | 0.12 |
Tangential velocity | −0.12 | 0.12 | 0.00 | 0.04 |
Normal velocity | −0.80 | 0.69 | 0.00 | 0.23 |
3-D velocity error | 0.01 | 0.82 | 0.24 | 0.13 |
Day-by-day orbit comparison (DIODE–POE) from DORIS/TOPEX data. September 11 to September 22, 2004. All units are in mm
Comparaisons journalières d'orbites (DIODE–POE) issues de données DORIS/TOPEX. Du 11 au 22 septembre 2004. Toutes les unités sont en mm
Day | Minimum | Maximum | Mean | Standard deviation |
September 11, 2004 | −131 | 85 | −16 | 51 |
September 12, 2004 | −126 | 88 | −18 | 52 |
September 13, 2004 | −121 | 108 | −12 | 49 |
September 14, 2004 | −92 | 62 | −7 | 27 |
September 15, 2004 | −108 | 82 | −2 | 35 |
September 16, 2004 | −109 | 104 | 4 | 41 |
September 17, 2004 | −119 | 111 | 2 | 49 |
September 18, 2004 | −121 | 118 | 3 | 41 |
September 19, 2004 | −154 | 104 | 0 | 44 |
September 20, 2004 | −99 | 122 | −4 | 38 |
September 21, 2004 | −123 | 109 | −8 | 46 |
September 22, 2004 | −118 | 54 | −27 | 39 |
Table 4 presents the day-by-day comparison over the same period. Please notice the 27 mm obtained on September 14.
Other current developments:
- – the number of channels in the DORIS receiver will be increased from 2 to 7, in order to perform more measurements. A very positive impact on system robustness as well as on orbit and positioning accuracy is of course expected from such an improvement of the DORIS system;
- – the latest issue of the navigation function now takes into account the measured attitude quaternions instead of using a simplified attitude model. This will allow a better monitoring of the center of phase–center of mass vector, and maybe a better modeling of the surface forces due to improved orientation in space;
- – a new altimetric bulletin will include geodetic information about the position of the satellite. Latitude, longitude, and altitude will be provided with respect to the geoid. Such information can be used with profit by altimeter instruments such as Poseidon-3 to be embarked onboard Jason-2/OSTM. Indeed, the on-board provision of such an altimetric bulletin to the altimeter instrument should help the altimeter to stay locked continuously over any desired target. The expected distance from the target will be directly computed by the altimeter instrument, by combining the altitude information computed by the DORIS receiver and a digital elevation model stored on-board. This can be used to track specific areas of interest over continents such as inland waters, which may not be properly tracked by the usual altimeter tracking systems;
- – osculating parameters (instantaneous Keplerian orbital elements that would apply if all force perturbations would be stopped) will also be delivered to the users to improve interpolation.
4.5 Conclusion
Four different DIODE versions are flying today, allowing real-time precise and reliable results. DORIS on-board orbit computation has become an operational facility, with good accuracy and convincing reliability. As minimizing ground operations and processing is one of the trends of the operational space systems, it then remains important to continue improving the onboard DORIS/DIODE capabilities. In this way, besides performing very accurate measurements that contribute to scientific applications, the DORIS receivers are able to provide attractive products to their carrier satellites, and the associated ground systems. These improvements will enhance the spectrum of flight opportunities and hopefully contribute to a larger number of DORIS instruments flying on different orbits. In return, this ‘constellation of DORIS receivers’ will contribute to a more accurate positioning of the beacon network, leading to better monitoring of the ‘DORIS Earth Reference System’.
5 Geodetic positioning accuracy
Instead of using the stations coordinates as known values in the POD estimations, it is also possible to use the DORIS data for geodetic purposes [8,11,41,48] and to estimate the positions (and eventually the velocities) of the DORIS tracking stations. In such a case, the satellites orbits and the station positions are estimated simultaneously in a combined free-network adjustment [24].
The precision of the geodetic results depends on the accuracy of the different model used (gravity field, solar pressure, Earth rotation...), but also on the amount of DORIS data available for this specific station.
Fig. 5a–c describes the sensitivity of the DORIS geodetic accuracy to the considered observation period in latitude, longitude and height. We have processed the DORIS data on daily basis during several months and we have computed for each day the position of all stations of the DORIS network using all data from the first day of this test to the considered day. We have then compared for each individual realization (from 1 day to 5 months) the derived geodetic reference frame (coordinates of all stations) to a better reference expressed at the mean epoch of observation (IGN04D02) [51].
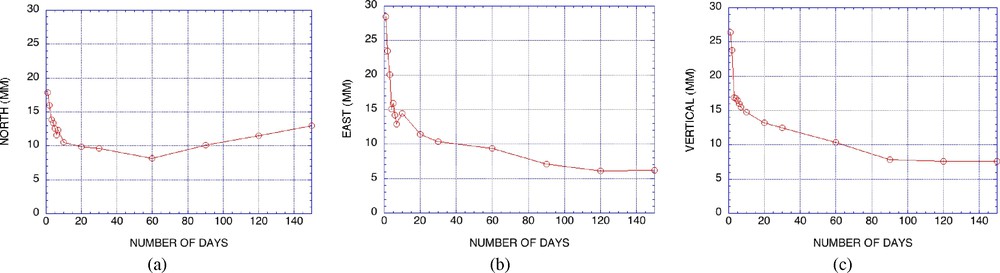
DORIS positioning precision with regard to the duration of observations ((a) in latitude, (b) in longitude, (c) in height). Days after February 1, 2004.
Précision de la localisation DORIS en fonction de la durée des observations ((a) en latitude, (b) en longitude, (c) en altitude). Nombre de jours à partir du 1er février 2004.
Fig. 5a–c shows that the precision of the results is directly linked to the duration of the DORIS observations. One-day solutions provide 20–30 mm precision, while 7-day solution as currently provided to IDS provide 10 mm or better accuracy, making the DORIS technique very competitive with other techniques such as GPS. The observed degradation in Fig. 5 after several months is due to the fact that we do not take into account tectonic plate motions in this simple test. It must be noted that this recent improvement on precision was obtained when using the recent GRACE-derived gravity fields [43,50].
Another aspect related to the quantity of DORIS data per station during a fixed period of observation is the number of available satellites during the period of observation. Since 1993, most of the geodetic results were obtained using a limited constellation (2 satellites). Since June 2002, five satellites are now available for geodetic positioning (SPOT-2, TOPEX, SPOT-4, SPOT-5 and ENVISAT). Unfortunately, the Jason data cannot be used for geodesy due to an increased sensitivity to radiation of the oscillator over the South Atlantic Anomaly (SAA) [57,58].
In the following test, we have used the data for a specific week (February 1–7, 2004) but similar results can be obtained for any other week of DORIS data. As data from five different satellites were available for this specific week, we processed the data using either one satellite (5 choices = 1 per satellite), two satellites (10 choices), three satellites (10 choices), four satellites (10 choices) or five satellites (only 1 choice = all available satellites). For each individual solution, we have compared the position of all DORIS stations with our internal reference (IGN04D02) as before and derived a unique Weighted Root Mean Square (WRMS) value for this specific week.
Fig. 6 shows that the precision of the geodetic DORIS results improves with the size of the DORIS constellation: five satellites provide better results than one, even if the improvement is not linear and even if, for some specific cases, solution with two satellites (usually two SPOTs) would provide better results than solution with three satellites (usually including TOPEX). In general, SPOTs (98° inclination) provide better geodetic results than TOPEX (66° inclination) for stations at very high latitude because they generate more data for these stations during one week. Station positions at high latitude are also poorly determined by inclined satellites, due to the specific geometry of the passes, making it difficult to distinguish between all position components. It can also be seen that individual satellites provide different levels of geodetic precision: one satellite can provide for this specific week station coordinate accuracies ranging from 14 to 36 mm depending on the satellite orbit (better results for SPOTs and worse for TOPEX for reasons explained above). The best single-satellite solutions are obtained with SPOT-5 (equipped with a newer generation on-board receiver). Testing on a few other weeks indicates that, even though the results themselves slightly change from week to week, the general conclusions discussed above remain the same.
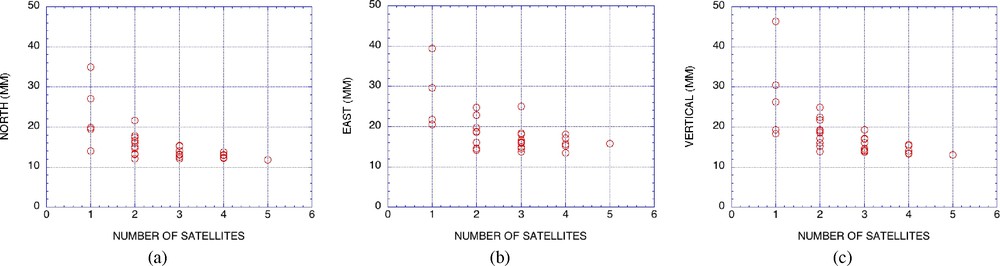
DORIS positioning precision with regards to number of available satellites ((a) in latitude, (b) in longitude, (c) in height). Single week results (February 1–7, 2004). All possible choices for satellites selection were considered.
Précision de la localisation DORIS en fonction du nombre de satellites disponibles ((a) en latitude, (b) en longitude, (c) en altitude). Résultats obtenus à partir d'une seule semaine de mesures (du 1er au 7 février 2004, en considérant tous les choix possibles de combinaisons de satellites).
Another way to look at the impact of the size of the DORIS constellation on the accuracy of the derived station coordinates is to consider all the weekly results available for the full considered DORIS data set (since January 1993) and to classify the results according to the total number of satellites available per week. Table 5 provides a detailed summary of these weekly comparisons to ITRF2000 [1] or IGN04D02 reference after grouping the results with regards to the size of the DORIS constellation. In both cases, a clear improvement can be seen, with the increase in satellite availability, confirming the previous results (Fig. 6), but on a much longer time span (12 years vs. 1 week). Comparisons with IGN04D02 perform better as this reference is directly based on those same DORIS results. ITRF2000 may also degrade with time, due to normal imprecision in the velocities (positions in 2005 are extrapolated beyond the last data in 2000) [51].
Assessment of the accuracy of the DORIS weekly positions as a function of the size of the DORIS satellite constellation, using different cumulative solutions as reference. From January 1993 to October 2004
Estimation de l'exactitude des positions hebdomadaires DORIS en fonction du nombre de satellites de la constellation DORIS, et en utilisant plusieurs solutions cumulatives comme référence. De janvier 1993 à octobre 2004
Number of DORIS satellites | Number of weeks | ITRF2000 | IGN04D02 | ||||
North (mm) | East (mm) | Vertical (mm) | North (mm) | East (mm) | Vertical (mm) | ||
1 | 1 | 40 | 41 | 42 | 33 | 31 | 40 |
2 | 167 | 27 | 32 | 31 | 26 | 30 | 26 |
3 | 321 | 25 | 29 | 27 | 23 | 26 | 21 |
4 | 4 | 22 | 20 | 26 | 19 | 20 | 16 |
5 | 120 | 18 | 16 | 23 | 14 | 15 | 13 |
6 Terrestrial reference frame maintenance
In the past few years, the whole concept of maintenance of the Terrestrial Reference Frame (TRF) has slowly changed. Previously, the TRF was defined as a set of station positions and velocities for a limited number (less than a few hundred) of fundamental geodetic stations all over the Earth [2,33]. The so-called maintenance of the frame was done by computing these solutions typically on a yearly basis to take into account the increase in data availability as well as the improvements in accuracy regularly obtained by the numerous Analysis Centers for the different techniques. More recently, instead of realizing the reference frame through a set of positions and velocities of a large number of stations (cumulative solution such as IGN04D02, for example), the notion is now evolving into time series of station coordinates [2]. The concept of the origin of the frame is also going though intense discussions and very precise definitions must now be provided [6,7,12,15,54]. DORIS provides valuable and independent information for all these investigations.
7 Earth orientation
DORIS can also be used to estimate the Earth Orientation Parameters (EOPs), and more specifically, the Earth's Polar Motion. As for the other geodetic results, we will show that the number of available DORIS satellites is critical to the accuracy of the results.
We have conducted for these parameters the same study as previously done for the station coordinates. In a first step, we have selected a specific week in which data from 5 satellites are available. We then estimate these parameters using only 1 to 5 satellites and looking at all the possible choices as before. In this case, we compared the DORIS X-POLE (resp. Y-POLE) with the values derived independently from a GPS analysis (JPL solution [49]). For each possible choice, we have estimated a Weighted Root Mean Squares (WRMS) of the observed differences (daily DORIS–GPS) over the seven days of the week without removing any bias or drift. We implicitly assume that the DORIS and GPS-derived EOP time series are expressed in the same Terrestrial Reference Frame.
Fig. 7 shows that the accuracy of the DORIS-derived polar motion strongly depends on the availability of DORIS satellites. The point on Fig. 7b (one satellite) that looks like an outlier corresponds to a limited satellite dataset (50% of the data were lost for this specific week for this satellite).
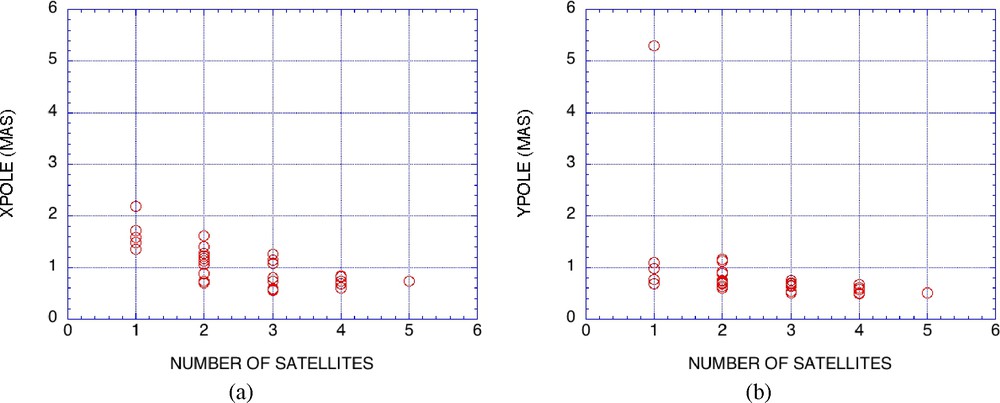
DORIS precision of the Earth Orientation Parameters with regards to number of available satellites ((a) in X, (b) in Y). Single week results (February 1–7, 2004). All possible choices for satellites selection were considered.
Précision obtenue par DORIS des paramètres de la rotation terrestre en fonction du nombre de satellites disponibles ((a) en X, (b) en Y). Résultats obtenus à partir d'une seule semaine de mesures (du 1er au 7 février 2004), en considérant tous les choix possibles de combinaisons de satellites.
In a second step, we have considered all weekly results of this type, taking into account for each week all available satellites (since 1993). We have then grouped the results not by time but by the number of available satellites available during each week. Table 6 shows a direct comparison of the DORIS/IGN-JPL polar motion time series with the GPS/JPL time series (no bias or drift removed) using two different reference frames ITRF2000 and IGN04D02 to express the results.
Assessment of the accuracy of the DORIS-derived polar motion as a function of the size of the DORIS satellite constellation using different cumulative solutions as reference. From January 1993 to October 2004
Estimation de l'exactitude de la position du pôle de la rotation terrestre dérivée des mesures DORIS en fonction du nombre de satellites de la constellation DORIS, en utilisant plusieurs solutions cumulatives comme référence. De janvier 1993 à octobre 2004
Number of DORIS satellite | Number of weeks | ITRF2000 | IGN04D02 | ||
X-Pole (mas) | Y-Pole (mas) | X-Pole (mas) | Y-Pole (mas) | ||
1 | 1 | 2.03 | 1.53 | 2.05 | 1.56 |
2 | 167 | 2.18 | 1.65 | 2.12 | 1.71 |
3 | 341 | 1.94 | 1.40 | 1.94 | 1.42 |
4 | 4 | 1.87 | 0.95 | 1.84 | 1.00 |
5 | 120 | 1.64 | 0.91 | 1.62 | 0.98 |
Table 6 confirms the previous results obtained on a limited dataset (Fig. 7). Sub 1 milli-arcsecond (mas) is presently achievable with the current DORIS system when a sufficient number of satellites is available. These results represent quite an improvement on previous submissions (2 mas estimated over the full 1993–2004 period [21], as the author did not consider possible improvement during time due to the successive changes in the DORIS constellation). In Table 6, very little difference can be found with the choice of the reference frame, showing that larger errors can be found presently in the DORIS data processing and cannot be attributed to the frame.
8 Sensing the atmosphere
It is also possible to derive from the DORIS data information on the atmosphere, both for the lower part (troposphere) and for its upper part (ionosphere). Concerning the troposphere, early studies [53] have shown that precision of 1 cm in zenith path delay can be obtained using three satellites (equivalent to 2 mm in Integrated Precipitated Water). More recent studies show that 6 to 8 mm can be obtained when using five satellites during the CONT02 campaign in comparison with VLBI and GPS results [39,40]. However, we must also say that these results can only be obtained after a one-month delay, making them totally useless for any assimilation in a weather prediction model. However, for climatology studies, DORIS could play a role as it provides an external reference for long time periods. DORIS is also less subject than GPS to antenna changes or equipment modifications such as radome changes.
It is also possible to use the data from the two DORIS frequencies (2 GHz and 400 MHz) to derive information on the ionosphere and more specifically on the Total Electron Content (TEC) in the direction of sight of the satellite. This can be used to derive ionospheric corrections in the case of a single-frequency altimeter like Poseidon on the TOPEX satellite [19]. It can also be used to derive information on the protonosphere (over 1000 km) as the DORIS satellites are lower (800 to 1300 km) than the GPS satellites (20 000 km) and they do not probe the totality of the ionosphere [47]. It is also possible to study ionospheric perturbations related to geophysical activities, such as earthquakes [46].
9 Conclusions
Since the launch of SPOT-2, the DORIS system has been used for Orbit Determination and for Geodesy or Geophysics. For Precise Orbit Determination, several important steps were taken in the last few years, specially using GRACE-derived gravity field, in order to obtain a 2-cm orbit on the radial component in post-processing mode or sub-centimeter when combined with GPS and Laser Ranging. Real-time orbits are currently derived with 20-cm precision for the radial component and are extremely reliable. This study shows that 5-cm level of performance could be obtained with Jason-2 with proper software upgrades. For geodesy and geodynamics, the precision of the station coordinates depends on the number of available DORIS satellites. Best results are currently obtained using 5 satellites and show that sub centimeter precision for weekly coordinates are now at reach, potentially corresponding to 1–2 mm yr−1 precision in station velocity determination since 1993. Polar motion estimations can now be obtained daily with sub milli-arcsecond precision. With its dense and permanent tracking network, DORIS should play a significant role in the realization of the Global Geodetic Observing System.
Acknowledgements
Part of this work was carried out at the Jet Propulsion Laboratory, California Institute of Technology, under a contract with the National Aeronautics and Space Administration.
1 Note added at proof stage: The CRYOSAT launch was not successful.