1 Introduction
Cameroon is underlain by Cretaceous to Recent volcanosedimentary formations and by a plutonometamorphic basement. This basement comprises the northern edge of the Archean Congo craton to the south (Ntem complex) and the Panafrican formations that occupy more than the half of the national territory (Fig. 1). Many petrographic, chemical, isotopic, and geochronological studies [29,46,54] were carried out in the Ntem complex. The Panafrican fold belt, defined in the 1960s and 1970s [5,16], is constituted by Neoproterozoic and Palaeoproterozoic formations that have been reworked during the Panafrican orogeny. During the 1980s and 1990s, petrographic, structural, chemical and geochronological studies were carried out in the northern [31,33,34,44,59] and southern parts [12,28,36,37,42,53] of the Panafrican fold belt of Cameroon. The northern part is made up of juvenile Neoproterozoic and Palaeoproterozoic material (660- to 580-Ma-old granitoids, 800-Ma-old orthogneisses) without contribution of Archean materials. The southern part is made up of 620-Ma-old schists and gneisses, and ∼700-Ma-old volcanic derivatives; these rocks were derived from juvenile materials contaminated by Palaeoproterozoic crust. Since the end of the 1990s, attention also focused on the geodynamic evolution of the fold belt [7,32,34,38,52,57].
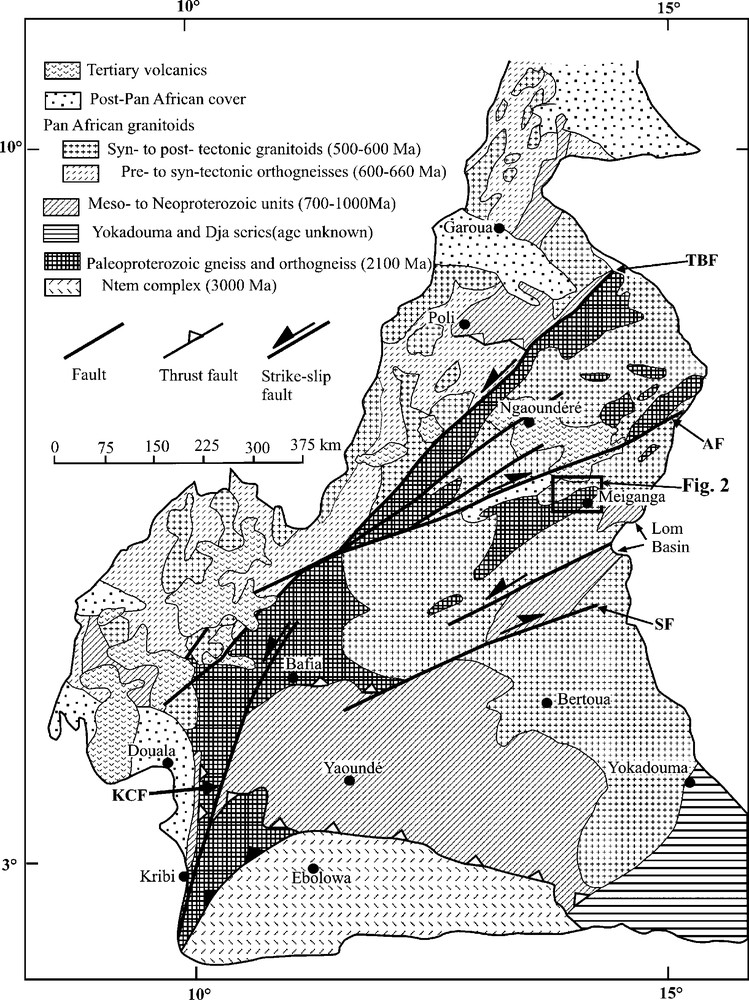
Geological map of Cameroon (after [58]) showing the major lithotectonic units. KCF, Kribi–Campo fault; AF, Adamaoua fault; SF, Sanaga fault; TBF, Tcholliré–Banyo fault.
Fig. 1. Carte géologique du Cameroun (d’après [58]) montrant les grands ensembles lithologiques. AF, faille de l’Adamaoua ; KCF, faille de Kribi–Campo ; SF, faille de la Sanaga ; TBF, faille de Tcholliré–Banyo.
The models of continental crust evolution are ill defined, since they all depend on poorly known parameters concerning the proportion of Archean material in the crust [1]. Indeed this assessment is difficult, because the segments of the various orogenies consist of ancient recycled crust associated with juvenile crust issued from the mantle. The present study is aimed at identifying Archean material in the Méiganga area (central Cameroon), which is composed of a 2.1-Ga-old metamorphic basement reworked during the Pan-African orogeny. The latter is characterized by the emplacement of granites within the 650–550-Ma time interval. A two-step approach has been used, based on:
- (a) the behaviour of the major elements, Y and REE, which allows one to characterize the Archean crust composed of a tonalite—trondhjemite—granodiorite (TTG) association;
- (b) after establishing the zircon typology through cathodoluminescence, 207Pb/206Pb dating on single zircon crystals, revealing the different phases of zircon growth, which are assumed to correspond to successive orogenies.
2 Geological setting
The Méiganga area (Fig. 2) belongs to the Adamawa region, in the central part of the Panafrican fold belt of Cameroon; it is underlain by syn-tectonic, late-tectonic, and post-tectonic granitoids [22,58], hosted in metamorphic formations. The granitoids are partially covered by basaltic lavas or crosscut by phonolitic domes [35]. Early data from the granitoids yielded ages in the 500–600-Ma range [4]. Recent Th–U–Pb monazite dating in the Ngaoundéré region yielded ∼615 ± 27 Ma and ∼575 ± 8 Ma for the emplacement of biotite–muscovite granite and biotite granite, respectively [56]. The metamorphic host rocks comprise metasediments and ∼2.1-Ga-old orthogneisses, which were reworked during the Panafrican orogeny [58]. U–Pb dating on metaigneous rocks (hornblende–biotite gneiss) of the Mbé region to the north of Ngaoundéré gives an age around 2.1 Ga [43]. In the Méiganga area, the basement comprises also banded amphibolites, amphibole biotite gneisses, and pyroxene amphibole gneisses. Banded amphibolites consist of quartz–feldspar layers alternating with amphibole-rich layers; amphibole–biotite and pyroxene–amphibole gneisses are made up of quartz, plagioclase, K-feldspar, biotite, hornblende, pyroxene, and accessory minerals (sphene, zircon, and apatite).
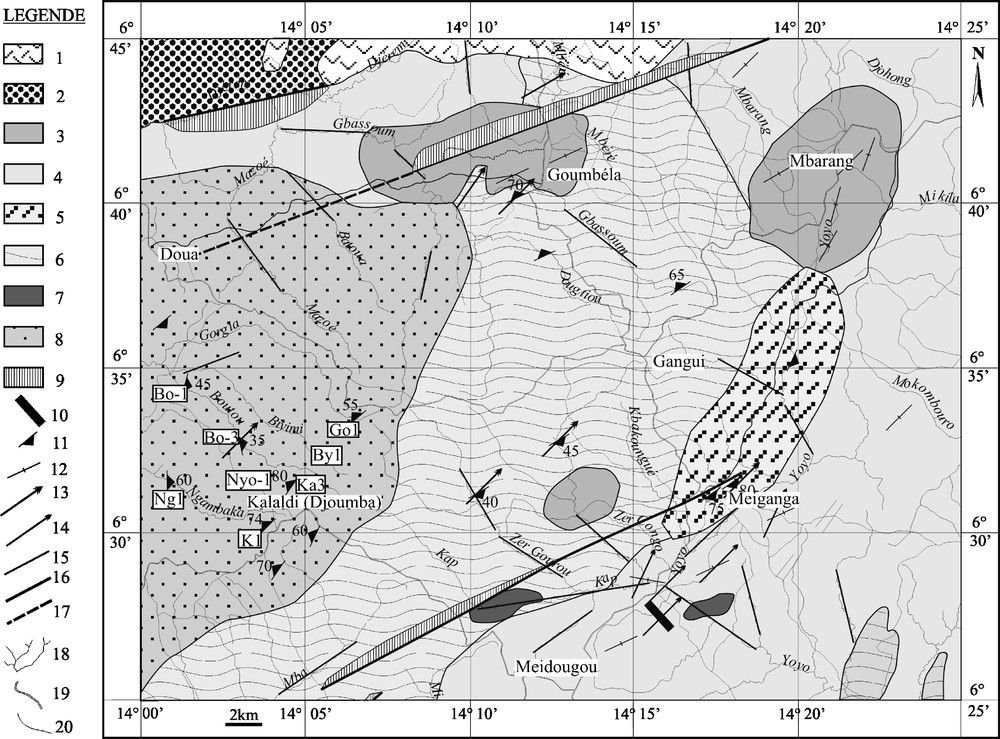
Geological map of the Méiganga area (after [13]) showing the position of the studied samples around the Kalaldi village: (1) basalt, (2) conglomerate, (3) biotite–muscovite granite, (4) pyroxene–amphibole–biotite granite, (5) banded amphibolite, (6) amphibole–biotite gneiss, (7) amphibolite, (8) pyroxene–amphibole gneiss, (9) mylonite, (10) dolerite, (11, 12) schistosity, (13, 14) lineations, (15) fractures, (16) fault, (17) supposed fault, (18) rivers, (19) road, (20) path.
Fig. 2. Carte géologique de la région de Méiganga (d’après [13]) montrant la position des échantillons analysés autour du village Kalaldi. (1) basalte, (2) conglomérat, (3) granite à biotite et muscovite, (4) granite à pyroxène, amphibole et biotite, (5) amphibolite rubanée, (6) gneiss à amphibole et biotite, (7) amphibolite, (8) gneiss à pyroxène et amphibole, (9) mylonite, (10) dolérite, (11, 12) schistosités, (13, 14) linéations, (15) fractures, (16) faille, (17) faille supposée, (18) cours d’eau, (19) route, (20) piste.
The studied samples were collected to the southwest of Méiganga, around Kalaldi, and to the southeast of Doua (cf. Fig. 2). The samples are pyroxene–amphibole-bearing gneisses, with a fine- to medium-banded structure. The banded structure is often reinforced by migmatisation, the melts being characterized by light quartzofeldspatic bands. The gneisses contain layers of amphibolite and sometimes enclaves of massive mafic rocks. Under the microscope, they show grano-nematoblastic to granoblastic structure and are made up of pyroxene, amphibole, biotite, quartz, feldspar, opaque minerals, and accessory minerals (apatite, titanite, and zircon). Pyroxene is xenomorphic in shape and often rimmed by secondary hornblende. Amphibole is a green hornblende, associated with dark brown flakes of biotite, which are often deformed. The rocks contain epidote (probably derived from the anorthite component of plagioclase), and biotite is partly transformed into chlorite. Plagioclase is 0.15 to 10 mm long, with oscillatory zoning; it is often affected by fractures. Quartz forms polycrystalline aggregates and shows undulatory extinction. It is generally an interstitial mineral.
3 Analytical methods
Major and trace elements were analysed by X-ray fluorescence (XRF) at the University of Tubingen. Rare-earth elements were analysed by Inductively Coupled Plasma–Atomic Emission Spectrometry (ICP–AES) at the CRPG (‘Centre de recherches pétrographiques et géochimiques’, Vandœuvre-lès-Nancy, France). Analytical uncertainties are estimated at ± 1% for major elements and 5–10% for most trace elements.
Zircon grains were separated from 200–63-μm sieved rock fractions by standard separation techniques (milling, wet shaking table, magnetic and heavy liquid separation) and finally handpicked under the binocular microscope. Cathodoluminescence images were performed on an electronic microscope LEO Model 1450 VP (variable pressure) 4-Quadrant BSE-Detector working with an accelerating voltage of 10 kV.
For single-zircon Pb evaporation, whole zircon grains were analysed using a double Re filament configuration. Principles of the evaporation method are described in [19,20]. Measurements were done using a Finnigan MAT 262 mass spectrometer equipped with a MassCom ion counter at the University of Tubingen. The linearity of the amplifier was controlled by the NBS standard 981. All 207Pb/206Pb ratios were corrected for common Pb according to [9], and the error for a single zircon age was calculated after [50]. Repeated measurements on natural zircon from Phalaborwa, South Africa [21], and from zircon standard 91500 of ‘Kuehl Lake’ (Canada) [63] were performed for geologically realistic age and error treatment [8]. The results were similar to those obtained by previous authors [21,63].
4 Geochemical characterization of TTG association in the Méiganga area
TTGs are intermediate to acid rocks (SiO2 > 56%) of andesite/diorite, dacite/granodiorite or rhyolite/granite type. The studied samples are intermediate to felsic, with 58% to 69% SiO2 (Table 1). The Al2O3 concentration varies between 13% and 17%. The K2O concentration increases with increasing SiO2, from 0.90% to 3.5%. The studied gneiss displays a medium- to high-K calc-alkaline character [14,23]. The ASI (A/CNK = [Al2O3/(CaO + Na2O + K2O) mol%]) indicates metaluminous composition, except for the sample Go-1, with ASI = 1.04. However, ASI values ≤ 1.1 point to an I-type character (e.g., [62]) for these samples. The normative An–Ab–Or and Q–Ab–Or diagrams (Fig. 3a and b) show the studied samples in comparison with the Ebolowa TTG [29] and Sangmelima granodiorite [27] in the Archean Ntem craton; the majority of the samples portray a trondhjemitic character, like the Ebolowa TTG, while three samples show a calc-alkaline character similar to that of the Sangmelima granodiorite. The ferromagnesian oxides content (Fe2O3Total + MgO + TiO2) varies from 6% to 12%. Mg#[Mg2+/(Mg2+ + FeTotal) × 100, with FeTotal as Fe2+] varies from 30 to 49, with a general decrease with increasing SiO2. Although K/Rb ratios (199–438) are higher that those reported by [10], similar values are known in many Archean cratons, among which are the Congo craton [46,47], the Antarctic craton [49], and the Sino-Korean craton [15]. The primordial mantle-normalized multi-element diagram [26] (Fig. 4a) shows a pattern similar to the Sangmelima TTG with a negative Ta anomaly. The U content (0–4.4 ppm) is higher than in the Sangmelima charnockites (mean 0.5 ppm) [47], and the Ebolowa potassic granitoids (mean 0.87 ppm) [55]. The [LaN/YbN] ratios are between 7 and 28 (cf. Table 1). The samples show slight negative to positive Eu anomalies (Eu/Eu* = 0.76 to 1.31).
Chemical data of the pyroxene–amphibole-bearing gneiss samples
Tableau 1 Données d’analyse chimique des échantillons du gneiss à pyroxène et amphibole
Samples | By1 | Go1 | Ka1 | Ka3a | Nyo-1 | Ng-1 | Bo-3 | Bo-1 |
SiO2 | 58.84 | 69.30 | 60.72 | 64.37 | 67.05 | 66.51 | 67.63 | 65.14 |
TiO2 | 0.84 | 0.46 | 0.65 | 0.71 | 0.83 | 0.65 | 0.74 | 0.55 |
Al2O3 | 16.66 | 15.04 | 16.08 | 15.95 | 13.73 | 15.40 | 13.64 | 15.81 |
Fe2O3 | 8.17 | 4.07 | 6.66 | 6.34 | 6.85 | 5.30 | 6.19 | 5.44 |
MnO | 0.13 | 0.04 | 0.11 | 0.12 | 0.09 | 0.07 | 0.09 | 0.08 |
MgO | 2.80 | 1.36 | 3.22 | 2.06 | 1.51 | 1.30 | 1.59 | 2.30 |
CaO | 5.80 | 2.68 | 6.11 | 5.29 | 4.00 | 4.97 | 3.47 | 4.27 |
Na2O | 5.43 | 3.58 | 4.20 | 4.17 | 3.43 | 4.16 | 3.22 | 4.05 |
K2O | 1.28 | 3.46 | 1.45 | 0.91 | 2.21 | 1.64 | 3.24 | 2.02 |
P2O5 | 0.19 | 0.19 | 0.20 | 0.19 | 0.26 | 0.21 | 0.26 | 0.19 |
LOI | 0.56 | 0.59 | 1.02 | 0.64 | 0.29 | 0.32 | 0.53 | 0.69 |
Sum | 100.93 | 101.15 | 100.56 | 100.96 | 100.49 | 100.73 | 100.84 | 100.74 |
Na2O + K2O | 6.71 | 7.04 | 5.64 | 5.08 | 5.65 | 5.80 | 6.46 | 6.06 |
Na2O/K2O | 4.25 | 1.03 | 2.90 | 4.58 | 1.55 | 2.54 | 1.00 | 2.01 |
A/CNK | 0.80 | 1.04 | 0.82 | 0.91 | 0.90 | 0.87 | 0.90 | 0.95 |
Mg# | 40 | 40 | 49 | 39 | 30 | 33 | 34 | 46 |
Ba | 748 | 2270 | 305 | 684 | 1377 | 897 | 1309 | 1079 |
Co | 22.6 | 9.2 | 55.9 | 35.4 | 16.1 | 13.3 | 13.4 | 14.3 |
Cr | 146 | 273 | 67 | 108 | 14 | 21 | 20 | 33 |
Ni | 86.2 | 121 | 56.6 | 61.4 | 1.6 | 9.9 | 5.8 | 18.6 |
Rb | 28.4 | 92.9 | 49.6 | 37.9 | 60.3 | 31.1 | 92.1 | 66.7 |
Sr | 659 | 495 | 389 | 619 | 242 | 513 | 249 | 389 |
V | 138.8 | 47.3 | 105.8 | 96.4 | 88.4 | 73.2 | 80 | 82.1 |
Y | 25.5 | 7.2 | 23 | 23.3 | 31.7 | 14.9 | 42.8 | 15.9 |
Zn | 77.4 | 37.3 | 46 | 46.7 | 60.5 | 31.8 | 56.8 | 51.4 |
Zr | 228 | 173 | 143 | 211 | 232 | 188 | 269 | 184 |
K | 10600 | 28746 | 11995 | 7554 | 18378 | 13614 | 26870 | 16743 |
La | 43 | 104 | 40 | 43 | 28 | 40 | 59 | 39 |
Ce | 51 | 199 | 55 | 46 | 60 | 49 | 129 | 68 |
Pr | 28.9 | 56.3 | 6.51 | 5.25 | 29.8 | 27.8 | 55.4 | 6.30 |
Nd | 5.3 | 11.1 | 30.1 | 24.7 | 6.2 | 4.9 | 7.7 | 25.4 |
Sm | 1.9 | 1.8 | 5.5 | 4 | 1.1 | 1.5 | 1.2 | 6.2 |
Eu | 2 | — | 1.3 | 1.7 | 2.7 | 1 | 3.6 | 1.3 |
Gd | 19.7 | 12.9 | 4.52 | 3.65 | 13.3 | 10.9 | 19.8 | 2.69 |
Tb | 8.6 | 39.3 | 0.66 | 0.55 | 4.6 | 10.9 | 14.9 | 0.36 |
Dy | 0.4 | 4.4 | 3.75 | 3.19 | — | 3.2 | — | 1.92 |
Ho | 19.2 | — | 0.70 | 0.62 | — | — | 18.3 | 0.34 |
Er | 373 | 309 | 2.01 | 1.75 | 305 | 438 | 292 | 0.91 |
Tm | 0.04 | 0.19 | 0.31 | 0.25 | 0.25 | 0.06 | 0.37 | 0.13 |
Yb | 21.5 | 8.93 | 2 | 1.8 | 7.09 | 3.41 | 11.08 | 0.72 |
Lu | 14.47 | — | 0.32 | 0.24 | 7.63 | 27.38 | 5.81 | 0.12 |
Hf | 25.8 | 68.75 | 3.57 | 4.62 | 34.4 | 3.93 | ||
Ta | 0.97 | 0.72 | 0.19 | |||||
W | 341 | 188 | 7.76 | |||||
Pb | 10.6 | 14.3 | 5 | |||||
Th | 2.3 | 5.1 | 3.6 | |||||
U | — | 0.4 | 2.8 | |||||
Nb | — | — | — | |||||
Be | 1.461 | 1.29 | 1.15 | |||||
Cs | 0.51 | 0.79 | 0.30 | |||||
Cu | 38.3 | 45.1 | 22.4 | |||||
Ga | 20.7 | 18.4 | 19.7 | |||||
Ge | 1.31 | 1.08 | 0.99 | |||||
Mo | — | 1.28 | — | |||||
Sn | 1.81 | 0.92 | 0.99 | |||||
K/Rb | 242 | 199 | 251 | |||||
Rb/Sr | 0.13 | 0.06 | 0.17 | |||||
Th/U | 13.62 | 12.75 | 1.29 | |||||
LaN/YbN | 0.77 | 16.27 | 22.02 | |||||
Eu/Eu* | 16.9 | 1.31 | 0.94 | |||||
Sr/Y | 26.5 | 24.5 |
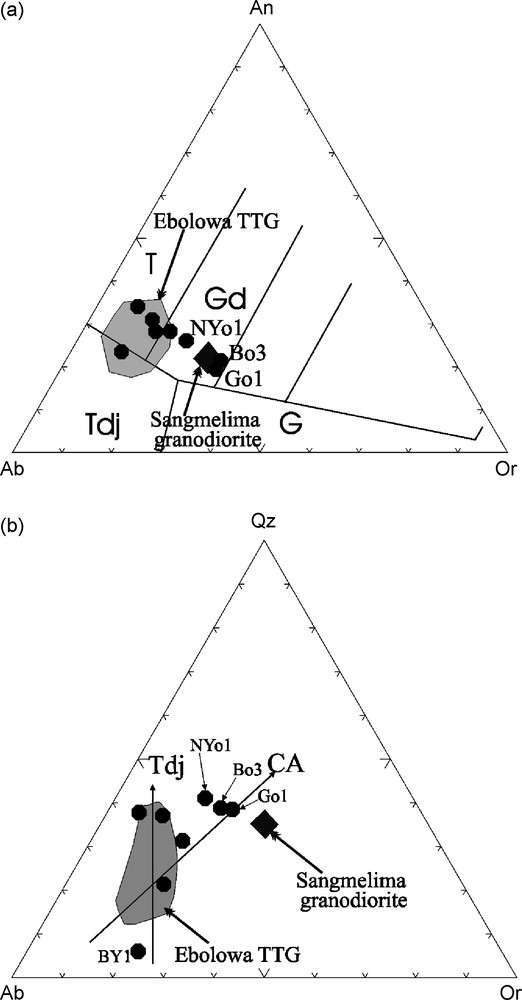
(a) Normative An–Ab–Or classification from O’Connor [39] of studied gneiss in comparison with Ebolowa TTGs [29] and Sangmelima granodiorite [27]. (b) Q–Ab–Or normative diagram of Barker and Arth [3] showing the trondhjemitic character of five samples and the calc-alkaline character of three samples (compared with Ebolowa TTG [29] and Sangmélima granodiorite [27]).
Fig. 3. (a) Classification des gneiss étudiés dans le diagramme An–Ab–Or de O’Connor [3], comparé aux TTG d’Ebolowa [29] et la granodiorite de Sangmelima [27] (zones grisé clair et grisé sombre. (b) Q–Ab–Or normatif d’après Barker et Arth [3], montrant le caractère trondhjémitique de cinq échantillons et calco-alcalin de trois échantillons (zone en grisé clair : TTG d’Ebolowa [29] ; zone en grisé sombre : granodiorite de Sangmelima [27]).
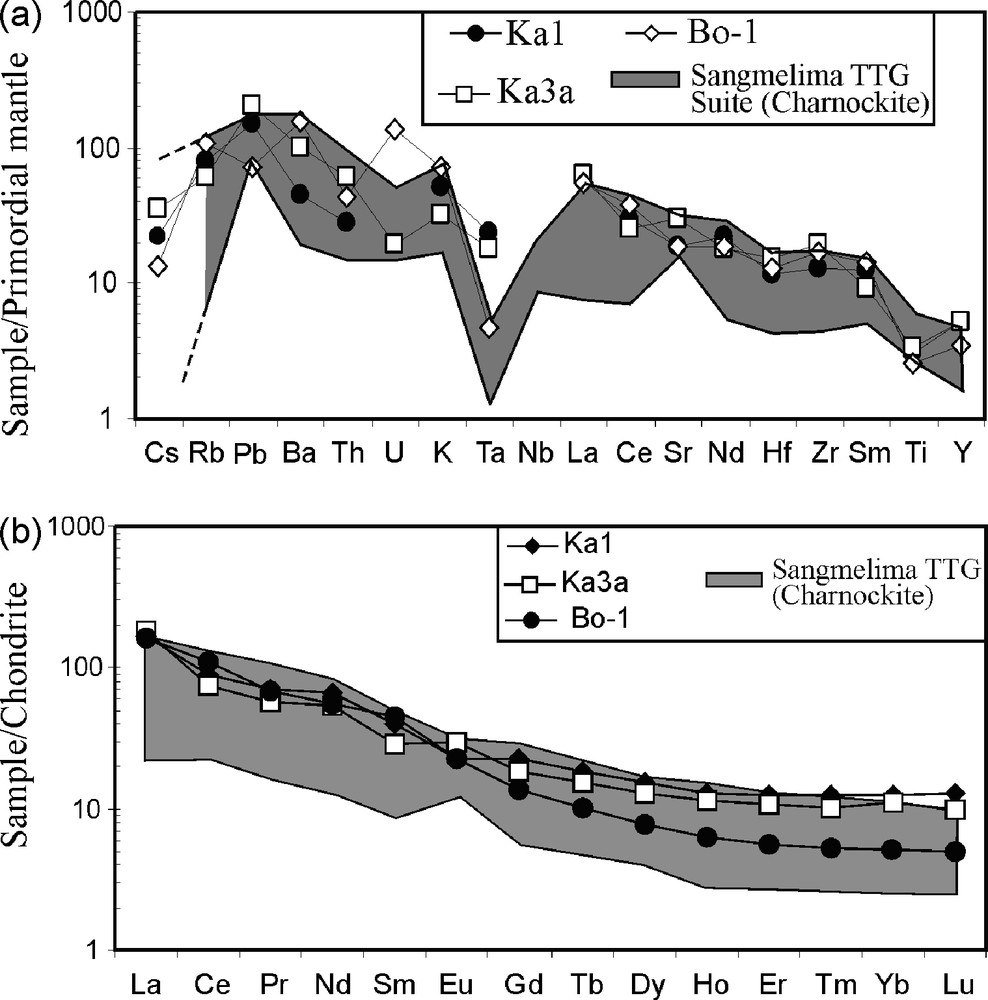
(a) Primordial mantle-normalized spidergrams of the studied gneiss in comparison with the Sangmelima TTGs [48]; normalisation values are from [26]. G = granite ; Gd = granodiorite ;Tdj = trondhjemite ; TTG = tonalite, trondhjemite, granodiorite. (b) Chondrite-normalized REE patterns (normalized values are from [25]), in comparison with the Sangmelima TTGs [48].
Fig. 4. (a) Diagramme multiélément normalisé au manteau primitif des gneiss étudiés, en comparaison avec les TTG de Sangmélima [48] ; les valeurs de normalisation sont issues de [26]. G = granite ; Gd = granodiorite ; Tdj = trondhjemite ; TTG = tonalite, trondhjémite, granodiorite. (b) Spectre des terres rares normalisées à la chondrite (les valeurs de normalisation sont issues de [25]), en comparaison avec les TTG de Sangmélima [48].
The main characteristic of Archean crust is an extremely low content in Y and heavy REE due to partial fusion of a source containing garnet as a residual phase. Accordingly, TTGs usually display Y and Yb concentrations less than 10 and 1 ppm, respectively, which is two to three times less than in normal calc-alkaline rocks. The chondrite-normalized REE patterns [25] (Fig. 4b) of representative samples do not reveal any significant slope from Dy to Lu for samples Ka3 and Ka1; accordingly, this feature permits to conclude that these samples do not belong to TTGs. In contrast, a significant slope from Dy to Lu and low Yb concentration (<1 ppm) are observed for sample Bo-1, showing that this sample is probably a TTG. The Sr/Y ratios for samples Nyo-1, By1 and Bo-3 are less than 30 (7.63, 25.8 and 5.81), the Yb and Y contents greater than 1 and 10 ppm, respectively; samples Go1 and Ng-1 portray Sr/Y ratios of more than 30 and Yb contents of 1 ppm or below. Nyo-1, By1 and Bo-3 cannot be regarded as TTGs, while Go1 and Ng-1 can be.
5 207Pb/206Pb geochronology by evaporation on single zircon crystals
The internal structure of zircon grains was examined in sample Bo-1, a pyroxene–amphibole-bearing gneiss. Fig. 5 shows pictures of representative grains. The BSE (backscattered electron) images of the polished surfaces (indicated with letter a) are light grey (a1) to grey (a4). The zircon grains are fractured, indicating that they have been affected by brittle deformation. They are euhedral (a2) to subhedral (a4) in shape. Zircon grains b1 and b4 show highly luminescent cores without oscillatory zonation, surrounded by less luminescent and slightly zoned rims. The contact between the two parts is irregular and the external part tends to extend into the core. Zircon b2 is free of a core and shows euhedral oscillatory zoning. Zircon b3 has a well-developed euhedral, oscillatory-zoned core, surrounded by a less luminescent rim. Zircon b5, in contrast, shows a dark core with a rounded shape, and a less luminescent and less expressed oscillatorily zoned rim. Dark cores in zircon were described in high-grade gneiss terranes and reveal high content in U [40]. In the rims of the zircons b4 and b5, the zonation seems to blot out; this can be related to the differential absorption of contaminants during crystallisation [61] or to the influence of deformation [45]. The euhedral oscillatory zonation of the studied zircons is indicative of their magmatic origin.
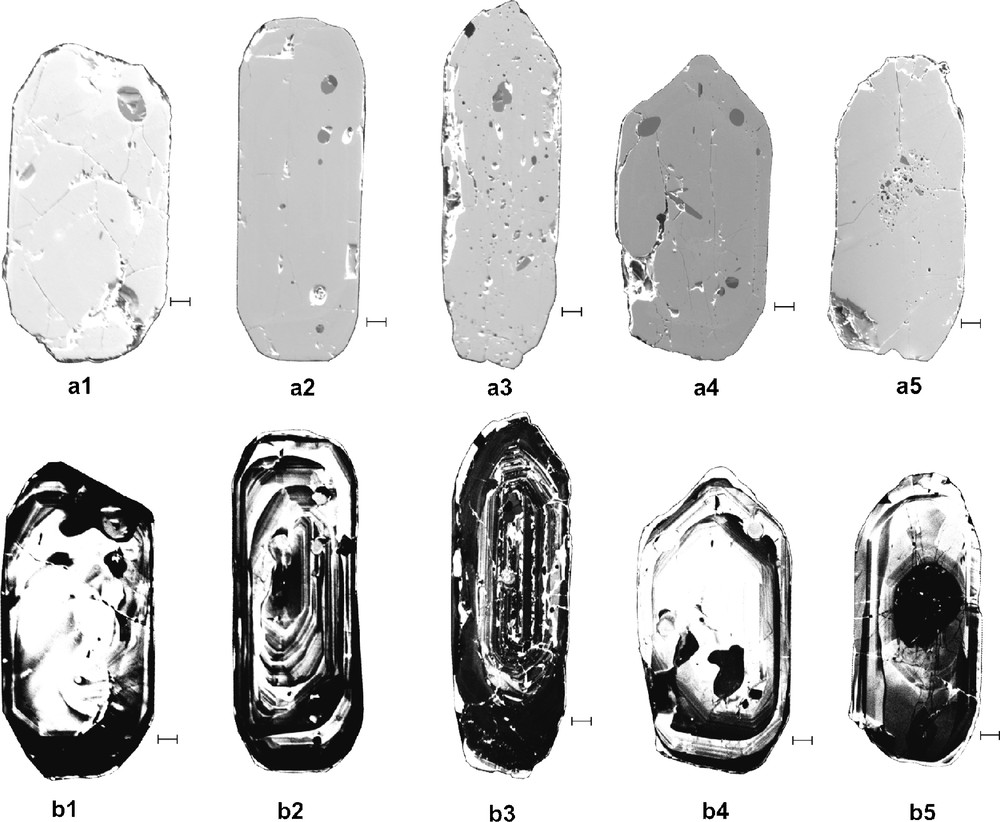
BSE images (a) and cathodoluminescence images (b) of representative zircon crystals of the studied gneiss (sample Bo-1).
Fig. 5. Images BSE (a) et cathodoluminescence (b) de quelques zircons représentatifs du gneiss étudié (échantillon Bo-1).
The results of evaporation of five representative zircon grains of sample Bo-1 are presented in Table 2. The Th/U ratios were calculated using the measured 208Pb/206Pb ratios and the apparent 207Pb/206Pb dates. The Th/U ratios are generally high and vary from one heating step to another of the same zircon grain, and from one grain to another. This variation is due to the magmatic growth of the zircon, and reflects the magma evolution during zircon growth [17,18]. The Th/U ratios, which vary from 1.3 to 2.7, display no correlation with the heating temperature; they can be compared with the range of values yielded by zircons from the Sangmelima granodiorite [48]. The Th/U ratios could be influenced by Th-rich inclusions; therefore, the inclusions revealed by the polished section of zircons (cf. Fig. 5) may be Th-rich minerals such as thorite (ThSiO4) or thorianite [(Th,U)O2]. The 204Pb/206Pb ratios are less than 0.0001 in seven steps, and 0.001 in one step. The 207Pb/206Pb ratios vary from 0.104847 ± 094 to 0.174236 ± 195, the corresponding ages range from ∼1.7 Ga (Palaeoproterozoic) to ∼2.6 Ga (Late Archean) (Fig. 6). There is no good age reproducibility either between different heating steps of the same grain or between different grains. This is probably due to the presence of cores in many zircons of the studied sample.
Zircon evaporation data including radiogenic 207Pb/206Pb ratios and corresponding ages for the studied gneiss (sample Bo-1)
Tableau 2 Données d’évaporation du zircon avec les rapports 207Pb/206Pb radiogénique et les âges correspondants du gneiss étudié (échantillon Bo-1)
Sample and zircon number (a,b,c = temp. step | Evap. Temp °C | No. of ratio | U/Th ratio | 206Pb/208Pb ratio | 204Pb/206Pb ratio | 207Pb/206Pb isotope ratio | 207Pb/206Pb age (Ma) 2σ error |
1–1a | 1400 | 88 | 1.46 | 4.89 | 0.000065 | 0.110869 ± 0.000100 | 1813.7 ± 3.4 |
1–1b | 1430 | 96 | 1.87 | 6.24 | 0.000069 | 0.104847 ± 0.000094 | 1711.7 ± 3.4 |
1–1c | 1460 | 70 | 1.97 | 6.58 | 0.000067 | 0.103344 ± 0.000147 | 1685.0 ± 4.0 |
1–2a | 1410 | 114 | 2.00 | 7.21 | 0.000239 | 0.159781 ± 0.000284 | 2453.4 ± 4.3 |
1–2b | 1440 | 114 | 1.87 | 6.71 | 0.000239 | 0.165466 ± 0.000164 | 2512.3 ± 3.4 |
1–2c | 1470 | 114 | 1.64 | 5.84 | 0.000239 | 0.162137 ± 0.000144 | 2478.2 ± 3.3 |
1–3a | 1380 | 114 | 2.45 | 8.36 | 0.000019 | 0.157660 ± 0.000145 | 2430.7 ± 3.4 |
1–3b | 1410 | 36 | 2.10 | 7.17 | 0.000014 | 0.164673 ± 0.000209 | 2504.3 ± 3.7 |
1–3c | 1435 | 152 | 1.41 | 4.84 | 0.000010 | 0.174236 ± 0.000195 | 2602.6 ± 13.5 |
1–4a | 1380 | 114 | 1.28 | 4.34 | 0.000337 | 0.135458 ± 0.000195 | 2170.0 ± 4.0 |
1–4b | 1400 | 113 | 2.70 | 9.17 | 0.000153 | 0.143879 ± 0.000117 | 2274.5 ± 3.3 |
1–4c | 1420 | 70 | 2.51 | 8.55 | 0.000103 | 0.153723 ± 0.000314 | 2387.8 ± 4.6 |
1–5a | 1380 | 111 | 1.44 | 5.11 | 0.000253 | 0.154660 ± 0.000251 | 2398.2 ± 4.1 |
1–5b | 1400 | 106 | 1.65 | 5.67 | 0.000051 | 0.165629 ± 0.000177 | 2514.0 ± 3.5 |
1–5c | 1430 | 112 | 1.70 | 6.00 | 0.000165 | 0.172099 ± 0.000279 | 2578.2 ± 4.0 |
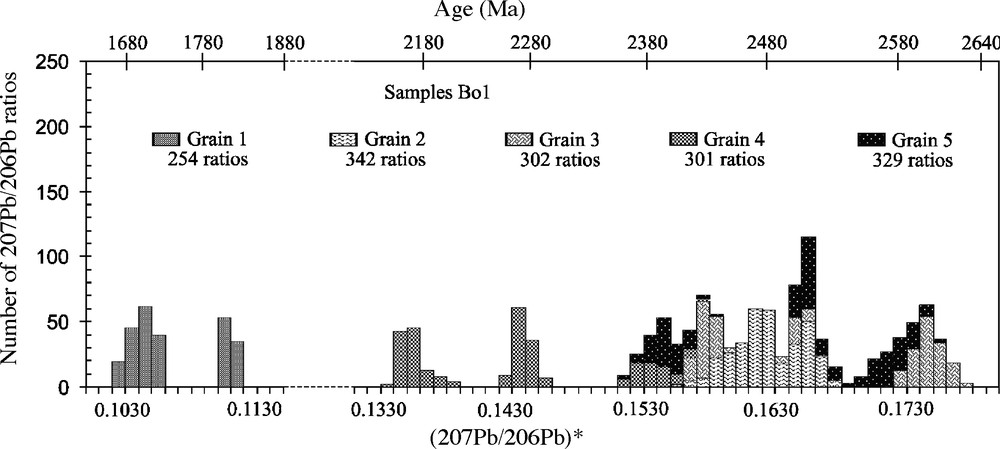
Histogram showing the distribution of radiogenic 207Pb/206Pb ratios obtained from evaporation of zircons from pyroxene-amphibole gneiss (sample Bo-1).
Fig. 6. Histogramme montrant la distribution des rapports 207Pb/206Pb obtenus par évaporation des zircons du gneiss à pyroxène et amphibole (échantillon Bo-1).
6 Discussion and conclusion
The Méiganga area is underlain by granitic and metamorphic rocks comprising pyroxene–amphibole-bearing orthogneisses. These are mesocratic to leucocratic rocks with medium- to high-potassic calc-alkaline character. Their normative composition and the decrease of the Mg# relative to the increase of SiO2 (Fig. 7) show affinities with many TTGs: Sangmelima charnockites [47], Ebolowa granitoids [55] from the Congo craton, and other <3.0-Ga-old TTGs [51]. They exhibit negative anomalies in Ta and Ti, and are enriched with LREE with respect to the HREE (cf. Fig. 4a and b).
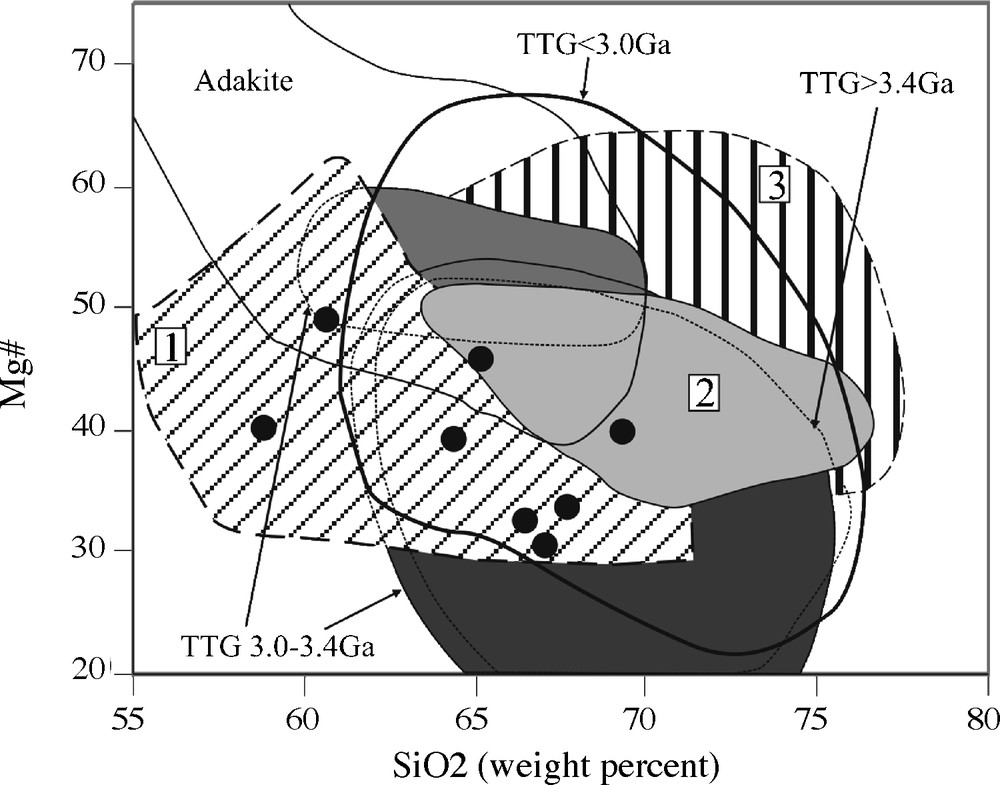
SiO2 versus Mg# (Mg number) diagram of studied gneisses in comparison with the Sangmelima TTG (1: charnockitic suite; 2: tonalitic suite; 3: granodiorite [48]) and other TTGs [51].
Fig. 7. Diagramme SiO2 en fonction de Mg# (Mg number) des gneiss étudiés, en comparaison avec les TTG de Sangmelima (1 : suite charnockitique ; 2 : suite tonalitique ; 3 : granodiorite [48]) et les champs de TTG de Smithies [51].
The zircons of the gneisses are euhedral, with oscillatory zonation. They show U-rich cores (e.g., [40]) and have been influenced by subsequent deformation [45]. The 207Pb/206Pb ages around 2 Ga (samples Bo1–1 and Bo1–4, Table 2) are probably close to the magmatic crystallisation age, concerning the magmatic feature of the zircons; thus they testify to the production of juvenile crust material during the Eburnean orogeny. The zircon ages between 2.5 and 2.6 Ga from assumed TTG reflect Latest Archean granite formation, which is a worldwide feature, or mixing ages. Anyhow, the 2.6-Ga age represents a minimum one for the inherited zircon cores of the studied sample.
The study area belongs to the West Central African Belt (WCAB), which resulted from the Eburnean collision between the Congo and São Francisco Cratons, and which is present as septa in the Late Neoproterozoic Panafrican Belt [41]. The main metamorphic event in the WCAB was dated at about 2050 Ma in the external zone (including the Nyong series) and 2100 Ma in the internal zone, the latter comprising the study area [41]. In the external zone, the metamorphic event was accompanied by emplacement of granodiorites (2066 Ma) and syenites (2055 Ma) [24]. We propose that the emplacement of the intrusive protolith of the pyroxene–amphibole-bearing gneiss occurred prior to the metamorphic event around 2.1 Ga, which then superimposed the gneissic structure to the rock.
The presence or influence of the Archean crust has been put into evidence in several regions in Nigeria [11]. The Kabba-Okenne granodiorite (widely occurring orthogneiss unit in the basement complex of Nigeria) gives a precise U–Pb zircon age of 2.1 Ga, which is interpreted as the crystallization age [2], but the Nd model age of 2.5 Ga in this granodiorite suggests that some Archean crustal component was incorporated when the Lower Proterozoic crust was formed [11]. Zircon Pb–Pb ages from an orthogneiss complex of the Caruaru area, northern Brazil, yielded crystallisation ages of 2.098 to 2.075 Ga [30]. Zircon U–Pb ages from orthogneisses north of the Pato shear zone, NE Brazil, reflect the 2.2–2.0-Ga Palaeoproterozoic crust, with local preservation of an Archean domain [60]. The Sm–Nd ages of 2.0 to 3.0 Ga in the Sertânia metasedimentary complex in the Alto Moxotó belt portray its provenance from Palaeoproterozoic and Archean sources [6].
The geochemical affinity of the studied gneisses with the Archean rocks (i.e. Sr/Y ratio, Y and REE contents) shows the inheritance of an older crustal signature during the emplacement of the protolith of the pyroxene–amphibole-bearing gneiss. The 207Pb/206Pb evaporation age around 2.0 Ga might be close to the crystallisation age, whilst the oldest age (2.6 Ga) probably represents a minimum age for the inherited zircon cores. Such cores could represent xenocrysts older than the gneiss protolith, which were incorporated into the melt during formation or emplacement. The zircon cores regularly show a round shape that might be due to their detrital origin. Such grains cannot be formed by magmatic precipitation in the original gneiss protolith. The detrital nature of the zircon cores provides evidence of the presence of older formations prior to the emplacement of the magmatic protolith of the pyroxene–amphibole-bearing gneiss.
The Archean zircon age (2.6 Ga) is the first Archean age obtained by the 207Pb/206Pb evaporation method in the Palaeoproterozoic septa of the Panafrican fold belt to the north of the Congo Craton in Cameroon. Archean inheritances had been demonstrated in the Panafrican fold belt by Nd model age or by upper intercept of U–Pb discordia ages. An amphibolite interlayered in the studied gneiss gave an Nd model age of 2.7 Ga [13]. In the Kombe area, in the southern part of the Panafrican fold belt in Cameroon, gneisses are characterized by Archean Nd model ages between 2.7 Ga and 3.5 Ga [12]. Archean ages (2.9 Ga) were also deduced from upper discordia intercepts (U–Pb) in many parts of the Panafrican fold belt [58], e. g., the Makenene, Kekem, and Kalaldi-Toldoro areas.
In the Nyong series, ages of 2.5 Ga to 2.6 Ga were obtained from pre orogenic sediments that had been submitted to the 2050 thermotectonic event [24]. The 2.4–2.6-Ga-old zircon cores in the studied gneiss probably derived from (meta)sediments deposited during the Palaeoproterozoic. This deposit was metamorphosed at ca. 2050 Ma during the Eburnean orogeny, which might have involved the entire West Central African Belt (WCAB). The studied gneiss belongs to an Eburnean–Transamazonian domain, which covers Central Africa and extends to northeastern Brazil.
Acknowledgements
The authors acknowledge Dr Hartmut Schulz for the CL images. The first author (AAG) is highly indebted to the German Academic Exchange Service (DAAD) for support of his research stay in the University of Tubingen. Frédéric Villeras of LEM (Nancy, France) is acknowledged for the REE analyses. Thanks go to two anonymous reviewers for their critical reviews of the manuscript.