1 Introduction
Among the many facets of the so-called “dolomite problem” (see Machel, 2004, for a discussion and references), hydrothermal dolomite has been recently the object of interest and debate (Davies and Smith, 2006; Machel and Lonnee, 2002). In order for dolomite to be interpreted as hydrothermal, not only a relatively high temperature of formation is to be proved, but it should be coupled to inferred burial depths that cannot justify the calculated temperatures, insofar suggesting the upward advection of hot dolomitizing fluids (Machel, 2004).
In the stratigraphic successions of the Maritime Alps at the south-eastern termination of the Argentera Massif, a dolomitization of the Jurassic limestones was reported by those authors who dealt with these areas in the past decades (Campanino Sturani, 1967; Carraro et al., 1970; Malaroda, 1970), but neither detailed description nor attempts of interpretation were provided. Stratigraphic constraints, geometry and petrography of the dolomitized bodies, and isotope data and fluid inclusion microthermometry allow to reconstruct age, temperatures and burial depth for the dolomite of the Jurassic succession of the study area.
The purpose of this article, therefore, is to contribute to refine the understanding of the dolomitization process, by means of a case history, and, from a regional point of view, to evaluate the implications of the occurrence of this kind of dolomite on the tectono-sedimentary evolution of this part of the passive European Tethyan margin.
2 Geographic and geological setting
The study area is located in the north-eastern Maritime Alps (North-Western Italy), between the Sabbione Valley to the west and the upper Vermenagna Valley to the east, close to the village of Palanfrè (Fig. 1). This sector is composed of several tectonic units, presently superimposed along low-angle NW-SE striking Alpine tectonic contacts. Their geometrical position has been classically interpreted to reflect the paleogeographic position along the Mesozoic European paleomargin of the western Tethys, thus the lowest units have been classically attributed to the more external Dauphinois Domain, and the overlying units to the more internal Subbriançonnais Domain (Carraro et al., 1970).
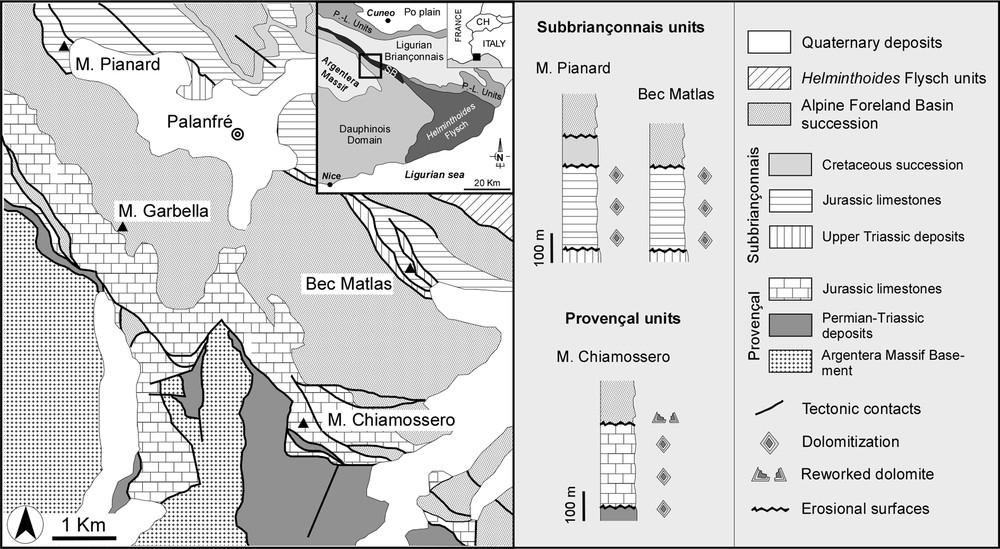
Geological sketch map of the study area, with three simplified stratigraphic logs of the Subbriançonnais and Provençal Units (SB: Subbriançonnais; P.-L. Units: Piemonte-Liguria Units).
Schéma géologique du secteur étudié, avec trois successions stratigraphiques simplifiées des Unités Subbriançonnaise et Provençale (SB : Subbriançonnais ; P.-L. Units : Unités Liguro-Piémontaises).
More precisely, in the study area the main features of the Dauphinois succession are closely comparable to the Provençal ones, that are characterized by a reduced thickness and shallow water facies. In the following, we will refer to this succession as Provençal. It starts with Permian continental sediments that rest on the crystalline basement of the Argentera Massif and are characterized by marked thickness changes; they are followed by some tens of meters of Lower Triassic coastal siliciclastic deposits and Middle Triassic peritidal dolomitic limestones. A regional discontinuity surface corresponding to a Late Triassic-Middle Jurassic hiatus is followed by a thick (200-300 m) succession of Middle?-Upper Jurassic platform limestones. Locally, at the top of the Jurassic carbonates a few meters of Lower Cretaceous calcareous-marly deposits are preserved (Carraro et al., 1970).
The basement of the Subbriançonnais succession is unknown as it is detached in correspondence of Upper Triassic shales. Above, a thick carbonate succession (200–300 m) of uncertain age (Early-Late Jurassic) is present, and shows the same features as the coeval Provençal interval. The Cretaceous succession is characterized by important lateral variations: it is absent in the south-eastern sector (Bec Matlas, Fig. 1), while it reaches thicknesses of one hundred meters in the north-western sector (M. Pianard, Fig. 1). Here, it is formed by bioclastic limestones, probably of Early Cretaceous age, and by Upper Cretaceous marly limestones and sandy limestones (Zappi, 1960).
In both Provençal and Subbriançonnais units, the top of the Mesozoic succession is truncated by a regional unconformity corresponing to a hiatus spanning the Late Cretaceous-Middle Eocene. Above the unconformity, the Alpine Foreland Basin succession consists of the Middle Eocene Nummulitic Limestone, followed by the hemipelagic Upper Eocene Globigerina Marl and by the Upper Eocene-Lower Oligocene turbidite succession of the Grès d’Annot (Sinclair, 1997).
The tectonic history of the above-described succession was marked in Mesozoic times by syn-sedimentary extensional and strike-slip tectonics (Bertok et al., 2011, 2012). Since Late Eocene-Early Oligocene the paleo-European continental margin was then progressively involved in the on-going formation of the Alpine belt. All the study successions underwent at least three deformation events well recorded at regional scale, firstly with outward (south-west) brittle-ductile thrusting and superposed foldings, north-east back-vergent folding and then with S-ward brittle thrusting and flexural folding. The overall regional kinematic was displayed in a transpressional regime with important strain partitioning of contractional vs. strike-slip related structural associations (Molli et al., 2010; Piana et al., 2009), as evidenced by the occurrence of a post-Oligocene NW-SE Alpine transcurrent shear zone (Limone Viozene Zone, LIVZ) extending for several kilometres from Tanaro valley to the study area. The LIVZ dies out north-west-ward some kilometers south of Gesso Valley where it merges with the east-west strike-slip shear zone system known as “Stura Fault” of Ricou and Siddans (1986). Despite the high amount of finite deformation in the study area, strain partitioning allowed preservation of most of the primary stratigraphic features and geometrical relationships.
The occurrence of dolomitization affecting the Jurassic limestones of both Provençal and Subbriançonnais units in the study area was already reported, although very briefly, by previous authors (Campanino Sturani, 1967; Carraro et al., 1970; Malaroda, 1970). However, no description of the stratigraphic and petrographic features of the dolomitized sediments was given and no explanation was proposed regarding their genetic processes.
3 Methods
Field work and geological mapping were performed in order to reconstruct the geometry, extension and distribution of the dolomitized bodies. Petrographic studies were carried out on selected samples by optical microscopy and cathodoluminescence aimed to distinguish different dolomite generations. Fourteen microdrilled samples were measured for their carbon and oxygen isotope composition following the method after McCrea (1950) in which carbonate powder is reacted in vacuum conditions with 99% orthophosphoric acid at 25 °C (time of reaction: 4 h for calcite and 6–7 h for dolomite) using a Finnigan MAT 252 mass spectrometer (MARUM Stable Isotope Laboratory, Bremen, Germany). The isotopic ratios are expressed as δ13C and δ18O per mil values relative to the Vienna Pee Dee Belemnite (VPDB) standard (precision ± 0.05‰). Petrography and microthermometry of primary fluid inclusion assemblages on saddle dolomite were performed, using a Linkam THMSG600 heating–freezing stage coupled with an Olympus polarizing microscope (100× objective) at the Department of Earth Sciences, University of Torino (Italy), in order to record the homogenization temperatures.
4 Host rocks
The studied dolomite occurs mainly within the Jurassic limestones of both Provençal and Subbriançonnais units; locally, however, it also affects tectonic slices of Middle Triassic peritidal carbonates present in the LIVZ. The Jurassic limestones are organized in ill-defined dm- to m-thick beds and mainly consist of mudstones to packstones with echinoderm fragments; the upper portion is made up of bioclastic limestones, ranging from packstones to rudstones and boundstones, rich in corals, nerineid gastropods, thick-shelled bivalves, and stromatoporoids. The stratigraphically highest dolomitized beds are locally represented by lagoonal charophyte-rich wackestones. The lower portion of the dolomitized succession was attributed to the Middle-Upper Jurassic (Carraro et al., 1970), whereas the upper part was dated to the Kimmeridgian-Tithonian by Campanino Sturani (1967). However, lagoonal charophyte-rich beds analogous to those observed in the study area, are reported in the same stratigraphic position in the Provençal successions of the Nice Arc, and are dated to the Middle-Late Berriasian (Dardeau and Pascal, 1982). At present, the study of the charophyte associations is in progress, in order to verify the possible Berriasian age of the top of the dolomitized limestones.
5 Dolomite features
Dolomitization shows a great variability in the study area. Some Jurassic limestones are only partly dolomitized with development of scattered crystals with euhedral habits (planar-e fabric of Sibley and Gregg, 1987) (Plate 1A). In other cases dolomitization is more intense and follows a more or less complex network of randomly oriented veins (100 μm- to 2 mm-thick on average) (Plate 1A, B). Veins locally are more closely spaced and arranged along subvertical, cm- to dm-wide, zones. No significant geometric relations with the main macroscale Alpine tectonic features (such as faults, master joints or hinge zone of flexural folds) have been observed. Dolomite veins crosscut the bedding and are displaced by younger calcite vein system of tectonic origin. Cm-wide, subvertical tabular bodies of dolomite-cemented breccias with partially dolomitized limestone angular clasts also occur (Plate 1B). Mm- to cm-sized irregularly shaped cavities are geopetally filled up with a basal fine-grained dolomite sediment and by coarse dolomite and calcite cements (Plate 1C) and are interpreted as the result of localized dissolution of the enclosing limestone. Fully dolomitized rocks, with a complete obliteration of primary fabrics, occur as small (dm- to m-wide) masses randomly distributed within the partially dolomitized limestones and show a variety of structures documenting complex transformations. Three most representative types can be recognized:
- • Type 1: quite homogeneous finely to medium-crystalline dolostones, resulting from pervasive replacement of the Jurassic limestones;
- • Type 2: clast-supported breccias made up of clasts of type 1 dolostones (Plate 1D). Clasts range from centimetric to decimetric in size, subrounded to angular in shape. Locally, angular clasts show a jigsaw puzzle arrangement. The voids between the clasts are filled up with mm-thick rims of a coarsely and very coarsely-crystalline (up to a few millimetres) whitish dolomite cement, followed by a dark sparry calcite cement plugging the remaining pores;
- • Type 3: clast-supported breccias, entirely composed of mm- to cm-long and 100 μm- to 2 mm-wide, plate-like, clasts of medium to coarsely-crystalline dolomite, strongly resembling the veins crosscutting the host limestones. The voids between the clasts are filled up with a dark sparry calcite cement.
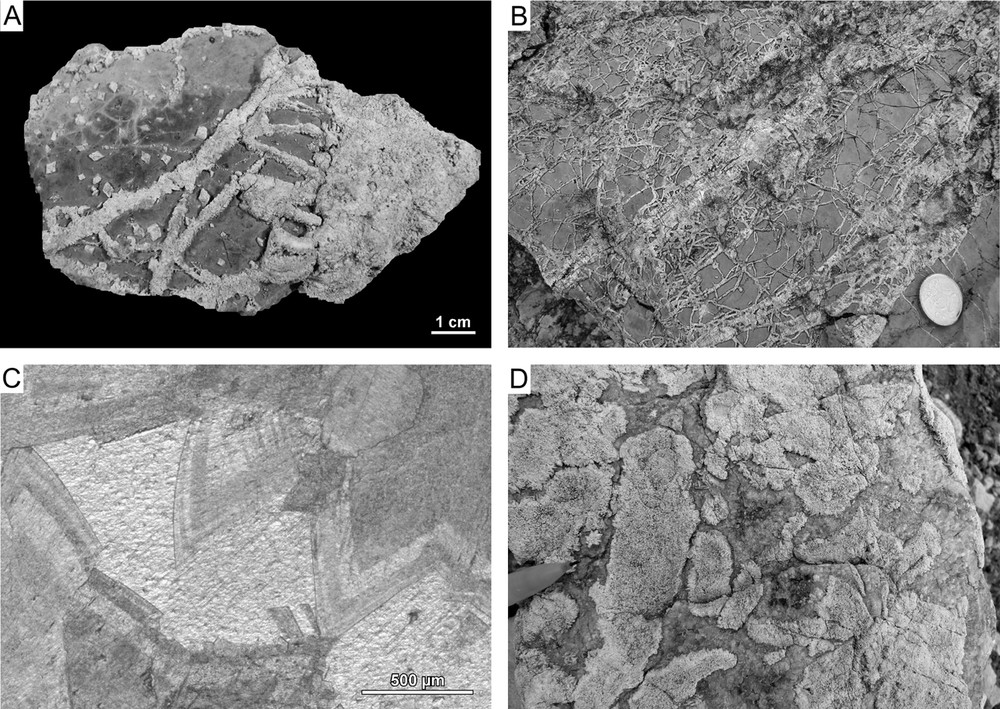
A. Hand specimen of the Jurassic limestones showing different degrees of dolomitization. The left portion is only partly dolomitized with euhedral scattered crystals of dolomite; the right portion is fully dolomitized, whereas in the middle portion dolomitization follows a network of veins. B. Upper portion of the partially dolomitized Jurassic limestones: note the juxtaposition of cm-wide bands of rock with randomly oriented dolomite-cemented veins and of subvertical tabular bodies of dolomite-cemented breccias. C. Photomicrograph of a cavity filled up with a rim of saddle dolomite and a sparry calcite cement. Note the curved crystal faces and the well-defined zonation of the saddle dolomite coarse crystals. D. Clast-supported breccia with fully dolomitized clasts coated by a mm-thick rim of whitish dolomite cement. Remaining voids are plugged by a sparry, grey to black, calcite cement. Pencil tip for scale, on the left, is 1.5 cm long.
A. Échantillon de calcaire jurassique montrant différents degrés de dolomitisation. La partie gauche est seulement partiellement dolomitisée, avec des cristaux euhédraux dispersés de dolomite ; la partie droite est entièrement dolomitisée, tandis que, dans la partie centrale, la dolomitisation suit un réseau de veines. B. Partie supérieure des calcaires jurassiques partiellement dolomitisés: noter la juxtaposition, à l’échelle centimétrique, de bandes de roche avec réseaux de veines dolomitiques diversement orientées et de corps tabulaires de brèches à ciment dolomitique. C. Vue en lame mince d’une cavité bordée d’un ciment de dolomite en selle et remplie d’un ciment calcitique. Noter les faces courbes des cristaux de dolomite en selle et leur zonage. D. Brèche à support clastique avec clastes entièrement dolomitisés et revêtus d’une frange millimétrique de ciment dolomitique blanchâtre. Les vides résiduels sont remplis d’un ciment de calcite sparitique, de couleur grise à noire. La pointe de crayon sur la gauche mesure 1,5 cm de longueur.
Dolomitization, both partial and complete, crosscuts the host limestone bedding. It affects discrete rock masses that are randomly distributed, and is not constrained to specific stratigraphic intervals.
From a microscopic point of view, two dolomite types may be distinguished. One is finely to medium-crystalline, non planar to planar-s (Sibley and Gregg, 1987), inclusion-rich, and occurs only as replacive dolomite. It shows a moderate, purple-red cathodoluminescence. The second type is coarsely- to very coarsely-crystalline, and shows the typical features of saddle dolomite (curved crystal faces, sweeping extinction) (Plate 1C). The alternation of more and less inclusion-rich bands outlines different growth stages. A well-defined zonation is also recognizable in cathodoluminescence: a moderate purple-red zone is followed by a non-luminescent zone with thin red hairlines, in turn overlain by a second moderate purple-red zone. It occurs both as replacive and void-filling dolomite, the latter giving rise to mm-thick rims fringing dissolution cavity walls and breccia clasts.
O isotopes of both dolomite types show values (δ18O ranging from –4 to –6‰ VPDB: Fig. 2) clearly more negative than seawater for dolomitizing fluids. More indicative data come from microthermometric analyses of a selection of primary fluid inclusions on saddle dolomite. Although this study is still in progress and it has not been conducted systematically on all the samples, more than 30 homogenization temperatures measured on a selection of four samples, show a relatively tight distribution, ranging from 170 to 220 °C, with the highest frequency around 200 °C. The determination of the fluid salinity has not been possible so far because of the small diameter of the fluid inclusions (few micrometers), that make difficult the observation of the complex phase transitions during freezing and melting of the fluid.
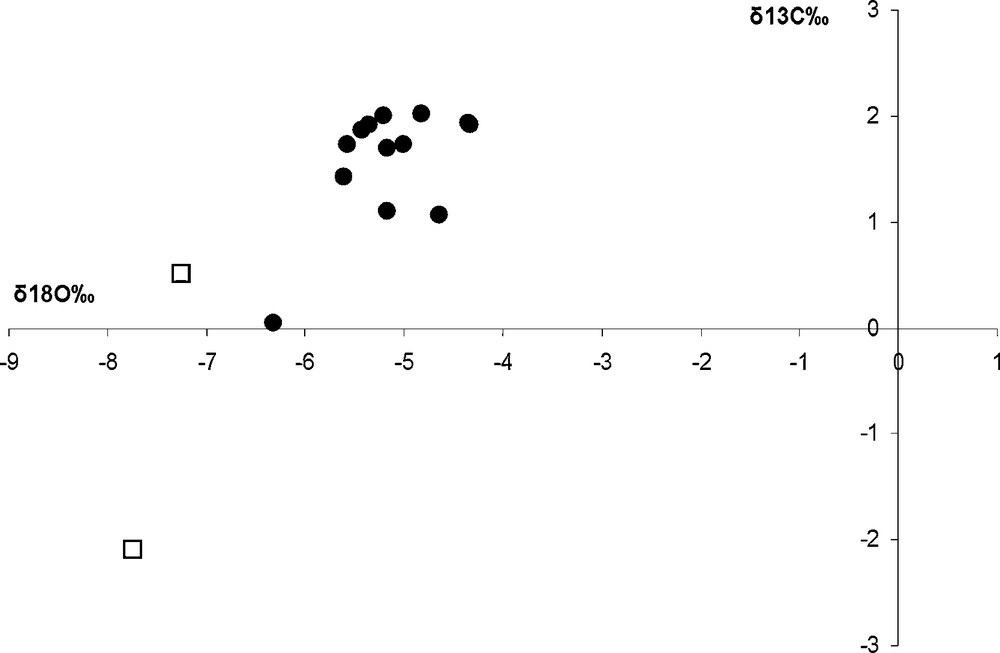
δ18O versus δ13C crossplot of pore-filling calcite (empty squares) and saddle dolomite (black dots). As a term of comparison, dolomites formed at surface in marine environments display isotopic values ranging approximately from +1 to +3 ‰ VPDB for both δ18O and δ13C (Tucker and Wright, 1990).
Graphique combiné avec les rapports δ18O et δ13C, mesurés sur la calcite (carrés vides) et la dolomite en selle (points noirs) de remplissage des pores. Pour comparaison, la dolomite formée à la surface dans des environnements marines montre des valeurs isotopiques compris entre +1 to +3 ‰ VPDB environ pour δ18O et δ13C (Tucker et Wright, 1990).
6 Reworked dolomite
The base of the Middle Eocene Nummulitic Limestone, resting on the regional unconformity, is locally represented by a m-thick bed of clast-supported conglomerate with dm-sized clasts of limestones and coarsely-crystalline dolomites. The conglomerate is followed by a several m-thick succession of dm-thick normally graded beds, made up of conglomerates to arenites; clasts and grains consist of dolomitic rocks and dolomite crystal fragments (Fig. 3). Clasts commonly show bivalve borings. Petrographic features of clasts and grains clearly document that they represent fragments of the underlying dolomitized limestones.
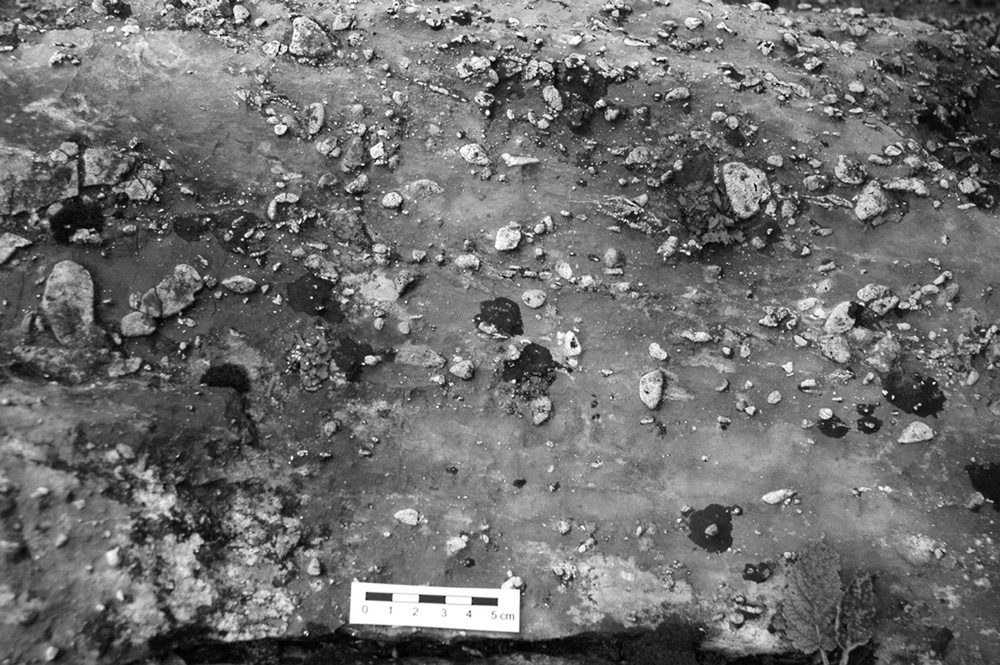
Conglomerate bed in the lower part of the Nummulitic Limestone, with mm- to cm-sized clasts of dolomitized rocks.
Niveau conglomératique dans la partie inférieure des Calcaires nummulitiques, contenant des galets dolomitisés millimétriques à centimétriques.
The occurrence of dolomitic clasts in the lower portion of the Nummulitic Limestone was already reported by Campredon (1977) who, however, did not recognize their provenance from the dolomitized Jurassic succession.
7 Discussion and conclusions
7.1 Age of dolomitization
Although no direct dating is available, timing of dolomite formation is well constrained by undisputable stratigraphic evidence. The age of the youngest dolomitized sediments documents that dolomitization cannot be older than Kimmeridgian-Tithonian (Campanino Sturani, 1967) or even Berriasian on the basis of the newly recognized charophyte-rich beds.
The occurrence of clasts and grains of the dolomitized limestones within the basal levels of the Nummulitic Limestone, conversely, states that dolomitization cannot be younger than the Bartonian as indicated by the Nummulites association usually found in the Nummulitic Limestone (Campredon, 1977; Varrone and Decrouez, 2007). More precise data come from the observation of Cretaceous sediments of the Provençal Domain in the study area. They are generally referred to the lower Lower Cretaceous (Neocomian: Carraro et al., 1970) and, in none of the limited and scattered outcrops available in the study area, are dolomitized. This allows to further restrict the dolomitization event within the Early Cretaceous.
7.2 Dolomitization process
The geometric (partially dolomitized bodies and dolomite-filled veins crosscutting host limestone bedding), petrographic (saddle dolomite, indicating temperatures higher than about 60 °C; Radke and Mathis, 1980), and geochemical (δ18O systematically lower than –4‰ VPDB) features of the dolomite, together with preliminary data from primary fluid inclusions (homogenization temperature values around 200 °C), attest that dolomitization took place after the deposition of the whole package of Jurassic limestones, and that dolomitizing fluids were hot and flowed mainly through fractures and veins. Dolomite precipitated both along such fracture systems and replaced, partially or completely, non-fractured volumes of carbonate sediments (type 1 dolostone). This clearly shows that at least part of the host Jurassic limestones were still permeable enough to allow a diffuse flux of dolomitizing fluids. This could suggest that dolomitization took place in an early stage of diagenesis, once again pointing to the Early Cretaceous. In this frame, type 2 dolomite breccias resulted from disruption of fully dolomitized limestones, due to the upward flux of overpressurized fluids whereas type 3 dolomite breccias document a thorough dissolution of a veined host limestone and the consequent collapse of the isolated dolomite vein fills.
In order to reconstruct the diagenetic environment of such dolomitization, the burial history of the Jurassic limestones must be taken into account. The occurrence of reworked dolomite clasts, commonly bored, within the basal Nummulitic Limestone interval clearly documents that in the Bartonian the Jurassic limestones, already dolomitized, were exposed on rocky coasts where they could be colonized by endolithic organisms and eroded. Cretaceous to Lutetian sediments are missing or very thin all over the study area. Moreover, they are completely missing as clasts in basal Eocene conglomerates, and the underlying unconformity is never associated to evident angular geometrical relationships. All these lines of evidence lead to conclude that if a Cretaceous-Lutetian sediment package had been deposited on top of the Jurassic limestones, it was surely thin enough as to be removed without leaving any trace before the Bartonian transgression. Further to the north-west, at the north-western edge of the Argentera Massif, a Turonian unconformity, associated with slump-scars and chaotic deposits and covered by platform-derived redeposited limestones of Late Turonian to Campanian age, is known in literature within the basinal Calcari del Puriac (Bersezio et al., 2002; Carraro et al., 1970; Sturani, 1962). This documents different kinds of erosional processes (gravity sliding and platform shedding) during the Late Cretaceous. However, in coeval basinal sediments, cropping out close to the study area, no evidence of these phenomena occurs, which supports the hypothesis of the substantial primary absence of Cretaceous sediments in the studied successions. A very shallow burial environment can thus be argued for the Jurassic limestones at the time of their dolomitization.
From the foregoing considerations it follows that the dolomitizing fluids were at a significantly higher temperature than the ambient temperature of the host rocks, and thus they can be considered true hydrothermal fluids (sensu Machel and Lonnee, 2002; Davies and Smith, 2006). The spatial arrangement of the vein network, the occurrence of the breccia bodies, and their features point to a hydrothermal system characterized by several dolomitization pulses separated by hydrofracturing processes. The latter were due to the abrupt expulsion of overpressured fluids raising up along main fluid-flow pathways, likely represented by high-angle faults and related fracture systems. Hydraulic fracturing was commonly associated to dissolution of the host limestone (documented by geopetally filled cavities and type 3 breccias) and followed by dolomite precipitation (cementation of veins and cavities and replacement of the host limestone). This suggests that fluid composition was undersatured with respect to calcite and oversatured with respect to dolomite. If the overall picture of a superficial hydrothermal system is supported by field and laboratory data, further geochemical analyses are required in order to better define origin, composition and evolution through time of the dolomitizing fluids and to understand what caused precipitation of the sparry calcite cement which represents the last stage of filling of the still open pores.
7.3 Regional implications
The recognition of the described dolomite bodies has also important regional implications. Hydrothermal dolomitizaton indirectly documents a fault activity during the Cretaceous roughly along the transition zone between the Ligurian Briançonnais and the Provençal Domains. Structural and stratigraphic evidence of tectonic activity since Early Cretaceous has been indeed recognized in the adjacent External Ligurian Briançonnais Domain (Bertok et al., 2012), where approximately coeval, although less intense, dolomitization processes are known (Bertok, 2007).
Acknowledgements
The authors thank Simona Ferrando (Dipartimento di Scienze della Terra, Università di Torino) for guidance and assistance in fluid inclusion microthermometry. Thierry Dumont and an anonymous reviewer provided useful suggestions which improved the manuscript. The research was partly supported by funds from the Italian CNR (National Research Council), Istituto di Geoscienze e Georisorse, unità di Torino. A financial contribution by Italian Association for Sedimentary Geology (GeoSed) to L. Barale (2011) is kindly aknowledged.