1 Introduction
Our objective was to enlarge the fluorescence use in biosciences, in two fields of research, one mostly involved in the photodynamic therapy for the cancer treatment 〚1–3〛 and the other one more involved in the synthesis of fluorescent tracers as specific probes for biological cells labelling 〚4–6〛, a strong link between the two parts being fluorescence microscopy.
Photodynamic therapy is a rapidly growing modality for the treatment of light accessible tumours such as head and neck cancer, digestive and vesical tumours, cutaneous and subcutaneous cancer, as well as chest wall recurrence of breast cancer 〚7〛. PDT is based upon visible light-induced activation of photosensitising compounds, and results in an oxidative damage following the production of reactive oxygen species such as singlet oxygen (1O2) 〚8〛. As 1O2 intracellular diffusion is limited by its short lifetime to 0.01–0.02 μm 〚9〛, a correlation between dye localisation (investigated by fluorescence microscopy), organelles damages and mechanism of cell death has been suggested 〚10〛. m-THPC, a second generation sensitiser, is considered to be one of the most active photosensitiser in the clinical PDT of cancer 〚11〛. Moreover, PDT has been proposed in several studies as an alternative in overcoming ‘multidrug resistance phenotype’ (MDR) resulting in frequent failure of conventional chemotherapy 〚12〛.
In the present study, we have inspected the subcellular distribution, the efficiency as well as the cell death mechanism induced by m-THPC-based PDT in cells expressing ‘MDR phenotype’ (see section 2).
Because the rate of incorporation of the photosensitiser and especially its localisation are critical parameters for the PDT efficiency, our goal was to move firstly to the study of new fluorescent photosensitisers more selective regarding their localisation (glycosylated derivatives of porphyrin) and secondly to the development of the scanning near-field optical microscopy (SNOM), in order to set up a new optical way for the analysis of the different areas of the cells. The principle and the interest of these two approaches will be briefly mentioned (see section 3).
2 Applications of fluorescence microscopy for the photodynamic therapy
2.1 Experimental
2.1.1 Cells culture conditions
MCF-7 human breast cancer cell line and its subline MCF-7DXR were cultivated as described previously 〚1〛. The resistant subline, MCF-7DXR, was maintained in 10 μM doxorubicin-containing medium and is approximately 200-fold resistant to doxorubicin.
2.1.2 Cytotoxic effect of m-THPC-mediated photodynamic treatment
Logarithmically growing MCF-7 and MCF-7DXR cells preloaded with m-THPC (1.5 μM) were irradiated for 3 h at 650 nm with a dye laser (Spectra-Physics 375B), pumped with an argon dye laser (Spectra-Physics 2020, France) with an output of 0.3 W. Light spots of 7 cm diameter provided a fluence rate of 7.8 mW cm–2. Cell viability was assessed 24 h after illumination using MTT (3-(4,5-dimethylthiazol-2-yl)-2,5-diphenyl-2H-tetrazolium bromide, Sigma) colorimetric assay 〚13〛. Results are expressed as percentage of data obtained with non-irradiated drug-free controls.
2.1.3 Fluorescence microscopy
2.1.3.1 m-THPC subcellular distribution
Cells, plated onto Slideflasks (Nunc, Merck Eurolab, France), were incubated with 1.5 μM m-THPC for 3 h and observed immediately with an upright epifluorescence microscope (AX70 Provis, Olympus, France) equipped with a 100 W mercury vapour lamp. The specific filter set for m-THPC fluorescence detection (λex = 652 nm) consisted of a 400–440 nm band-pass (BP) excitation filter, a 570 nm dichroic mirror (DM) and a 590 nm long-pass (LP) barrier filter. Neutral density filters were used to reduce sensitiser photobleaching. m-THPC fluorescence images were recorded using a strictly controlled 2 s integration time. No interfering autofluorescence signal was measured in the experimental conditions. Microscopic images were recorded using a Peltier-cooled charge-coupled device camera (LH1600, Lhesa Électronique, France).
2.1.3.2 Organelles staining of MCF-7DXR cells
Lysosome and nuclear DNA were detected using acridine orange at 3.3 μM for 30 min. The filter set used to detect these acidic compartments consisted of a 400–440 nm or a 460–490 nm BP excitation filter, a 570 nm DM and a 590 nm LP barrier filter. Mitochondria were visualised after 30 min incubation with 5.2 μM rhodamine 123 and detected using a filter set composed of 460–490 nm BP excitation filter, a 505 nm DM and a 510–550 nm BP filter.
2.1.3.3 Cell death mechanism after m-THPC-mediated PDT: apoptosis vs necrosis?
Four hours after cell irradiation with specific light dose inducing 50% cell kill (LD50 determined by MTT test 24 h after treatment), cells were incubated with 2 μg ml–1 Hoechst 33342 (Molecular Probes) for 20 min at 37 °C and then labelled with 0.5 μM Sytox®Green nucleic acid stain (Molecular Probes) for 15 min at room temperature. Hoechst 33342 probe (detected using a filter set composed of 330–385 nm BP excitation filter, a 400 nm DM and a 420–460 nm BP filter) stained the nucleus of each cells. ‘Intact cells’ presented nucleus with uncondensed chromatin, ‘apoptotic cells’ were judged by the appearance of patches of condensed chromatin, fragmented nuclei and apoptotic bodies. Cells labelled with Sytox®Green nuclear acid stain (detected with a 460–490/505/510–550 nm set) and characterised by nuclear membrane alterations were undergoing necrosis. Such labelling permitted the distinction of apoptotic and necrotic cell morphological pattern by fluorescence microscopy and the subsequent evaluation of cell death mechanism induced by m-THPC-mediated PDT.
2.2 Results
2.2.1 Cytotoxic effect of m-THPC-mediated photodynamic treatment
Photocytotoxicity was assessed in both cell lines under the conditions of similar m-THPC uptake (1.5 μM, 3 h of contact); 24 h after m-THPC-mediated PDT, a decrease in cell survival in a fluence-dependent manner was observed (Fig. 1). The loss of cell viability was markedly enhanced in MCF-7DXR compared to MCF-7 parental cell line.
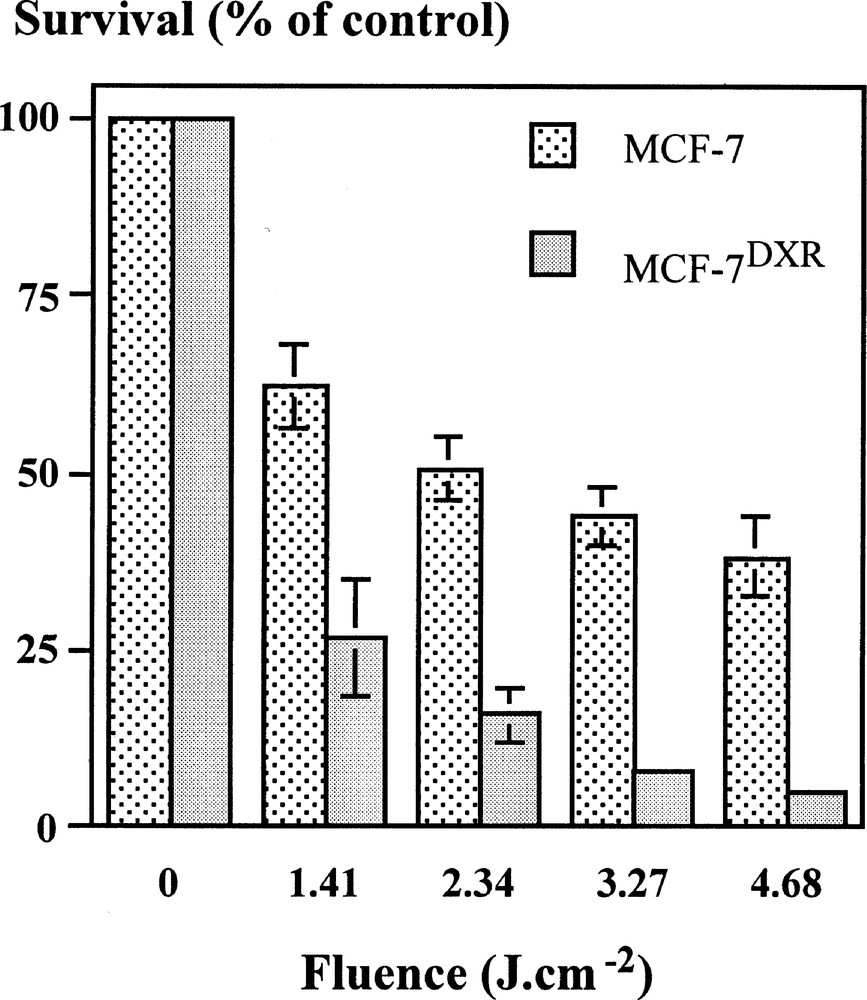
Photocytotoxicity of MCF-7 and MCF-7DXR cells exposed to m-THPC (1.5 μM) and red light irradiation (650 nm, 7.8 mW cm–2) at different light fluences. Survival was assessed by MTT colorimetric assay 24 h after light exposure. Data are the mean ± s.e.m. of at least three experiments.
2.2.2 m-THPC subcellular localisation
After 3 h incubation, m-THPC fluorescence images revealed a diffuse pattern of dye localisation throughout the cytoplasm with fluorescence intensification in the perinuclear area sparing the nucleus in both cell lines (Fig. 2). The only difference between the two cell lines was the presence of punctate bright fluorescence pattern throughout the cytoplasm in MCF-7DXR (Fig. 2b). This punctate localisation was very different from rhodamine 123 mitochondrial staining (Fig. 3a), but seemed to correspond to acidic organelles, especially lysosomes as shown by acridine orange staining (Fig. 3b).
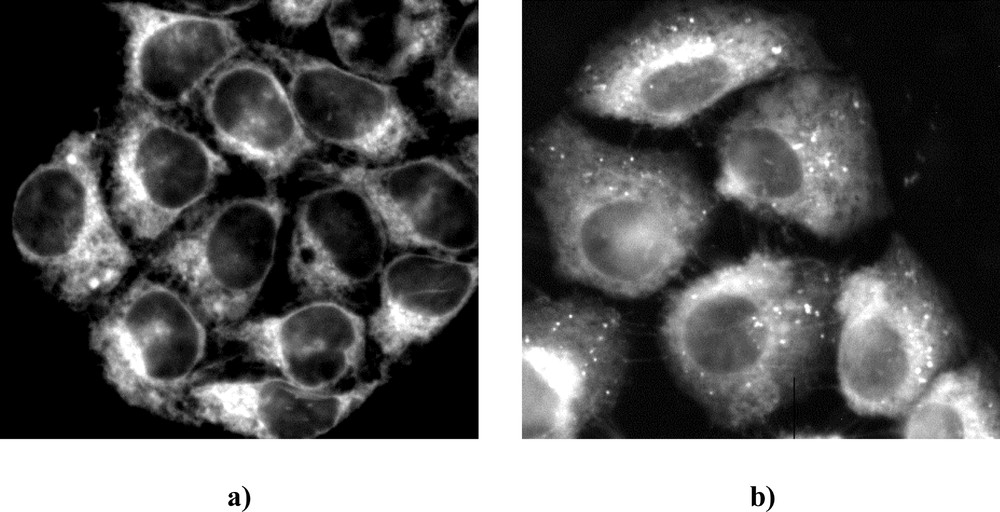
Distribution of m-THPC fluorescence in MCF-7 (a) and MCF-7DXR (b) cells following 3 h of incubation with the drug (1.5 μM).

Typical fluorescence staining of subcellular organelles of MCF-7DXR cells. Acidic organelles (a) were stained with acridine orange (nucleus in green and lysosomes in red), mitochondria (b) were stained with rhodamine 123.
2.2.3 Cell death mechanism after m-THPC-mediated PDT: apoptosis vs necrosis?
Double staining of cells nuclei with Hoechst 33342 and Sytox®Green enabled us to distinguish necrosis and apoptosis cell death pattern (Fig. 4). No main difference was observed between MCF-7 and MCF-7DXR cell lines. Twenty-four hours after PDT, necrosis was found to be the main mechanism of cell death in both cell lines, apoptosis being limited to a few percent of the cell population.
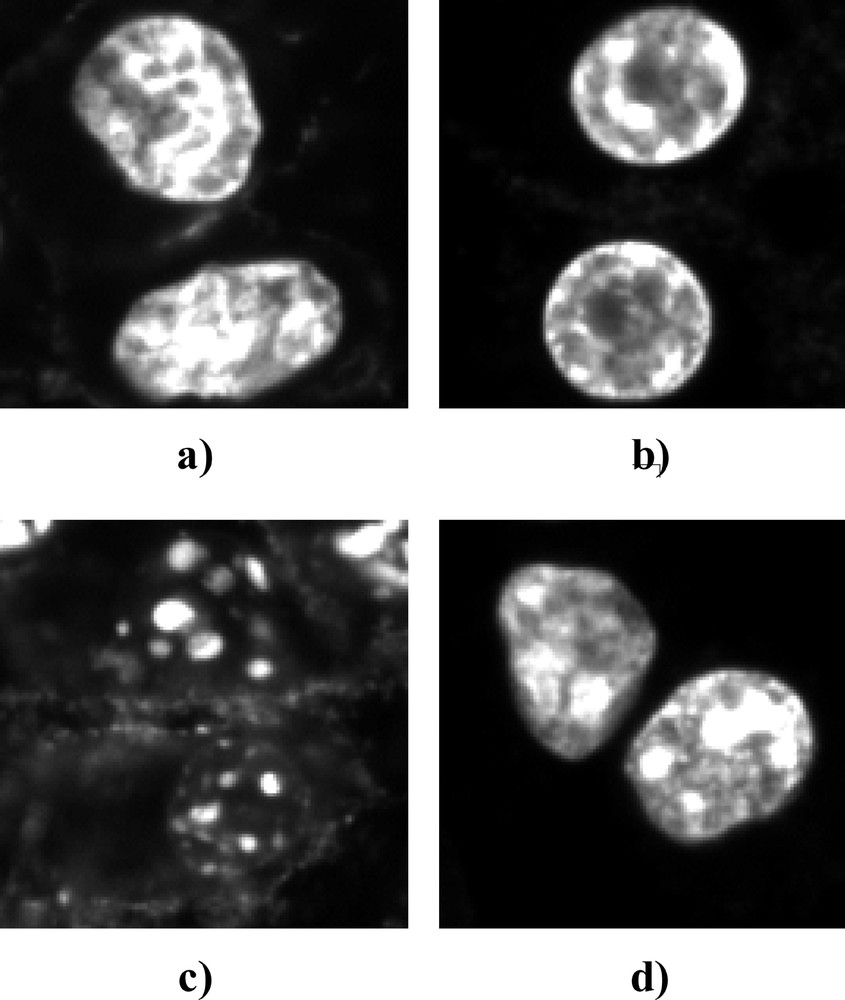
Representative fluorescence microscopy images for MCF-7 cells: intact cells (a), early apoptotic cells (b) and apoptotic bodies (c) labelled with Hoechst 33342; necrotic cells (d) identified by Sytox®Green acid staining.
2.3 Discussion
According to several studies proposing PDT as an alternative way of eradication of MDR cells 〚12, 14〛, we found a higher cytotoxicity of m-THPC-mediated PDT in MCF-7DXR than in MCF-7 cells, despite of a similar m-THPC uptake in both cell lines.
Consistent with our previous work 〚2〛, m-THPC was found diffusely localised throughout the cytoplasm outside the nucleus, indicating general staining of cellular organelles. The only difference in the m-THPC distribution pattern between MCF-7 and MCF-7DXR cells consisted in the appearance of distinct bright fluorescence spots that could be critical targets for m-THPC-PDT cellular toxicity observed in MCF-7DXR. Comparison of m-THPC distribution with organelles staining suggested m-THPC trapping in acidic organelles such as lysosomes/endosomes. Experiments using fluorescence microscopy also permitted to evaluate that necrosis is the major cell death mechanism induced by m-THPC-mediated PDT in these two cell lines.
3 Towards new routes for the photodynamic therapy
3.1 Glycosylated fluorophores as new tracers and photosensitisers
Recently, we have devoted our synthesis work to obtain coumarin-like fluorophores with a glucosamine ring (Fig. 5) 〚6〛. The localisation of these tracers is strongly dependent on the glucosamine functions, either hydroxyl (R = H) or acetyl (R = Ac). As an example of specific localisation, we recall the result previously published for the tracer CNCONHGluOAc with the endothelial cells, the fluorescence analysis indicated that the tracer labelled the endoplasmic reticulum of endothelial cells (double-labelling process and analysis using 3-D fluorescence microscopy with the CELLscanTM method 〚6〛).
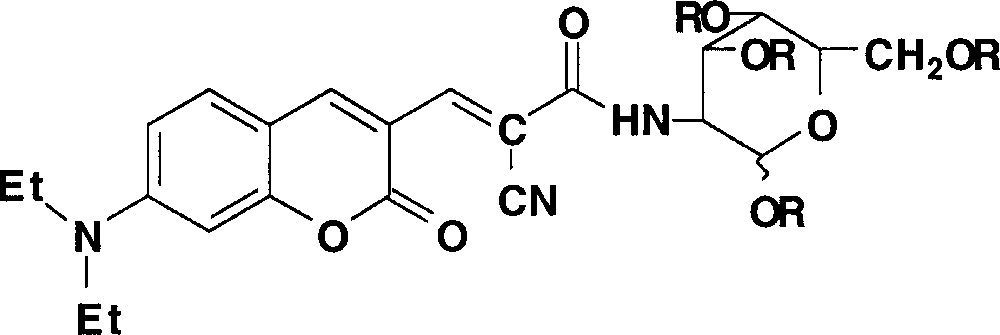
Chemical structure of coumarin like fluorescent tracers with a glucosamine ring. R = H: CNCONHGluOH; R = Ac: CNCONHGluOAc.
In order to move to the PDT research, we retained the synthesis of a porphyrin–sugar conjugate by linking a carbohydrate to the carboxylic acid moieties of the protoporphyrin IX (Fig. 6). Indeed, water soluble porphyrins have been shown to have a special affinity for some biomolecules and cancer cells. Especially, in the case of cancer phototherapy, the sugar appears to increase water solubility, membrane interaction, specific receptor targeting 〚15〛 and plasmatic lifetime 〚16〛.

Chemical structure of phorphyrin-like photosensitiser with glucosamine rings (R = H or Ac).
Among the works that have been done concerning the synthesis of glycosylated porphyrins, only a few concerned the protoporphyrin IX. The introduction of the glucosamine ring was previously performed by Fuhrhop et al. 〚17〛, but no study has been done to know the biological response to these modified photosensitisers. The synthesis of the target porphyrins is presently under progress.
3.2 Scanning near-field optical microscopy imaging
Biological cells exploration by SNOM has recently been developed 〚18–21〛, permitting to produce simultaneously topographical, optical (through signal transmission or reflection) and fluorescence emission images without damaging the biological material. Adaptation of the technique to liquid medium experiments was also reported 〚18〛.
Here, in order to demonstrate the potentialities of the method, we report the images related to the analysis of reference specimens like a polymer film containing calibrated fluorescent beads or supported lipid monolayers (images done at SIMS-NRC, Ottawa, Canada, through a scientific collaboration with Dr L. Jonhston 〚19〛): (i) Fig. 7 corresponds to the analysis of a 200 nm fluorescent bead included in a polymer film (Fig. 7, image a: topography and Fig. 7, image b: fluorescence emission) and (ii) Fig. 8 represents the near-field fluorescence image for a monolayer of DPPC (1 mg ml–1) labelled with BODIPY (0.5% weight) at 25 °C (the monolayer was transferred onto a mica surface at the pressure of 5.5 mN m–1), which indicates the presence of BODIPY in the liquid expanded (LE) of the monolayer. These images point out the capacity to obtain imaging of nanometric-size objects.

Polymer fluorescent bead (200 nm): topography (a) and fluorescence emission (b).
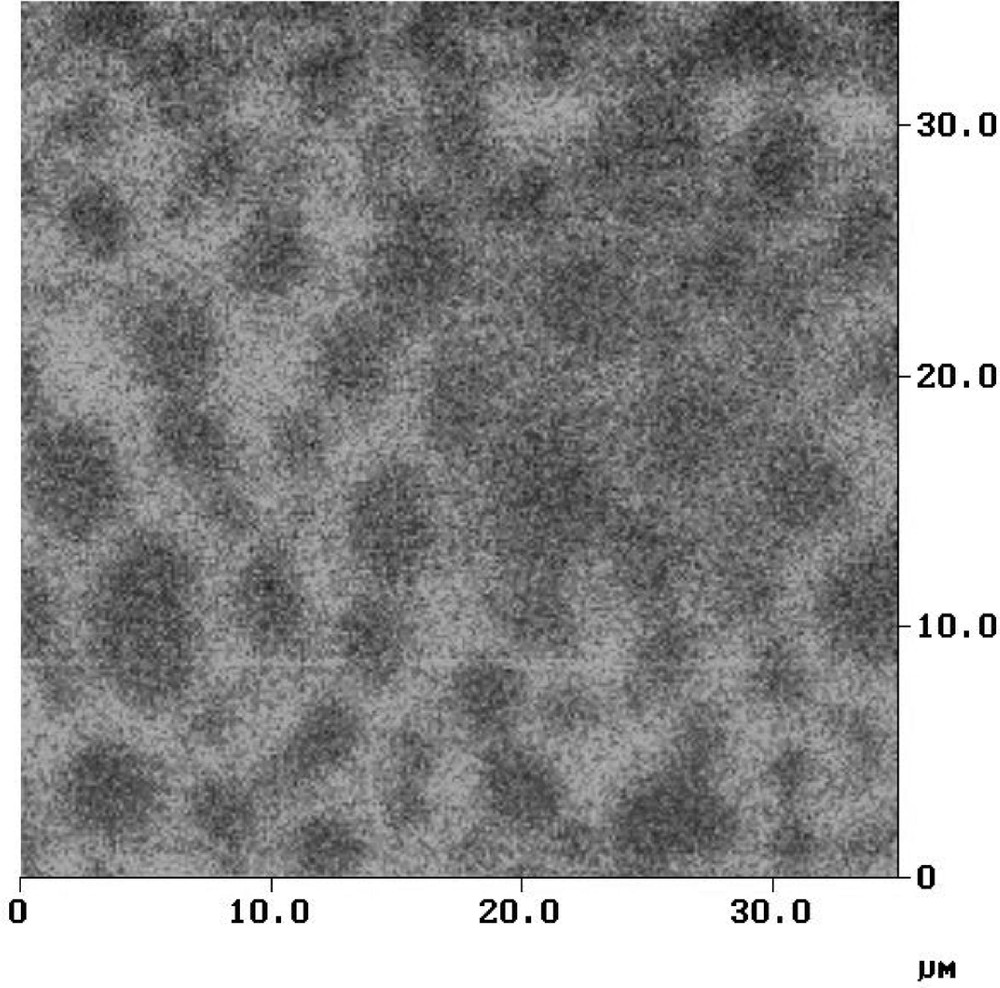
Near-field fluorescence image of a 35 μm × 35 μm region of DPPC/0.5% weight BODIPY monolayer.
4 Conclusion
m-THPC sensitiser, which has proven its cytotoxic effect, has exhibited fluorescence properties valuable for distribution and localisation studies.
Moreover, in order to orientate the localisation of photosensitisers, we start to develop their association with hydrophilic sugar ring. Even if the chemical way for the synthesis does not follow the one previously used for coumarin-like fluorescent compounds, our first results related to other synthetic routes are promising for porphyrin derivatives.
The SNOM technology seems also very promising to detect nanodomains, but despite of several improvements, it appears that work remains to be done to obtain the required conditions for the investigation of biological media.
Acknowledgements
We thank the French MEN–MR, the CNRS, the INSERM, the ‘Région Lorraine’, the CUGNancy, the French ‘Ligue nationale contre le cancer’ and ‘Comités lorrains’ for grants. We thank Dr L. Johnston, SIMS, Ottawa, NRC Canada for her collaboration and for her hospitality during the journey of P. Burgos. We are grateful to the National Research and Education Ministry of Luxemburg for awarding a scholarship to M.-H. Teiten.
Version abrégée
Avec pour objectif principal d’accroître les potentialités de la fluorescence pour les biosciences, nos travaux concernent, d’une part, l’étude de fluorochromes utilisés en thérapie photodynamique (PDT) pour le traitement de certains cancers et, d’autre part, la synthèse et l’étude photophysique de nouveaux traceurs fluorescents spécifiques de marquage cellulaire. Ces deux approches sont fortement en interaction par l’utilisation de la microscopie de fluorescence (champ lointain et champ proche).
En premier lieu, nous décrivons les résultats concernant l’utilisation de la méta-tétra(hydroxyphényl)chlorine (m-THPC) comme photosensibilisant de seconde génération pour la PDT. L’étude de sa localisation dans des cellules humaines de cancer du sein (MCF-7 et sa lignée résistante MCF-7DXR) a été réalisée par microscopie de fluorescence, afin d’appréhender les cibles impliquées dans la photo-inactivation des cellules et d’évaluer les mécanismes de mort cellulaire induits. Pour une incorporation intracellulaire similaire dans les deux lignées, la m-THPC présente une cytotoxicité plus importante dans la lignée résistante MCF-7DXR en comparaison de la lignée parentale MCF-7. Cette différence d’effet phototoxique semble liée à une différence de localisation intracellulaire de la m-THPC, qui se traduit par une séquestration ponctuelle de ce photosensibilisant dans des vésicules intracytoplasmiques dans la lignée MCF-7DXR. Ces « spots fluorescents » semblent correspondre aux lysosomes (hypothèse émise après comparaison avec des marquages d’organites). D’autres expériences en microscopie de fluorescence permettent d’évaluer la nécrose comme mécanisme majeur de mort cellulaire dans les deux lignées étudiées.
En second lieu, nous présentons des travaux selon deux nouvelles voies de recherche, qui ont pour but d’améliorer les études en PDT. Il s’agit, d’une part, de la synthèse de traceurs fluorescents modifiés par la présence d’un cycle hydrophile glucosamine pour orienter leur localisation à l’échelle cellulaire (localisation préférentielle dans le réticulum endoplasmique de cellules endothéliales pour un traceur de type coumarine–glucosamine acétylée ; synthèse de porphyrines glycosylées) et, d’autre part, de l’utilisation de la microscopie optique en champ proche pour atteindre, avec une meilleure résolution, les sites de fixation des traceurs (visualisation de monocouches de DPPC marquées avec un traceur BODIPY).