1 Introduction
Although collective prey capture is widespread in nature, cooperation during prey transportation or retrieval is an uncommon phenomenon. Retrieval of prey and transport to the nest is well known for ants [1–5]. Working together, ants may transport prey that are 5000 times heavier than any individual ant [6]. Among social Arthropods, only a few species of social spiders are able to collectively transport prey. These species are able to capture a wide range of prey, differing in length and genus. This is especially true for the South American social spider, Anelosimus eximius Keyserling (Araneae, Theridiidae) that can capture prey that vary in size from less than 5 mm up to more than 50 mm [7,8]. The dry weight of the prey can be 700 times heavier than the dry weight of one spider [8]. Despite the impressive aspect of this phenomenon there are only a few studies concerning the mechanisms underlying cooperative transport, even in ants [5,9]. The roboticists were the first to model collective transport based on data available for ants [10,11]. Information on the transport of captured prey by social spiders is sparse (Agelena consociata [12], Stegodyphus sarasinorum [13], A. eximius [14]), and has not been detailed in A. eximius at all. Here we wish discover how the cooperative behaviour, observed during prey transportation by social spiders, is achieved.
Two main hypotheses can be proposed: one complex and the other simplistic. Under the complex hypothesis each individual possesses a global representation of the situation, for example the length and weight of a prey item. It also assesses the number of nest mates already involved in the capture effort and uses these two pieces of information in deciding whether to participate in transport of the captured prey. But no experiment on social Arthropods, to the best of our knowledge, argues that individuals make such assessments and use such a global representation. Under the simple hypothesis it is possible to explain complex collective behaviour by assuming that each individual reacts only to local information that emanates mainly from the prey (movement, retrievability) and would not require any information about the overall situation (number of conspecifics participating). This corresponds to the theory of self-organisation. Self-organisation can be defined as all processes in which ‘complex collective behaviour may emerge from interactions among individuals that exhibit simple behaviour: in these case there is no need to invoke individual complexity to explain complex collective behaviour’ [15]. Self-organized process has been applied effectively to better understand collective behaviour of birds [16], of hares [17] and of social insects [18–20]. Concerning collective transport, Detrain and Deneubourg [9] have shown, by modifying the characteristics of the prey of the ant specie Pheidole pallidula, that the tractive resistance of prey was the key parameter the forager used to estimate prey size and that it ruled their trail-laying intensity. Franks et al. [5] have shown in the army ant that the front-runner who initiates prey retrieval is typically unusually large and that the second-largest ant is typically unusually small. They explained this distribution by the fact that other large ants found that the remaining work was too slight to employ their full efforts and hence chose not to participate to the transport. Smaller ants, whose efforts were fully employed, then joined the team.
We try here to show that self-organising processes can explain how spiders adapt themselves to the transport of a wide range of prey and could permit the understanding of the variation of individual participation in predation. The ultimate goal is to gain a better understanding of how the adaptive foraging response can emerge from simple mechanisms in a social evolutionary perspective.
Predation in A. eximius can be divided into three steps: recruitment, capture and transport [8,14]. Krafft and Pasquet [21] have investigated the recruitment phase of cooperative foraging and several studies have examined the cooperative prey capture sequence itself [7,8,14,22,23]. Studies have shown that during recruitment more spiders approach and join in attack when encountering prey with a high resistance [7,8,13,24,25]. The number of individuals participating in each step has not been quantified nor has the mechanism by which the recruitment occurs been analysed. The mechanism may well involve vibratory signals [21,23,26]. Vakanas and Krafft [23] have shown that vibratory signals, emitted by the prey, are at the origin of the coordination of the behaviour of spiders in a group foraging event. During capture the observed coordination of A. eximius does not need direct communication between individuals but is the result of the adaptation of each individual to the vibratory state of the prey [23]. However, we do not have similar information for prey transport. During transport, as an aid to the movement of the prey, spiders weave silk that we named ‘traction silk’, fixed between the prey and the web (in the direction of the shelter) that will permit a slight lifting of the prey. This process will be repeated until the prey has been transported under the shelter. Natural observations revealed that the number of individuals that were participating in predation varies with the prey type [8] and varies between the different steps of the predation (personal observations). Nevertheless there is no data, no quantification of the number of individuals participating in the different steps of predation, especially the transport. This paper quantifies the variations of the number of individuals participating in predation of different prey types. We will focus our study on the transport steps because the mechanisms involved in capture have already been studied [23]. To sum up, how is the number of individuals that participate in transport of different prey types regulated?
For this purpose and with a self-organising approach we have studied the influence of some characteristics of the prey on transportation: the mass, the length and the vibrations emitted by the prey. To underline which characteristic is decisive we have studied the characteristics separately. Vibrations were expected to play a major role during recruitment with few spiders recruited when the prey generates few vibrations. By varying only the length of the prey (identical masses) we did not expected to influence the number of transporters because we thought it was the mass that would influence the number of spiders participating in transport. Indeed, difficulties in pulling the prey may ‘attract’ new spiders during the transport. Hence the heavier the prey, the greater the number of transporters should be.
2 Materials and methods
2.1 Biological material
A. eximius were collected from four colonies in French Guyana in February 2000. In the laboratory, we housed approximately 300 spiders in each cage measuring (length × height × width). Spiders from different colonies were mixed together because Pasquet et al. [27] report that there is no recognition of nestmates in A. eximius (A. eximius do not have closed groups). The cages had ventilation on the sides and removable glass fronts and backs . The room housing the cages was maintained at a temperature of 26 °C and 70% humidity at day and 18 °C and 80% humidity at night. The laboratory photoperiod was 12 h of light and 12 h of dark, the dark starting at 6 pm. Crickets (Gryllus campestris) and flies (Lucilia caesar) were offered daily to the spiders and distilled water was sprayed into the cages twice daily.
2.2 Experimental device
The experimental chamber consisted of a Plexiglas box of , divided into two compartments by a Plexiglas block. The chamber had a removable glass front and back. We used these chambers to film two groups of spiders simultaneously, one in each compartment. Each compartment had ventilation (removable) on the side as well as two openings, one in the middle of a side that allowed prey to be introduced, and the other in the roof that enabled dead prey to be withdrawn. With the help of strips of black paper on the top we created a dark zone . Spiders were sheltered under this zone (named shelter); using it as they would use the shadow of a leaf under natural conditions. A Sony digital camera filmed the entire box (i.e. the two compartments). Fluorescent tubes placed near the two sides permitted uniform illumination of the box.
Each observation was performed on groups of 20 spiders (selected from the cages). Groups of 20 spiders were the maximum we could use to allow a difference in the number of spiders participating in the predation to be observed since spiders were not marked. Using more than 20 spiders was not feasible because it became impossible to differentiate between different spiders on the prey. We used 20 different groups for each experiment except for the wingless flies where we used 22 groups. We only used adult females because males do not participate as much in prey capture [28]. Since participation in predation is modulated by spider body weight [25], females were chosen with approximately the same abdomen size. Each group was only used once for statistical validity.
2.3 Protocol
Each experiment was initiated one week after the introduction of the 20 spiders into the compartment. During the week preceding the start of the experiment, each group of spiders was fed with one fly (Lucilia caesar) every other day. The remains of these preys were removed one day prior to the start of the experiment. Flies subsequently were used as the prey type in the experiments and were of approximately the same size as each other (about 1.2 cm).
Experiments were performed at the end of the day (between 4 pm and 8 pm), when spiders are most active [8]. The prey was introduced through the lateral opening. Once in the web, the vibrations it produced attracted spiders coming from the shelter (recruitment step). The initiation of the capture sequence was defined as the first physical contact between a spider and the prey and the end when transport begun. Transport begun with the physical movement of the prey item from the capture site and ends when the prey was no further displaced for a period of three minutes. The prey was transported , in the majority of the cases, in the direction of the shelter (90%) where it was eaten by the spiders.
We observed several variables. First, the total number of spiders that made contact with the prey (once the prey was introduced): the number of contacting spiders. Second, the total number of individuals that had participated in transport, disregarding their participation duration: the total number of transporters. Third, the number of individuals that participated during the first minute of the transport (disregarding their participation duration): the number of first transporters. The comparison of these last two variables permitted us to detect any new arrival of spiders during transport. Forth, the mean number of individuals that participated, at any time, during transport. This number permitted us to take into account the variation occurring in individual participation during transport. This number was calculated by divide the sum of each individual participation duration by the transport duration . For simplification we called this number: mean number of transporters . Finally we observed the transport speed : the time between the beginning of transport and its end, divided by the transport distance. To make easier its readability we have multiplied it by 1000. The length of transport was the distance between the initial and the final position of the prey. We measured this distance directly on the screen during the video analyses of the predation with respect to the scale. The prey was always transported vertically so we used a 2-dimensional representation.
We did not measure vibrations transmitted by the web because the irregularity of the web makes every measurement very difficult and because of the perturbations it could generate. As no similar study has already been done, we have avoided making any experimental intrusion in the web to prevent any perturbation.
2.4 Prey characteristic treatments
To study the influence of the mass of the prey we carried out predation sequences using flies that had a mean weight of . The flies were ballasted with a fishing-weight weighing . The fishing-weight was flat and glued onto the thorax of the fly. Results were compared with results from the standard groups . Standard groups had received flies that had a mean weight of . Ballasted flies were significantly heavier than standard flies: versus (non-paired t test: , ). Results obtained were compared with results from the standard . Therefore, the only factor that was modified was the mass.
To study the influence of the prey length we attached a fly to a second fly using a metal wire that was glued between the head of the first fly and the abdomen of the second. The two flies were close together. The first fly was dead to ensure only one fly vibrated the web. We did not observed, at first sight, differences in the wing-vibration of the living fly glued to a dead fly compared to a living fly alone. As it will be shown later, spiders were similarly attracted onto the two types of prey. Weights of elongated and ballasted flies were not significantly different: versus (non-paired t test: , ). Results obtained with this artificially elongated prey were compared with results from experiments with the ballasted flies. Therefore, the only factor that was modified was the length.
To study the influence of the vibration emitted by the prey we recorded predation sequences on flies from whose wings had been cut. The two types of prey had the same weight: versus (non-paired t test: , ) and length (about 1.2 cm). Results obtained were compared with results from the standard . The only factor being modified was the vibration emitted by the fly.
3 Statistical tools
For the data concerning the mean number of transporters, the transport distance and transport speed, we tested whether the data were normally distributed (Kolmogorov–Smirnov normality test) and whether there was any significant difference between the variances (equality variance F test). If these conditions were met parametric tests (ANOVA test and Bonferroni–Dunn test for multiple comparisons) were used, if not, non-parametric tests were used (this was the case for the transport length).
Non-parametric tests were applied in the comparison of the number of individuals that had participated in predation (number of recruited spiders, number of first transporters and number of total transporters). The Kruskal–Wallis test (K–W test) was performed to compare all the groups together. A test for multiple comparisons for non-parametric data [29] was used to compare the different groups (two by two). As the Friedman test revealed significant differences between the number of individuals contacting the prey, the number of first and total transporters in each treatment (Friedman test – control: , , ; ballasted: , , ; elongated: , , ; wingless: , , ; Fig. 1), we used the Wilcoxon test to compare the number of individuals within an experimental group (since an individual spider can participate in each step of the capture).

Action of the prey mass, length and vibration on the evolution of the number of spiders having contacted the prey and having participated in transport (first and total transporters). Results are median, first, and second interquartile ranges, and the lower and upper bars represent the 10th and 90th percentiles, respectively. Only significant results are shown. Statistics are K–W post hoc test and Wilcoxon tests; .
4 Results
4.1 Recruitment stage
Different numbers of spiders contacted the four different prey types (K–W test: , ; Fig. 1). The significant result is due to fewer spiders contacting the wingless fly (Post-hoc K–W test, , , ; Fig. 1), while the other prey types did not differ in how many spiders were attracted (post-hoc K–W test, p: ns; Fig. 1).
Moreover, as a general pattern in each of the treatments, there were more spiders contacting the fly than actually participating in initial transport (number of first transporters) (Wilcoxon signed-ranks test: standard prey: , , ; ballasted prey: , , ; elongated prey: , , ; wingless prey: , , ; Fig. 1).
4.2 Number of transporters
In the elongated flies predation, one group had not transported the prey so concerning transport variables. Finally during wingless flies capture, four fly were not captured so concerning transport variables. If we consider all the groups together we found some significant differences in the transport speed (ANOVA test: , ; Fig. 2), in the mean number of transporters (ANOVA test: , ; Fig. 2), in the number of first transporters (K–W test: , ; Fig. 1), and in the number of total transporters (K–W test: , ; Fig. 1).
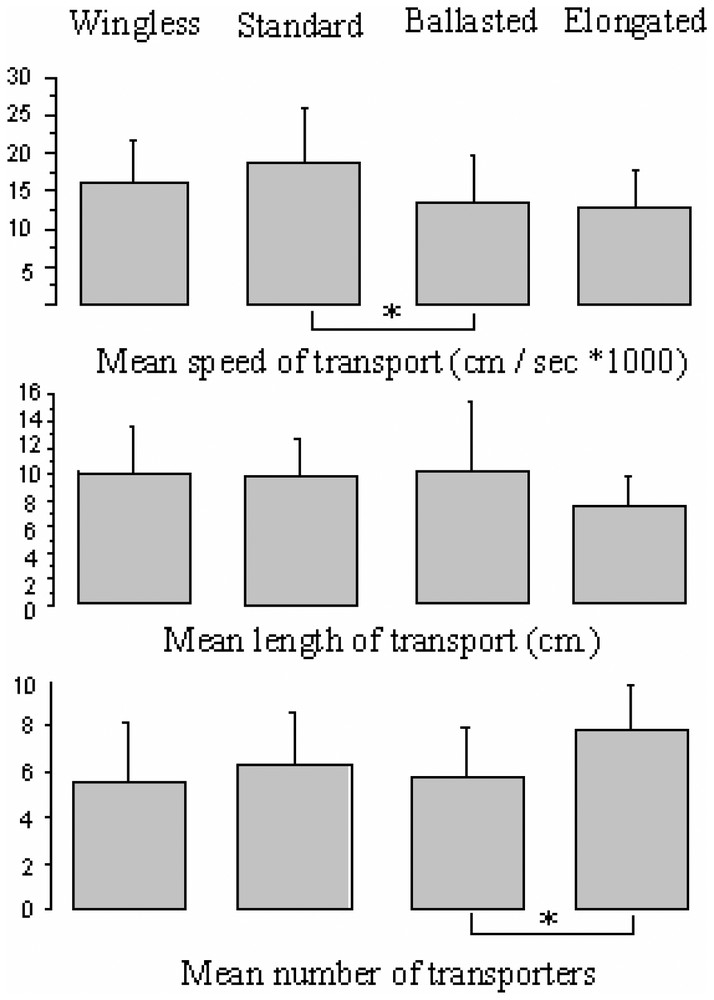
Action of the prey weight, length and vibration on transport duration , transport length , and on the mean number of transporters . Only significant results are shown. Statistics are Bonferroni–Dunn test; .
The number of first transporters was higher in elongated fly transport than in ballasted fly transport (post-hoc K–W test, ; Fig. 1) and was higher in standard fly transport than in wingless fly transport (post-hoc K–W test, ; Fig. 1), whereas no difference was observed between transports of standard and ballasted flies (post-hoc K–W test, p: ns; Fig. 1).
The mean number of transporters was higher during transport of the elongated fly than during transport of the ballasted fly (Bonferroni–Dunn test, , , ; Fig. 2; in two cases during ballasted flies predation, it was impossible to differentiate between individuals during the totality of the transport so ), whereas no difference was found for the other categories of flies (Bonferroni–Dunn test, p: ns; Fig. 2).
The number of total transporters was higher in ballasted fly transport than in standard fly transport (post-hoc K–W test, ; Fig. 1) whereas no difference was observed for the other categories of flies (post-hoc K–W test, p: ns; Fig. 1).
The number of total transporters was higher than the number of first transporters in all the categories of flies, except the standard fly (Wilcoxon signed-ranks test: ballasted fly: , , ; elongated fly: , , ; wingless fly: , , ; standard fly: , , ; Fig. 1).
4.3 Duration and distance of transport
The standard fly was transported faster toward the shelter than the ballasted fly (Bonferroni–Dunn test, ; ; Fig. 2), whereas no difference was found for the other categories of flies (Bonferroni–Dunn test, p: ns; Fig. 2).
No difference in the transport length was found between all the categories of flies (K–W test: , ; Fig. 2). Nevertheless, we noted that the distance of double-prey transport had a tendency to be shorter than ballasted prey transport. Indeed the elongated prey tended to be captured nearer the opening in the box where the prey was introduced, possibly because of its larger dimensions. The surface area of the prey in contact with the web is indeed higher than in the case of a standard prey, causing the prey to move with more difficulty.
5 Discussion
Results permit us to confirm, with quantified data, that the number of individuals participating in the different steps of the predation, especially the transport, is fluctuating. Thus, the number of recruited spiders is, in all our experiments, higher than the number of spiders participating in transport. Therefore, all the spiders that have contacted the prey will not be involved in transport (in all prey types). In addition to that, results show that the number of total transporters can be higher than the number of first transporters. In such a case there was arrival of new individuals during transport. This has significantly been observed during the transport of all the prey types except the standard prey. We better understand why these variations appeared thanks to the study of the influence of some characteristics of the prey.
Prey vibrations play a major role in the recruitment step. Once the prey has fallen into the web, its vibrations attract spiders. When vibrations had a lower intensity and/or were qualitatively different (wingless fly vs. standard fly) they caused, apparently, the web to vibrate less. As a consequence, in the case of the predation of a wingless fly, there were fewer spiders entering into contact with the prey and therefore, fewer spiders were involved in the beginning of the transport. In this case four wingless flies were not captured. Contrary to our expectations the prey mass did not seem to influence the mean number of spiders participating in the transport even if the total number of transporters was higher than with standard prey. Conversely, when the prey item was heavier, transport was more difficult as demonstrated by a slower transport speed. On the other hand, the prey length seems very important in the regulation of the number of spiders that participated in transport. In the case of the elongated prey more spiders participated at the beginning and during the transport (mean number of transporters) compared to ballasted prey transport. As the prey compared have the same mass we can indeed conclude that it is the length that is responsible for this variation.
These results, for the first time, permit us to better understand the mechanisms involved in collective prey transport and lead us to do several conclusions. Firstly, our results confirm the importance of vibrations during the recruitment. This has already been studied by Burgess [26] who showed the importance of the frequency and the intensity of vibratory signals on the predatory behaviour of Mallos gregalis, with no or few individuals recruited with a non-buzzing prey. Vollrath [24] and Ebert [25] noted that small dipterous produce less vibration than big prey and so will be detected only by the nearest spiders. The silk network plays a fundamental role in this dynamic by diffusing vibrations in a space whose area depends upon the prey's vigour. Contrary to Pasquet and Krafft's [8] hypothesis that the lower level recruitment observed during grasshopper capture compared to moth capture could result from different tactics adopted by the spiders (selected by evolution), with the aim of diminishing the risk of injury, we suggest that this different level results from the difference in the vibrations emitted by the prey, with no relation with the prey dangerousness. Natural observations show that buzzing prey provokes more spiders to be recruited than non-buzzing prey. The recruitment we observed probably does not result from direct communication between the spiders. We have observed no spider pulling the silk that could have attracted other spiders. It is the prey itself that ‘recruits’, by its vibrations, the required number of individuals. It is however possible that a second mechanism, linked to the synchronised movement observed during the approach to the prey, intervenes. Krafft and Pasquet [21] showed that spasmodic movements (especially observable when the prey moves quickly in the web) of the spiders provoke quantifiable vibratory phenomena and they suggested that it could recruit inactive spiders.
Secondly, our results (in all prey types) show that some spiders that contacted the prey did not help to transport it. Likewise, some spiders do not participate in the entire capture [23] probably because too many spiders contacting the prey will result in the departure of some spiders. The number of spiders that participate at the beginning of the transport depends upon the number of spiders that are recruited and hence that were capturing. But we also observed, during the recruitment step, that some spiders return to the retreat probably because the prey was already immobilised, hence they were no more attracted by its vibrations. On the other hand results lead us to hypothesis that vibrations produced (involuntarily) by the movement of the spiders during the transport of the prey will probably alert new individuals that will join the transporters. Indeed, when there are some difficulties in pulling a fly, spiders will do more round trips with the aim of transporting the prey toward the shelter. This could explain why there were new spiders arriving during transport of ballasted and elongated flies. In the case of the wingless fly the few numbers of first transporters makes transport more difficult. Hence, the situation was comparable with ballasted prey transport and new spiders were attracted. That permits, even with a low number of individual participating at the beginning of the transport, a mean number of transporters comparable to that of standard prey transport.
Finally, our results and our observations reveal the importance of the prey length in the regulation of the spider participation in transport. Indeed, why was the mean number of transporters during ballasted fly transport not higher than during standard fly transport? This should ameliorate the transport speed. We found a possible response regarding elongated flies transport. With elongated flies the mean number of transporters was higher than with ballasted prey, probably because the number of available sites for pulling the prey is greater on a larger prey. Observations suggest (in standard flies transport) that if there were too many spiders close to the fly, some spiders will give up in transporting the prey. This is partially confirmed because there are, at the beginning of the transport, more individuals on the elongated prey than on the ballasted prey. In the case of the ballasted flies transport, the lack of available sites on the prey probably limits the number of transporters. This might seem unlikely, but in fact under natural conditions the weight of the prey grows with the prey length as showed by Schoener [30] in temperate and tropical forest insects. So these two factors (length and weight) are inextricably linked. Nevertheless another hypothesis that worth considering is that the prey weight could influence the probability of the spider being involved in transport because of its effect on tautness of the silk used to pull the prey during transport. Perhaps a spider would participate more if the silk was tight (and if the available sites are numerous enough).
To summary, our study shows a possible mechanism through which spiders could have adapted for the predation of various prey. Vibrations play a major role in the regulation of an individual's participation in each step of the predation (recruitment, capture and transport). The second important factor is the number of available sites on the prey that make spiders participate or not. Therefore, it is not necessary to refer to the intervention of a direct communication between spiders to explain efficient predation, nor to the adoption of a specific group strategy for each sort of prey. It has not been mentioned (except by Bradoo [13]) that active recruitment exists in social spiders during predation and so during transport. This does not preclude, as we have shown, that during transport in A. eximius there is an arrival of new spiders. These spiders are certainly attracted by the vibrations produced (involuntary) by the spiders that are actively transporting the prey. This absence of a specific recruitment signal, i.e. a signal that the spider made with the aim of attracting other spiders, is an important difference with social insects and particularly ants. An ant, which after a few minutes cannot displace a prey, will actively recruit conspecifics by producing a trail [9] or by emitting volatile pheromones [31]. This will be important in a social evolution context (developed further).
Therefore, the efficiency of the colony can be explained from self-organised processes. The single fact that individuals respond locally to the environmental stimuli (vibration intensity, available site, perhaps the silk tautness) permits an explanation of the regulation of individuals participating in all the steps of the predation (recruitment, capture and transport). Therefore, the coordination in collective transport seems to occur through the item being transported: movement of one spider engaged in group transport is likely to modify the stimuli perceived by the other group members (such as vibration produced, or indirectly, available site on the prey) possibly producing, in turn, recruitment or departure of individuals. Such a result, in social spiders, is in agreement with results obtained in prey retrieval in ants [5,9] and in the regulation of ant's foraging to the honeydew production of aphids [32]. Thus coordination and efficient predation emerges from the group functioning without the necessity of using direct communication between individuals. In fact spiders cooperate indirectly. Coordination in spider colonies is based on signals that are made inadvertently as side products of their activities. The communal network, as a means of information, seems to be at the origin of cooperation. This supports the hypothesis of a sudden passage from solitary to social life in spinning spiders [23,33–35]. As soon as individuals are tolerant and if environmental conditions become favourable spiders can live close together and collective tasks such as predation will emerge from the group functioning (there is no need to develop complex communication system as in ants).
To confirm and enlarge our results it will be very interesting to do experiments under natural conditions where preys are more diverse. Such results would permit a cooperation with computer scientists into the modelling of and hence prediction of, the number of spiders that can intervene in the different predation steps. This work is among the first to try to gain a better understanding of the collective decision during collective transport in social spiders and consequently a lot of facts still remain to be understood. To be able to measure and quantify vibration transmitted by the silk in such a situation is a challenge that could give valuable further information.
Acknowledgments
The National ‘Cognisciences’ program of the CNRS supported this study. We thank C.N.R.S. to have permitted us to collect spider in French Guyana. We thank Dr Desor, Dr Bernard, Dr Hill for theirs suggestions and Mr Moncotel, Mr Vogel and Ms Leclerc for their helps in video, apparatus device and spiders maintenance.