Version française abrégée
Le genre Acacia, avec plus de 1350 espèces, correspond au deuxième plus grand genre de la famille des légumineuses. Le sous-genre Phyllodineae forme un immense groupe de plus de 960 espèces, principalement présent en Australie. Ces espèces sont connues pour avoir un polymorphisme des caractères végétatifs où les feuilles bipennées caractéristiques des légumineuses se transforment plus ou moins progressivement en phyllodes. Les modalités d'acquisition du phyllode figurent parmi les critères utilisés pour la taxonomie et la phylogénie du genre. Cependant, ces descriptions sont souvent qualitatives, et aucune méthode générique n'est proposée pour analyser et quantifier l'évolution nœud à nœud de la forme des feuilles le long d'un axe.
Plusieurs études, menées sur des espèces fruitières (pommier, abricotier, kiwi...) et forestières (chênes, cyprès, hêtres...), ont permis d'établir un contexte méthodologique statistique adapté à l'analyse de séquences d'événements botaniques pour analyser les processus de croissance, de ramification et de floraison. En s'appuyant sur cette littérature, l'objectif de ce travail est d'évaluer la pertinence de modèles tels que des semi-chaînes de Markov cachées pour caractériser et quantifier l'évolution nœud à nœud des formes de transition chez l'Accacia mangium, depuis les première feuilles jusqu'à la mise en place de phyllodes dans les premiers stades suivant la germination.
Cent soixante-treize plantules de moins de trois mois ont été étudiées au sein d'une régénération naturelle dans la région de Lampung nord, au sud de Sumatra, en Indonésie. Huit types foliaires ont été définis sur la base du nombre de folioles présents et de la largeur du pétiole. Les axes ont été décrits nœuds à nœud sous forme de séquences, et la variable mesurée était le type foliaire tel que défini précédemment. L'évolution du type foliaire est modélisée par une semi-chaîne de Markov cachée à huit états. Les principales propriétés des séquences, telles que le nombre de nœuds avant la première occurrence d'un type foliaire ou bien le nombre de nœuds successifs portant un type foliaire donné, sont restituées correctement par ce modèle. L'analyse met en évidence une séquence précise et ordonnée de types foliaires avant la mise en place des premiers phyllodes. La forme foliaire de transition définie par la présence de deux folioles avec un pétiole de largeur supérieure à 0,5 cm est facultative et très peu représentée.
Sur un second jeu de données de plantules âgées de six mois et ne portant que des phyllodes (les formes foliaires de transition étant tombées), nous mettons en évidence un changement de la phyllotaxie, depuis une phyllotaxie alterne spiralée d'indice 2/5 vers une phyllotaxie alterne spiralée d'indice 3/8. La distribution du rang du nœud où intervient ce changement est similaire à la celle du nœud où s'exprime le premier phyllode mis en place (entre le range 8 et le rang 16) obtenu sur le premier jeu de donnée. Ceci soutiendrait l'hypothèse d'un changement conjoint de la phyllotaxie et de l'acquisition du phyllode.
Le résultat majeur de ce travail est la démonstration de la pertinence de l'utilisation de modèles stochastiques markoviens pour caractériser et quantifier l'évolution de la forme foliaire chez l'Accacia. De nombreuses méthodes de comparaison de séquences ou de modèles Markoviens étant disponibles, ce travail – préalable nécessaire – ouvre de nouvelles perspectives pour tester la pertinence de la prise en compte des modalités d'expression de l'hétéroblastie pour souligner des groupes taxonomiques et définir les principales tendances de la différenciation évolutive des feuilles.
1 Introduction
Acacia is the second largest genus in the family Leguminosae, with about 1350 species [1]. The genus is divided into three subgenera: subg. Acacia, subg. Aculeiferum, and subg. Phyllodineae [2] (or Acacia subg. Acacia, subg. Senegalia and subg. Racosperma [3], which are synonymous to Vassal's three subgenera). The subgenera Phyllodineae, which forms a huge group of around 960 spp. mainly distributed in Australia, was the first described by Wildenow [4] and de Candolle [5], as the species are characterised by a polymorphism of vegetative characters where bi-pinnate leaves are replaced by a type of foliar organ called a phyllode [6]. Phyllodes are morphologically distinct from the ordinary foliage leaf [6,7]. Phyllodes are flattened along a vertical plane and are highly xeromorphic in structure, whereas the usual type of photosynthetic organ in the genus Acacia is a once-pinnate and bi-pinnate leaf composed of a variable number of leaflets [6].
The number of nerves in the phyllode is not a useful taxonomic character for sectional classification [8], whereas the ontogeny of the leaf in the genus Acacia provides useful criteria for taxonomy and phylogeny [9]. The study of the morphological stages of the primordial leaves of seedlings and of ontogenetic sequences helps the development of adult foliar types to be understood, highlights natural taxonomic groups, and defines the main trends in the evolutional differentiation of the leaf [9,10].
The difficulty when studying the Acacia species is the great diversity in the polymorphism of vegetative characters [10]. In 1967, Vassal [10] noted major, specific variations in the succession of foliar types for Acacia caven, but also noted that there was a ‘constant’. Indeed, he observed that some frequent variations reappear in several A. caven seedlings (such as the third tri-pinnate leaf). Thus, one possible solution would be to examine the ontogenic sequence from a number of seedlings big enough to find the most frequent variations in the rarer ones [11].
The ordered and indexed succession of the values taken into consideration vis-à-vis qualitative variables such as leaf shape along the axes corresponds to a “sequence of botanical events” [12]. Statistical methods exist that can be used to analyse sequences of events, ranging from exploratory analysis to model building. Markovian models have proven to be particularly pertinent in modelling the sequence of events [12,13]. In the case of leaf shape evolution, in which homogeneous observations can be made for successive zones, hidden semi-Markov chains are the models that best reveal the ontogenetic sequence of a given species [12,14].
As a means of better understanding how leaf shapes along the axes of seedlings change prior to phyllode acquisition, we used hidden semi-Markov chain models to study Acacia mangium Willd. seedlings where the different transitional forms range from pinnate leaves to phyllodes [6,7,15]. The aims of this study were (i) to define and quantify the different transitional forms in individuals as a way to characterise the average ontogenetic sequence in populations, (ii) to characterise the modalities of development from the pinnate leaf to the phyllode, and (iii) to define the link with changes in the phyllotactical pattern in A. mangium seedlings. This paper describes the ontogenetic sequence of leaf development in A. mangium seedlings as a means of discussing the concepts of juvenility and heteroblasty, as well as their potential applications for phylogeny.
2 Materials and methods
2.1 General information and study sites
Native of Australia, Papua New Guinea, and eastern Indonesia, Acacia mangium (Willd.), which is a pioneer species, has been planted in other tropical and sub-tropical regions as part of reforestation programmes. This fast-growing species, capable of colonizing damaged sites, is used in soil erosion control to avoid the spread of Imperata cylindrica (L.) weed grasses and to re-establish soil fertility [16]. A. mangium is a commercially important tree used as raw material by the pulp industry thanks to its high yield and high-quality fibre [17].
The Acacia mangium seedlings studied belong to a naturally regenerated stand from Perum Perhutani (state company managing forests and forest plantations in Indonesia) in the North Lampung area, 04°32′41.7″S, 104°57′04.8″E, (South Sumatra, Indonesia), in an area that was burned by a fire. The trees originated from Papua New Guinea. In this area, the soils are very deep, well drained, and extremely acidic, with low soil fertility. Elevation is around 60 m above sea level. The mean annual temperature is 28 °C, but varies between 22–33 °C. Annual rainfall averages between 2200–2500 mm over two main seasons: the rainy season, from November to May, and the dry season, from June to October.
2.2 Plant material and study protocol
A first sample of 173 Acacia mangium seedlings of different ages, from germination to three months old, were collected in the field, beginning in September 2004 and subsequently stored in a cold box for later observations and leaf measurements. All samples were seedlings during the same dry season and found close together (in an area around 20 m2) as a way to limit the influence of the weather and soil characteristics on growth.
The first stage of our study was to establish a complete repertoire of foliar types; a total of eight different leaf types were identified. As a means of quantifying changes in leaf shape, the number of leaflets and the widths of the petiole were recorded. The following coding was chosen: (0) leaf scar; (1) cotyledon; (2) pinnate leaves where (3) and (4) the petiole is not developed and where there are two and four leaflets per leaf, respectively; (5) the petiole is developed with a width inferior to 0.5 cm and there are four leaflets per leaf; (6) and (7) the petiole is developed with a width superior to 0.5 cm and there are four and two leaflets per leaf respectively; and, finally, (8) phyllode (Fig. 1). No other transitional forms were observed in this species.
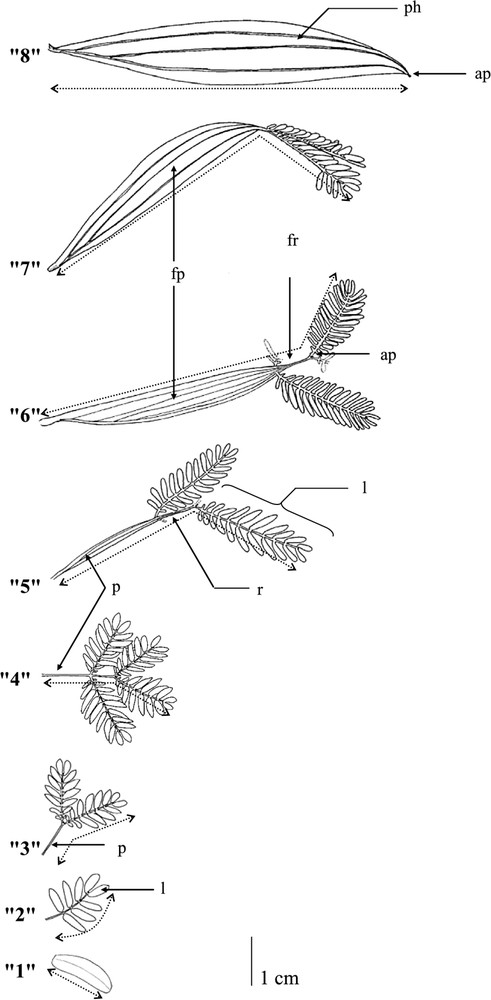
Different transitional-form leaves from the simple cotyledon and the pinnate leaf to the phyllode in Acacia mangium Willd. seedlings: ap = apical pointlet, fp = flattened petiole, fr = flattened rachis, l = leaflet, p = petiole, ph = phyllode, and r = rachis.
All seedlings, characterised by an average of nodes, were described node by node from the base to the tip by (i) recording the leaf form; among these seedlings, 45 individuals bearing the greatest number of leaves were chosen, and we recorded (ii) the length of the leaf and (iii) the number of leaflets. Leaf lengths were measured from the base of the leaf to the terminal part of the leaflet, as shown in Fig. 1.
One of our objectives was to determine if there is a correlation between changes in the phyllotactical pattern and the structure of the phyllode. The seedlings studied permitted us to observe the evolution of leaf shapes, but they did not bear enough nodes to enable us to define at which node changes in the phyllotactical fraction appear. A second sample of 40 six-month-old seedlings was then necessary to determine the phyllotactical arrangement. In this sample, all first foliar organs had fallen off, so that it was not possible to make a hypothesis about their types. All seedlings from this second sample started to grow in March, during the rainy season; they were harvested at the beginning of September 2004, during the second part of the dry season.
2.3 Methods of analysis
The quantitative measurements of the different transitional forms were studied and statistical analyses conducted using ‘R’ software (http://www.r-project.org/). Means and standard deviations for the different transitional forms, including length and number of leaflets, were compared using Student's t-test at the 95% threshold [18].
The leaf shape sequences observed in A. mangium may be viewed as a succession of homogeneous zones or segments, in which composition properties (in terms of proportion of leaf shapes) do not change substantially within each zone, but between zones. The composition of each zone may be defined by a single type of leaf shape, but they can also combine different types.
The selected statistical model is called the hidden semi-Markov chain, and its structure very directly reflects the structuring of the axillary sequences (for more details, see [12,19]). The first level represents both the succession of zones and the length of each zone. Each zone is represented by a mathematical object, called a state. The different states are connected by transitions with associated probabilities that sum to one for the transitions leaving a given state (probabilities of moving from state i at rank to state j at rank n). Initial probabilities are also needed to select the initial states (probabilities of being in a given state at the beginning of the sequence). An occupancy distribution, which represents the length of the corresponding zone in terms of number of nodes, is associated with each state, except for the final, absorbing state. The particular case of the final absorbing state (a state such that after entering it, the process remains there forever) requires a particular convention, since the time spent in this state (which is infinite) cannot be represented by an explicit state occupancy distribution (this requirement for the correct specification of semi-Markov chains is discussed in [20]). A second level consists in associating with each state a discrete distribution representing the leaf shapes observed within the zone.
We used the following convention to represent the estimated hidden semi-Markov chains: each state is represented by a box, which is labelled with an upper-case letter in its lower right-hand corner. Boxes representing transient states have a single-line border, while those corresponding to the final absorbing state have a double-line border. The possible transitions between states are represented by arcs, with their probabilities noted alongside. Thick arcs entering states indicate initial states. The attached initial probabilities are noted nearby. The occupancy distributions are shown above the corresponding boxes. The possible leaf shapes observed in each zone are indicated inside the boxes, the font sizes being roughly proportional to the observation probabilities.
The frequency distribution of a numerical variable can be characterized by measures of location (mean, median, mode), dispersion (variance, standard deviation, mean absolute deviation) or shape (coefficient of skewness, kurtosis coefficient). The characteristics of a sample of sequences are not numerical values such as for a frequency distribution, but take the form of families of frequency distributions [12]. These characteristics are divided into the three following categories: ‘Intensity’ is the empirical event distribution extracted for each successive node rank from a sample of sequences; ‘Interval’ with (i) time up to the first occurrence of an event (or first passage of time during an event; i.e., the number of transitions (or internodes) before the first occurrence of this event); (ii) recurrence time (i.e., the number of internodes starting from a given event until its subsequent occurrence); (iii) sojourn time (i.e., ‘running length’ of an event or the number of successive occurrences of a given event); and ‘Counts’ or the number of occurrences of a given pattern that are extracted for each sequence.
Families of characteristic distributions are useful for the exploratory analysis of the structure of the sequences (for example, intensity provides an overview of process ‘dynamics’). The accuracy of the estimated model is also mainly evaluated by the way in which the characteristic distributions computed from model parameters fit with the corresponding empirical characteristic distributions extracted from the observed sequences (see [12]). Statistical analyses of the sequences were calculated using Amapmod software [21].1
3 Results
3.1 The morphology of transitional leaf forms
A pair of simple cotyledons is followed by a heteroblastic sequence before the initiation of a simple phyllode encoded ‘8’ (Fig. 1). The sequence is constituted by a once-pinnate leaf encoded ‘2’ (Fig. 1), and then a succession of different bi-pinnate leaves encoded ‘3’, ‘4’, ‘5’, ‘6’, and ‘7’, as shown in Fig. 1.
The total length of each transitional form increases significantly with leaf length according to the heteroblastic sequence from leaf shape ‘2’ to leaf shape ‘6’ (Fig. 2A). At the same time, the average number of leaflets increases significantly from leaf shape ‘2’ to leaf shape ‘6’ (Fig. 2B).

Box plots of the total leaf length (A) and the total number of leaflets (B) for the different leaf shapes in Acacia mangium seedlings. The upper and lower borders of the boxes are the 75th and 25th percentiles, respectively; the black horizontal lines within the boxes are medians and the error bars are the 10th and 90th percentiles. a, b, c, d, e, f and g: comparison using Student's t-test; a change in the letter between two boxes on the plot indicates that the difference is significant at the 95% threshold.
3.2 Developmental modalities of transitional leaf forms
The model estimated from the leaf shape sequences is composed of seven successive transient states and a final absorbing state (Fig. 3). The states are principally defined by a single type of leaf shape, possibly combined with 0 (absence of leaves) due to the natural self-pruning of leaves. Only state F is defined by a true mixture of leaf shape types with ‘6’ (leaves with developed petiole/rachis and a 4-pinna bi-pinnate leaf) and ‘7’ (leaves with a developed petiole and a 2-pinna bi-pinnate leaf).

Modelling of the transitional-form leaves in Acacia mangium seedlings using a hidden semi-Markov chain. Each state, indicated by a letter and corresponding to a leaf shape, is represented by a circle. Transient states are surrounded by a single line, while the final state is surrounded by a double line. The possible transitions between states are represented by arcs with the attached probabilities noted nearby (for legibility, only transitions with probabilities exceeding or equal to 0.05 are shown). The thick, blank arrow entering states indicates initial state. The related initial probabilities are noted nearby. The observation probabilities are indicated for each state and each leaf shape, which are indicated by a number. The occupancy distributions are shown at the right side of each corresponding state.
States A, B, C and D are linked together with a transient probability equal or close to 1 (; ). The initial state A corresponds to the cotyledons that occur in the first node. All had fallen off by the time of the observations (probability for ). State B is also defined by a sojourn time of one node and defined by leaves with one-time-compound leaves (type ‘2’), of which 11% are still present (connected to the axis). State C concerns 4.8 nodes on average and is defined by a bi-pinnate leaf (type ‘3’), of which only 18% are still on the axis. State D is characterised by an occupancy distribution of 2.1 nodes on average and is defined by a 4-pinna bi-pinnate leaf (type ‘4’), of which 64% are still on the axis. From state D, the most frequent transition concerns the sequence of states E, F and H (, , ). State E concerns 1.7 nodes on average and is defined by a 4-pinna bi-pinnate leaf and a developed petiole with a width greater than 0.5 cm (type ‘5’). State F concerns 1.3 nodes on average and is defined by a combination of a well-developed petiole (>0.5 cm), a 4-pinna bi-pinnate leaf (type ‘6’, 85%) and a 2-pinna bi-pinnate leaf (type ‘7’, 15%). The final absorbing state H is defined by the phyllode (type ‘8’); once the phyllode has begun to develop, the process cannot be reversed. From state E, it is possible to jump directly to the final absorbing state H, with a probability of . State G is accessible from states D, E and F with the respective probabilities of , , , and always progresses toward the final absorbing state H (). State G concerns 1.2 nodes on average; it is defined by a 2-pinna bi-pinnate leaf and a well-developed petiole (type ‘7’).
The accuracy of the entire model was evaluated by comparing the intensity of the theoretical characteristics with the corresponding characteristics extracted from the observed sequences (Fig. 4). Theoretical and observed data had very similar profiles with the successive presence of the transitional forms before the initiation of the phyllode. Phyllodes generally develop on average at the 11th node from the base of the seedling's axis (Fig. 5A). The development of the first phyllode occurs from node 8 to node 16, depending on the seedling.
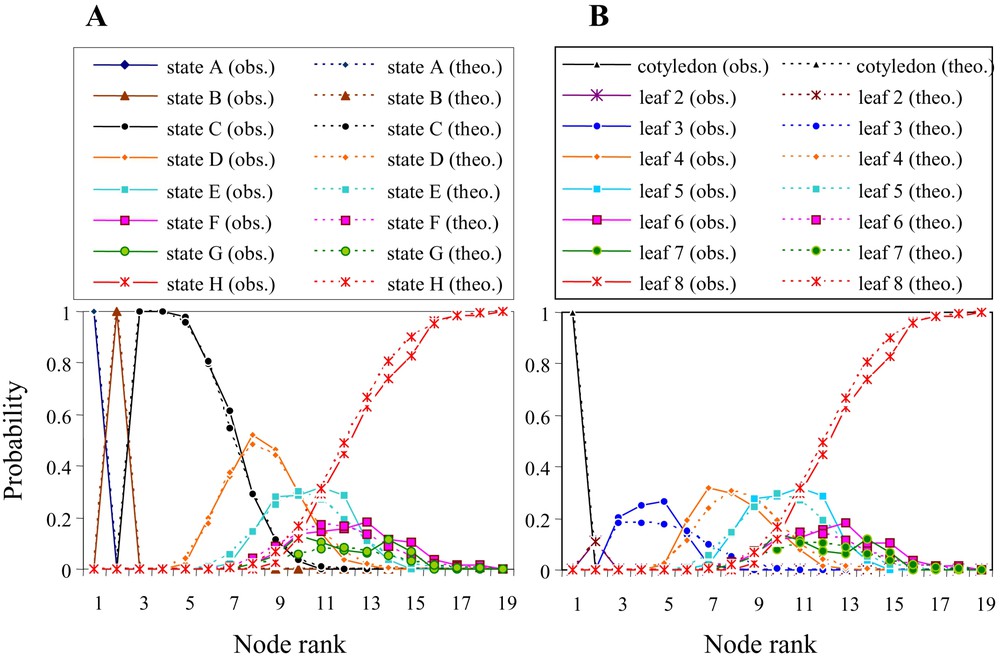
Probability of the different types of leaf shapes in relationship to node rank (intensity) from (A) the observed sequences and (B) the theory computed based on the model's parameters.

(A) Time until the first phyllode develops and (B) node rank of phyllotactical fraction modification from 2/5 to 3/8.
3.3 Phyllotactical pattern
The cotyledons on seedlings in the cotyledonous stage are paired. Young seedlings with transitional-form leaves show a spiral phyllotactical fraction of 2/5. Phyllodes on 6-month-old seedlings are initiated with a spiral phyllotactical fraction of 3/8. The phyllotactical arrangement of leaves changes from node 8 to node 22 or, on average, at node 14 (Fig. 5B).
4 Discussion
Analysing the succession of leaf shapes among A. mangium seedlings as discrete sequences highlighted the existence of successive zones. A hidden semi-Markov chain confirmed that the shape of the leaves depends on their location along the seedling's stem. The average successive leaf shapes correspond to the ontogenetic sequence , which is considered, as defined by Goebel [22], to be a heteroblastic development. The transitional form encoded ‘7’ (petiole developed with two leaflets per bi-pinnate leaf) is very slightly represented. The apical meristem has to go systematically through the ontogenic sequence from the transitional form ‘2’ to the transitional one ‘5’ before being able to produce a phyllode. The sojourn time in each transitional form is between one and two nodes, except for the transitional form ‘3’ with an average of 4.8 nodes. This higher sojourn time concerns the bi-pinnate leaf which constitutes, according to Vassal [10], the common foliar morphotype throughout the genus Acacia. Phyllodisation takes place through the gradual modification of the petiole-lamina region of pinnate leaves and changes in symmetry from a horizontal to a vertical position [6]. In some other Acacia species, such as A. urophylla, phyllodisation can be abrupt without transitional forms [10].
Vegetal maturation is very often marked by a progressive change in leaf shape, especially for the ‘heteroblastic’ species such as Hedera helix [23], Eucalyptus sp. [24], and Artocarpus elasticus [25], where the shape of the lamina develops in an obvious way or for Acacia species with phyllodes [6,7,26]. Foliar transformation takes place very quickly in A. mangium, since the meristem gives rise to the phyllodes from the ninth to the seventieth node from the cotyledon. However, Vassal [10] described Acacia seedlings where phyllodisation is specifically precocious – like for A. diaphyllodinea (first leaf after the cotyledon), A. confusa (second leaf after the cotyledon), A. peuce and A. grasbyi (third and fourth leaf after the cotyledon) – and could go unnoticed. In Kaplan's study [6], phyllodes can first appear from nodes 3–6 for A. longifolia, from nodes 5–9 for A. pravissima, and from nodes 4–10 for A. verticillata. In A. melanoxylon [27] and A. rubida [28], the evolution of the foliar organ during tree development is very progressive, with juvenile, transitional and adult leaves all visible on this small tree crown. Contrary to A. mangium, juvenile and adult leaves coexist on flowering A. melanoxylon axes, making the distinction between juvenile and adult phases difficult [27]. In other species, such as Rhaphidophora korthalsii [29], a single individual can have both juvenile and adult leaves alternating regularly along the axis. However, in A. mangium, once the phyllode is formed, it remains throughout the life of the tree and represents the mature foliar organ. That is to say, there is no spontaneous reversion to juvenile transitional-form leaves in A. mangium, as can be observed in other species [30].
It is interesting to note that the modification of the spiral phyllotactical fraction from 2/5 to 3/8 seems to occur at the same node rank of phyllode acquisition. This modification in the phyllotactical pattern can be observed in older seedlings that have enough nodes to enable the fraction to be calculated. However, we must be careful in correlating changes in the phyllotactical arrangement with phyllode acquisition, as the study was conducted on two distinct seedling samples that grew during different seasons. Indeed, we do not know whether these two processes are dependent or not on the environment. This question should be investigated by monitoring growth.
However, a similar modification in the phyllotactical pattern from a spiral pinnate leaf to a whorled phyllotaxical phyllode was also described in Acacia verticillata by Rutishauser [31]. In other species, like Hedera helix, the change in leaf shape comes with the modification of numerous internal potentialities, such as the ability to produce adventitious roots or rooted cuttings [23,32]. In Sequoiadendron giganteum, juvenile leaf type axes tend to be more easily propagated by rooted cuttings than mature leaf type axes [33]. A study of A. mangium showed that mature (i.e., in the state of phyllodisation) seedlings have a much lower potential to produce adventitious roots and thus to be propagated by rooted cuttings [15]. Differences in leaf morphology can also be associated with differences in the ability to produce adventitious roots [34], which is information of great interest to reforestation programs, especially those concerning A. mangium.
The evolution of phyllodes in Acacia is widely believed to be an adaptation to drought conditions [35,36]. According to Vassal [9], phyllodes seem to be the result of a kind of ‘evolutional explosion’ produced by a primordial speciation in response to considerable adaptive radiation. For these different reasons, the same author considers that the phyllode is evolved and that the bi-pinnate leaf is primitive. The bi-pinnate leaf, absent from the adult tree, corresponds to an ancestral foliar type. The degree of the relative evolution of the Acacia species where the ontogenetic sequence is condensed is, according to Vassal [9], more advanced than a longer ontogenetic sequence.
5 Conclusion
The use of a hidden semi-Markov chain to analyze plant structure or highlight the successive ontogenic phases of development has proven to be very useful for a very large range of plants. In this study, the use of this method permitted us to quantify leaf shape change and phyllode acquisition during ontogeny. However, the endogenous nature of the gradient expressed in this naturally regenerated stand should be carefully interpreted. Indeed, without any certitude about the age of the seedlings and the characterisation of their individual microenvironments, it is difficult to separate environmental effects from ontogenetical ones. However, the methodology used opens the door to a better understanding of the plasticity of this species based on its environment, but also allow its development to be compared to that of other Acacia species. Methods for comparing sequences or models exist [20] and could be used advantageously to highlight natural taxonomic groups and to define the main trends in the evolutional differentiation of the leaf in various phyllodineous Acacia species. We have also highlighted here a modification in the spiral phyllotactical fraction that seems to occur during phyllode acquisition. As a means of confirming this assumption and as a follow-up to this study, it would be interesting to eliminate the effect of the environment by monitoring changes in phyllotaxy in correlation with changes in leaf types as the plants grow.
Acknowledgements
The authors would like to thank Novi Sari Wahyuni for her help in the field. This research was carried out as part of the SAFODS (Smallholder AgroForestry Options for Degraded Soils) collaborative research project, partly funded by the EU: contract number ICA4-CT-2001-10092. AMAP (Botany and Computational Plant Architecture) is a joint research unit which associates CIRAD (UMR51), CNRS (UMR5120), INRA (UMR931), IRD (R123), and Montpellier 2 University (UM27); http://amap.cirad.fr/. The supports are gratefully acknowledged. We would also like to thank Yves Caraglio and Daniel Barthélémy for their relevant comments and Andrea Dejean for proofreading the English version of the manuscript.