Since their emergence in the 1980s, developmental genetics have mainly relied on the study of a handful of model organisms, Drosophila and C. elegans in protostomes, the mouse, chick, xenopus and zebrafish in deuterostomes. The focus on these sole species has clearly led to major advances in our understanding of the complex molecular and cellular mechanisms that gradually shape the embryo during ontogeny. However, as comparative data accumulate, limitations inherent to this strategy become more and more obvious. A major caveat is related to the absence of a systematic assessment of the evolutionary conservation of the mechanisms characterized in model organisms. This results in frequent overgeneralizations, that tend to consider processes identified in a single species as general to a whole taxon, in the absence of any explicit argument. Another one is that as any extant species, model organisms have independently diverged from ancestral patterns, each along his lineage. This divergence process can considerably obscure the relationships between the developmental processes characterized in each model organism. In the absence of unambiguous morphological conservation, comparisons of molecular patterns or interactions have opened new possibilities to alleviate this difficulty. However, the frequent recruitments of gene regulatory networks and signaling pathways, now known to repeatedly occur during evolution, make conclusions based on this sole criteria extremely hazardous. Comparisons at the broad evolutionary scale, such as those between Drosophila and vertebrates, are obviously faced with these limitations. But the same kind of difficulty can arise in comparisons between much more closely related species, as exemplified by the mechanisms of early axis specification, which apparently substantially diverge across vertebrates. This review first aims at an overview of the main characteristics of this process in vertebrate model organisms. We then show how an evo-devo approach, relying on the detailed molecular characterization of a chondrichthyan, the dogfish Scyliorhinus canicula, provides insight into the underlying unity of these apparently diverse mechanisms.
1 The genetic control of axis specification in the vertebrates: a difficult synthesis
In all vertebrates, the embryonic axis proper, from head to tail, is laid down during gastrulation. As in all bilaterians, this axis is endowed with two polarities, antero-posterior and dorso-ventral (left-right asymmetries appearing later, at advanced stages of gastrulation). However, bilaterality is established at much earlier stages of development. A first polarized axis (animal/vegetal), conserved across vertebrates, is thus inherited from the oocyte, while the second one results from a radial symmetry breaking event, which may involve different mechanisms depending on the species considered. Deciphering the mechanisms of axis specification in vertebrates thus implies answering two main questions: Which are the molecular mechanisms responsible for the establishment of the earliest polarities observed? And second, which is the link between these polarities and those of the embryo proper? Studies conducted in amphibians or teleosts on the one hand, and in amniotes on the other hand, lead to substantially different views.
In xenopus, which has long been the vertebrate reference for this process, radial symmetry is broken upon fertilization. Sperm entry thus triggers a microtubule-directed movement, which results in the displacement of vegetal maternal determinants towards the opposite pole of the egg [1]. At this location, these determinants initiate a succession of inductive events, ultimately leading to Spemann organizer formation. The canonical Wnt signaling pathway is central in this process, even though other signals may also be involved [2]. The organizer/ab-organizer axis thus formed is traditionally termed dorso-ventral, both because of the dorsalizing effects mediated by the organizer during gastrulation and the early activation of ventralizing BMP signals acting at the ab-organizer side of the embryo at blastula stages. Related mechanisms operate in the zebrafish. Even though the role of fertilization is unclear, a dorso-ventral, or organizer/ab-organizer axis is established as early as the two-cell stage, through a microtubule dependent process similarly involving the relocation of vegetal maternal factors [3]. As in xenopus, canonical Wnt signals are key players in the specification of the dorsal pole [4], which also involves the Nodal signaling pathway [5]. The mechanisms of axis specification in amniotes differ from the former on several important aspects. First, there is no overt homologue of the dorso-ventral axis in these species at blastula stages. Symmetry is broken substantially later than in amphibians and teleosts (blastocyst stage in the mouse) and the relationships of the underlying mechanisms with those operating in amphibians and teleosts remain unclear. Nomenclatures reflect these uncertainties, since in the mouse, the earliest asymmetries are generally correlated with the establishment of the later antero-posterior (instead of dorso-ventral) polarity, as defined by its relationship to the future head to tail orientation of the embryo proper. Another clear difference is that while the site of embryonic axis formation is already fixed at cleavage stages in amphibians and teleosts, it retains a considerable plasticity in amniotes until blastula stages. The chick blastoderm can thus be partitioned in several pieces, all of which are able to generate a full-length embryonic axis until blastula stages [6]. Similarly, in the mouse, no evidence for a regional specification of the epiblast, from which the embryo proper derives, can be observed prior to the onset of gastrulation. The last specificity of amniotes is the presence and early segregation of extra-embryonic tissues, such as, in the mouse, the visceral endoderm (with its derivatives, ExVE, EmVE, AVE, see Fig. 4 for abbreviations), and extra-embryonic ectoderm (ExE), respectively derived from the primitive endoderm and trophectoderm. These tissues, themselves involved in complex reciprocal interactions, play crucial roles in patterning the embryo along its rostro-caudal axis [7–9]. However, their phylogenetic origin as well as the conservation of the mechanisms controlling their specification and relative expansion remain unclear. Except for the anterior visceral endoderm (AVE), a derivative of the embryonic visceral endoderm (EmVE, Fig. 4), the presence of functionally equivalent territories outside amniotes also remains a totally open question. One interpretation of these differences is that the emergence and diversification of extra-embryonic tissues in amniotes may have paralleled the rise of novel mechanisms of anterior–posterior axis specification in the taxon. However, it is equally possible that amphibians and actinopterygians may have undergone convergent simplifications of more complex ancestral mechanisms or that these mechanisms may have been simply overlooked thus far in these fast developing species. Resolving this issue is important to obtain a comprehensive view of axis specification mechanisms in vertebrates and to gain insight into the diversifications that have taken place during early development in the main osteichthyan taxa.
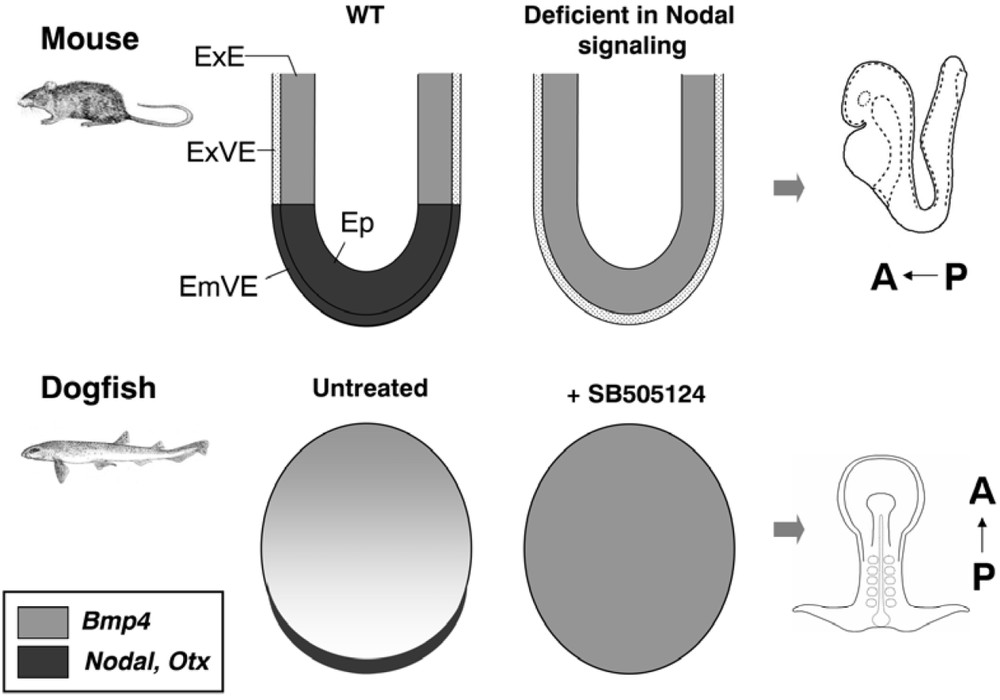
Similarities of the phenotypes induced by early inhibitions of Nodal signaling in the mouse and dogfish. In the mouse, an expansion of the Bmp4 expressing extra-embryonic ectoderm and defective specification of the Otx2 positive embryonic visceral endoderm are respectively observed in Smad2-/-; Smad3-/- and Nodal-/- mutant mouse embryos, both defective in Nodal signaling. These defects are reminiscent of those observed in dogfish embryos treated with SB505124, an inhibitor of Nodal/activin signaling (expansion of the Bmp4 positive extra-embryonic ectoderm concomitant with an extinction Nodal and Otx5 at the posterior margin, proposed to be evolutionarily related to the mouse EmVE). Abbreviations used: ExVE, extra-embryonic visceral endoderm; ExE, extra-embryonic ectoderm; Ep, epiblast; EmVE, embryonic visceral endoderm.
2 An evo-devo strategy: the dogfish as a chondrichthyan model
Different strategies can help to better understand the relationships between vertebrate model organisms during early development. The developmental genetics approach, which currently prevails, aims at an exhaustive characterization of the genetic mechanisms involved in each model. In this view, the difficulties encountered in comparisons are mainly linked to gaps in our knowledge, which preclude the identification of selectively conserved regulatory “kernels” within broader, possibly extensively diversified, gene regulatory networks [10]. However, one difficulty of this approach is to assess the evolutionary status of apparently conserved regulatory modules, which can reflect true homology of mechanisms and processes but can also result from co-options. In view of the complexity of the interactions involved during early development and of the multiple roles fulfilled by key players in this process, conclusions can be far from straightforward in the absence of robust comparative analyses. These considerations led us to use an original evo-devo approach, aimed at reconstructing the gnathostome ancestral state. Its rationale is that the link between model organisms is related to their common heritage of ancestral characteristics, a notion often overlooked by developmental genetics. A cautious reconstruction of the ancestral state can therefore help to unravel both the underlying unity of apparent highly divergent mechanisms and the diversifications characterizing each model organism. The general evo-devo strategy for such a reconstruction relies on the use of an outgroup. This in no way understates that such a group should be considered more primitive than any other but simply that in the most parsimonious interpretation, the occurrence of a given character in two distinct taxa implies its presence in its last common ancestor. Chondrichthyans appeared as an ideal outgroup for such a systematic comparative approach with each of the vertebrate model organisms. Their position, as the closest outgroup of osteichthyans, ensured robust morphological comparisons, difficult to obtain with more distant taxa such as ascidians or cephalochordates. The same argument is relevant from a molecular standpoint. It is currently clear that the gene duplications that have occurred early in the vertebrate lineage have predated the gnathostome radiation and no evidence for additional tetraploïdisations, such as those that have occurred in actinopterygians, has been obtained in chondrichthyans [11,12]. The gene contents of the genomes of chondrichthyans and osteichthyans are thus readily comparable and orthology relationships generally relatively easily resolved. Inside chondrichthyans, we chose to focus on the small spotted dogfish Scyliorhinus canicula. Two main criteria guided this choice. First, this is a common species on our coasts. Eggs can be easily collected, at very early stages (segmentation) and kept in sea aquariums until hatching. The dogfish is also a traditional model in classic comparative embryology and its development has been the focus of a number of detailed histological studies performed more than one century ago. It became rapidly clear that in situ hybridization and immuno-histochemistry protocols could be readily adapted, with excellent results.
To develop this model organism, our first objective was to obtain large-scale databases of embryonic ESTs. We thus constructed several cDNA libraries at selected embryonic stages (blastula to gastrula; early organogenesis; pre-hatching), which were subjected to systematic sequencing in collaboration with the Centre national de séquençage (Evry). With 250 000 available ESTs and arrayed recombinants, we now dispose of a unique collection of probes in a chondrichthyan. Another important aspect was to develop appropriate breeding facilities for adult dogfish in order to amplify and stabilize egg production. This optimization was successfully taken in charge by the Station biologique de Roscoff, now producing more than 10 000 eggs per year, which opens the way to large-scale molecular characterizations. The last challenge is now to develop functional approaches. While an important step will be to control in vitro conditions of embryo culture, in ovo pharmacological treatments using a wide range of well characterized antagonists of the major signaling pathways are already amenable (Coolen and Menuet, unpublished results). Together with the molecular and biological resources now available, this obviously opens important new perspectives for the model.
3 The dogfish view: unexpected similarities with amniotes
3.1 A highly interpretable mode of axis construction
In order to gain insight into the relationships between early development in the dogfish and the traditional vertebrate model organisms, we performed a detailed molecular characterization, from blastula to gastrula stages. The dogfish egg is telolecithal and as in the chick, the embryo develops as a large flat blastoderm (2–3 mm diameter at gastrulation), lying onto the surface of the yolk. The first morphological indication of an asymmetry of the disc-shaped blastoderm corresponds to the appearance of a thickening, at the posterior margin (as in the chick or the mouse, posterior referring here to the future polarity of the embryo proper). This is the site where the embryonic axis becomes visible, slightly later, at the beginning of gastrulation. Subsequent stages are marked by an elongation of this axis (stage 12–13) and the formation of a characteristic enlargement in the cephalic region (stage 14) (Fig. 1). We chose to first characterize the mode of axis formation during gastrulation, using markers of neuroectoderm and mesoderm at different antero-posterior levels [13]. This unambiguously shows that the embryonic axis extends at the level of the posterior margin from anterior to posterior, with a strict temporal regulation. At stage 12, forebrain and prechordal plate markers are thus fully established in completely segregated germ layers, while hindbrain or trunk paraxial mesoderm markers only become detectable at postero-lateral levels of the margin. In line with a pioneering description of cell movements published more than 70 years ago [14], this suggested that more and more caudal territories are specified at this location and that they converge posteriorly along the posterior margin before integrating into the extending embryonic axis. Such a tight temporal regulation of axis formation from anterior to posterior is potentially extremely interesting to accurately segregate the genetic programs involved at each level. The next step was to characterize the site of mesoderm internalization, using a whole set of mesoderm regional markers. Two conclusions emerged from this study. First, following an early and transient phase of mesoderm internalization around the whole rim of the blastoderm, the main site for this process becomes restricted to the posterior arms (Fig. 2). This result thus pointed to the posterior restriction of mesoderm formation as a common and convergent response of both chondrichthyans and amniotes to the increase of yolk [15]. In addition, the medial to lateral distribution of presumptive mesoderm along the posterior arms, as inferred by the molecular characterization, was found to precisely reflect its future regional identity in the embryonic axis [13]. The dogfish embryo therefore appeared amenable to highly straightforward interpretations, which contrasts with the relatively complicated and counter-intuitive cell movements observed in vertebrate model organisms, and particularly along the primitive streak of amniotes [16–18].
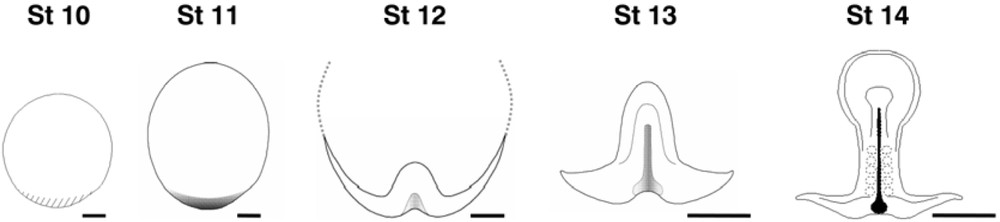
Dorsal views of dogfish embryos from pre-gastrula (stage 10) to neural plate (stage 14) stages. The site of axis formation becomes visible at the blastoderm posterior margin, as a marked thickening at stage 10 and bilayered overhang suggestive of involution cell movements at stage 11. The embryonic axis proper appears at stage 12 and extends at subsequent stages from anterior to posterior, as indicated by the transcript distribution of neuroectoderm and mesoderm regional markers.
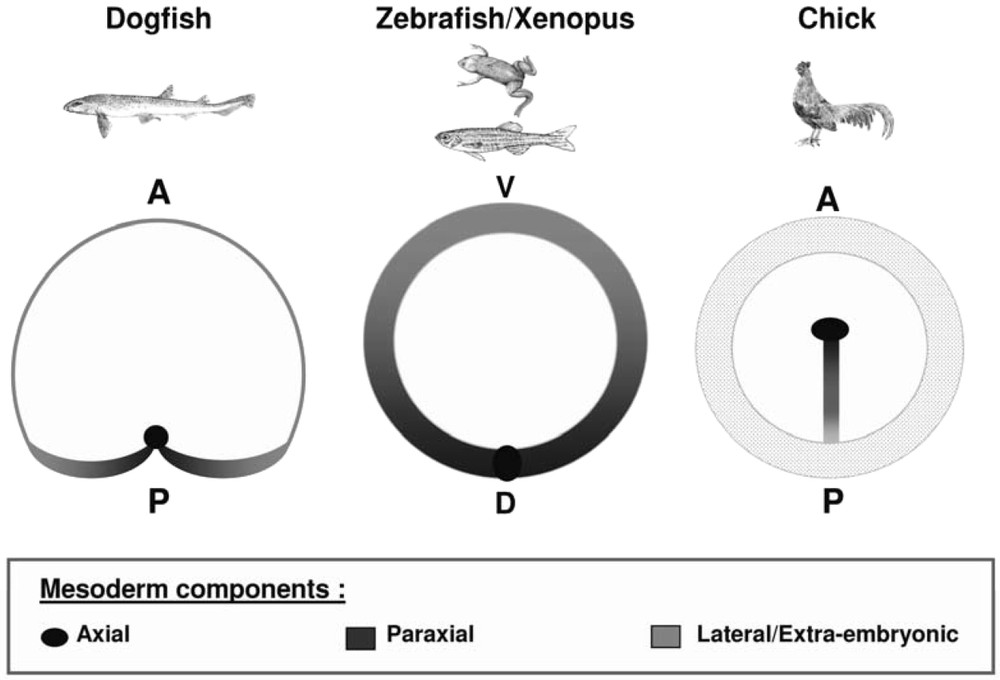
Comparisons of the site of mesoderm internalization in the dogfish, xenopus or zebrafish and chick. The sites of mesoderm internalization (blastoderm margin in the dogfish and zebrafish, blastopore lips in xenopus and primitive streak in the chick) are shown shaded in each species. The location of prospective axial, paraxial and lateral mesoderm components is shown in black, dark grey and light grey respectively. The dotted area in the chick symbolizes the area opaca. In the dogfish, the main site of mesoderm internalization is located along the posterior margin. However, the molecular characterization supports the presence of a minor prospective mesoderm cell population, contributing to extra-embryonic blood islands, along the lateral and anterior aspects of the blastoderm margin. The regionalization of the site of mesoderm internalization appears very similar in all species. However, it is most readily interpretable in the dogfish, the medial to lateral distribution of prospective mesoderm components along the posterior arms precisely reflecting their future location in the embryonic axis.
3.2 Signaling centers and molecules in the dogfish: towards a general model of AP axis specification in gnathostomes
Cell populations expressing Bmp antagonists such as Noggin and Chordin and homologous to Spemann organizer have been unambiguously identified in all vertebrate model organisms [19]. Not unexpectedly do we also identify a related cell population in the dogfish, at the level of the stage 12 notochordal triangle located in the middle of the posterior margin, where the posterior arms meet [13 and unpublished results]. In contrast, homologues of the mouse anterior visceral endoderm and the chick hypoblast, which play essential permissive roles in head formation and express Wnt and Nodal antagonists, are difficult to unambiguously identify in xenopus and zebrafish. Based on Hex and Cerberus expression, the xenopus deep and suprablastoporal endoderm and the zebrafish dorsal yolk syncitial layer have been proposed to correspond to this territory. However, functional evidence is still lacking. Furthermore, it is extremely difficult to identify a territory expressing the whole combination of markers characterized in amniotes (Hex, Lim1, Otx, Foxa2, Nodal) and clearly distinct from the early gastrula organizer and its derivatives (definitive endoderm, prechordal mesoderm). Such a territory can be unambiguously characterized in the dogfish, at the level of the stage 10–11 posterior margin. At this stage, this cell population expresses all the markers mentioned above. This early phase of Lim1 expression, which appears progressively displaced anteriorly, at a distance from the site of organizer formation, is clearly distinct from the subsequent notochordal triangle expression. Finally, different Otx paralogues are transcribed at this level and in the gastrula organizer, which definitely proves that these territories are subjected to differential genetic control. These results thus provide strong evidence for the presence of an AVE/hypoblast homologue in the common ancestor of gnathostomes. At pre-gastrula stages, the dogfish lends itself to particularly straightforward parallels with the chick owing to its flat blastoderm morphology. Further comparisons focussed on secreted signaling molecules known to play key parts in the induction of the embryonic axis highlight a very similar dynamic of gene expression at the posterior marginal zone of the latter and the posterior margin of the former. As in the chick [20,21], the onset of a transient phase of Vg1 and Wnt8 co-expression at the posterior margin of stage 9–10 dogfish embryos thus precedes the induction of a prominent Nodal expression phase spanning most of the blastoderm periphery, except for a limited territory at the level of the candidate early gastrula organizer. This Nodal expression domain is itself reminiscent of the proximal epiblast or early primitive streak expression territories observed in the mouse and the chick respectively, in line with a conservation of the well-characterized role of the gene in mesendoderm specification [22,23]. Finally, as in osteichthyans [24,25], the onset of Nodal expression is followed by an induction of the expression of Lefty, which codes for a feedback inhibitor of Nodal signaling. Taken together, these similarities support the conclusion that the genetic interactions acting at the level of the posterior margin of the dogfish blastoderm precisely reflect those known to occur at the posterior marginal zone of the chick. The most striking difference between the two species is that in the dogfish, these interactions take place at the blastoderm margin, at one pole of the bilateral symmetry axis, while in the chick, they occur inside the blastoderm, at the border between the area opaca and the area pellucida. Since Bmp4 is expressed radially in the chick area opaca [26], as well as in the mouse extra-embryonic ectoderm (ExE) [27], we used this marker in order to identify a candidate related territory in the dogfish. In this species, Bmp4 expression spans a broad territory, adjacent to, and excluding the posterior margin. As in the chick, this domain, which spreads over the yolk through epiboly movements, may primarily contribute to extra-embryonic tissues. At pre-gastrula stages, anatomical and molecular data therefore converge to support a relationship between territories located opposite to the organizer in the dogfish, but exhibiting a radial symmetry and encircling tissues contributing to the embryo proper in amniotes (Fig. 3). It is increasingly clear that in the mouse, signals secreted by the Bmp4 expressing ExE restrict the expansion of the AVE, thereby providing a mechanism for the reduction of the cephalic region in BMPRI-/- mouse embryos [9,28]. The relative location of the presumptive homologues of these tissues, located at each pole of the bilateral symmetry axis in pre-gastrula dogfish embryos, is consistent with a conservation of this interaction in chondrichthyans. Despite the phylogenetic distance between the mouse and the dogfish, available data in each of these species can thus be integrated into a coherent model of antero-posterior specification, relying on reciprocal antagonistic interactions between tissues respectively related to the AVE and ExE of the mouse prior to gastrulation and subsequent control of cell allocation to cephalic or caudal territories of the embryo proper by signals secreted by these tissues. The relative phylogenetic position of the dogfish and amniotes support the ancestrality of this two-step process in jawed vertebrates [13].
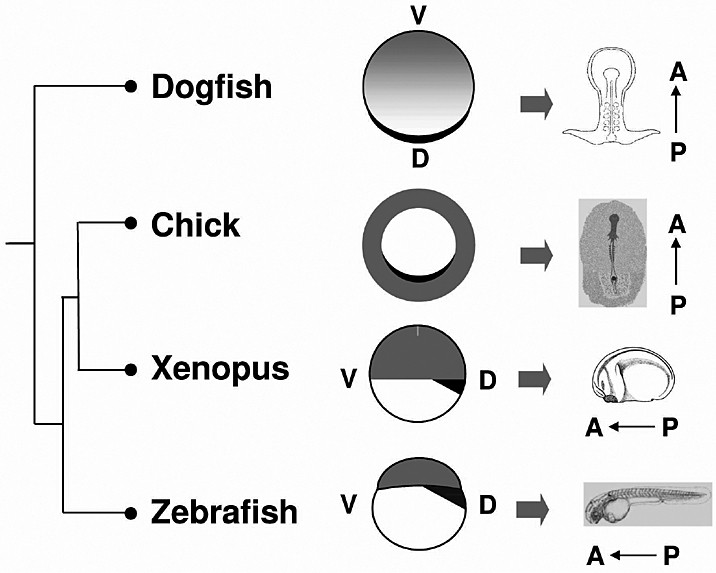
Territory equivalencies between the dogfish and vertebrate model organisms at pre-gastrula stages. Dark grey: proposed equivalents of the chick hypoblast (or mouse AVE) at the level of the posterior margin, deep or suprablastoporal endoderm and dorsal yolk syncitial layer in the dogfish, xenopus and zebrafish respectively. Light grey: Bmp4 expressing territories, corresponding to presumptive extra-embryonic ectoderm in the dogfish as in amniotes, ventral ectoderm in xenopus and zebrafish. Due to their flat blastoderm morphologies, the chick and dogfish are readily comparable. This comparison suggests a posterior expansion and radialisation of the extra-embryonic ectoderm in amniotes.
3.3 Early polarities in the dogfish: a rationale for territory comparisons between early gnathostome embryos
In order to address when a bilateral symmetry is first established in the dogfish, we extended the molecular characterization at earlier stages, predating blastocoele formation (stage 5/6). At this stage, three markers already define a polarized bilateral symmetry axis, which appears to be maintained until pre-gastrula stages. Nodal and Otx5 are thus expressed along a narrow crescent along the posterior margin (corresponding to the future organizer side), while Bmp4 expression encompasses a broad territory in the opposite, anterior moiety of the blastoderm [13]. Can the early polarity thus defined in the dogfish be related to those reported in model organisms? Based on Nodal and Bmp4 expression, a relatively straightforward relationship can be proposed with the early dorso-ventral polarity of teleosts and amphibians [19]. Comparisons are more difficult with amniotes and, in the absence of easy access to early chick embryos, are mainly restricted to the mouse. In this species, at egg cylinder stages, Nodal and the only Otx paralogue expressed during early development, Otx2, are expressed in the epiblast and overlying visceral endoderm (embryonic visceral endoderm, EmVE), while Bmp4 transcripts are restricted to the adjacent extra-embryonic ectoderm (ExE). Could the genetic interactions, which take place in the mouse along this proximo-distal, or embryonic-abembryonic axis, be conserved in the dogfish, along its early bilateral symmetry axis? Preliminary results recently obtained in the dogfish are consistent with this view. In the mouse, the specification and relative extent of the epiblast, EmVE and ExE are regulated by complex reciprocal interactions. Nodal/activin signaling play key roles in these interactions, being essential for the specification of both the epiblast and EmVE, respectively converted in ExE in Smad2-/-; Smad3-/- mutants [22] and extra-embryonic VE in Nodal-/- mutants [8]. In ovo pharmacological treatments of dogfish embryos using an inhibitor of Nodal/activin signaling, SB505124, result in a related phenotype, characterized by an absence of posterior margin specification and parallel expansion of the opposite Bmp4 expression territory (Coolen, Menuet and Mazan, unpublished results, Fig. 4). Together with our preliminary functional analysis, the molecular characterization of the dogfish is thus consistent with a relationship between the early dorso-ventral axis of amphibians and teleosts, the organizer/ab-organizer axis of the dogfish and the embryonic/extra-embryonic organization of mammals.
4 Conclusion: a comparative approach of early development in the vertebrates
The territory relationships and general model of AP specification proposed on the basis of comparisons with the dogfish suggest a strong, and as yet overlooked, underlying unity of the mechanisms controlling early development in jawed vertebrates. While congruent with current results of developmental genetics, these hypotheses should now be submitted to more systematic tests. An important issue will thus be to assess the ancestrality of the territory subdivisions (ExVE, VE, ExE, epiblast) observed in amniotes during early development and the conservation of the complex reciprocal interactions involved in their specification. Unexpectedly, the dogfish embryo may be a model of choice to address these issues, not only for his phylogenetic position as a sister group of osteichthyans, but also for the remarkable spatial and temporal resolution of expression patterns that it allows at blastula stages. These characteristics, possibly related to the protracted development and large embryo size of the dogfish, are obviously difficult to conciliate with the classic criteria for choice of model organisms (fast development, small embryo size). They may nevertheless be advantageous to address highly dynamic responses to the main signaling pathways, such as those recently reported in amniotes prior to gastrulation as well as in a number of other developmental processes (brain development or organogenesis for instance). Along with the molecular tools currently available (EST databases; protocols of pharmacological treatments; immuno-histochemical characterization of signaling activities), this may open a new era to dogfish embryology.
Acknowledgements
We are grateful to S. Paturance (UPS 44, Orléans) who optimised conditions for dogfish embryo maintenance, L. Lévèque and B. Kloareg (Station Biologique de Roscoff), who provided dogfish embryos and all former members of the group (C. Le Mentec, D. Nicolle, J.L. Plouhinec, T. Sauka-Spengler) for their contributions to the molecular characterization of the dogfish embryo. This work was supported by grants from the Groupement d'intérêt scientifique “Génomique marine”, Centre national de la recherche scientifique, Université Paris-Sud, Université d'Orléans and Région Centre.