Version abrégée française
La taxonomie des animaux présente un formidable tableau dans lequel il est difficile de percevoir un ordre. Si l’on se focalise sur les vertébrés, force est de reconnaître une sorte d’ordre, qui irait des chordés les plus simples, les céphalochordés (par exemple amphioxus), aux amniotes (par exemple un poulet), en passant par les anamniotes (par exemple une grenouille). La configuration de référence, au sens physique, pour ces animaux, est la boule ou le disque, qui sert de point de départ à leur développement. L’ovule initial est rond, et jusqu’à un nombre d’environ 20 000 cellules pour un amniote typique, la masse de cellules embryonnaire a une symétrie de révolution (ce qui ne préjuge pas d’un possible ordre interne, je ne parle ici que de la forme globale), on l’appelle morula, puis blastula. Au cours des premiers jours de développement, cette boule subit d’amples mouvements morphogénétiques, qui font apparaître des caractéristiques telles que les plis dorsaux, puis le cœur et la tête, absents des céphalochordés, puis le sac amniotique, absent des anamniotes. Ainsi, les animaux primitifs sont d’abord des sortes de tubes allongés présentant une ouverture bucale à un bout, une ouverture anale à l’autre, mais pas de tête, ni de cœur (comme amphioxus). Puis, apparaissent des animaux « plus évolués», ayant une tête et un cœur, enfin apparaissent des animaux qui se développent dans un sac, comme les oiseaux ou les mammifères. Cet ordre peut sembler parfaitement fortuit ou même le fruit d’une illusion rétrospective. Il est même difficile de justifier qu’il puisse s’agir là d’un « ordre » en un sens quelconque. Le but de cet article est précisément de montrer que l’ordre mécanique d’apparition des plans d’animaux n’est pas du tout intuitif, il s’agit de l’ordre dans lequel plie une boule, lorsqu’elle bouge. Ces plis sont organisés de façon successive, dans le sens du mouvement cellulaire. Il est bien connu en physique que les plis de surfaces contraintes se forment perpendiculairement au mouvement (flambage sous l’effet de la contrainte principale uni-axiale), comme lorsqu’on repasse une chemise. Or, la particularité du mouvement qui forme les vertébrés, est d’être hyperbolique. Ce mouvement est hyperbolique en ce que le point central a une vitesse nulle, les vitesses sont convergentes dans la direction gauche-droite, et divergentes dans la direction antéropostérieure. Ainsi, l’écoulement est d’abord lancé de façon bilatérale en direction d’un point de stagnation, puis le mouvement se poursuit par une recirculation du tissu vers l’avant et vers l’arrière, comme deux jets de tissu rentrant en collision et s’étirant le long de l’axe commun de collision. Dit simplement : le mouvement fonce d’abord de gauche à droite ce qui crée les plis du dos, puis tourne à angles droits et se poursuit le long de l’axe antéropostérieur, ce qui crée les plis de la tête et du cœur d’abord, du chorion ensuite. De ce fait, les premiers plis embryonnaires, perpendiculaires à l’écoulement, sont les plis dorsaux, et les plis suivants sont situés à l’avant et à l’arrière des deux apex, antérieur et postérieur, du pli dorsal. Les premiers de ces plis sont ventraux et forment le cœur et la poche abdominale, les seconds de ces plis sont dorsaux et se referment en iris en formant le chorion. Je montre ici que ces plis existent et adoptent cette topologie particulière, qui présente une symétrie miroir entre la partie antérieure, crâniale, et la partie postérieure, caudale. Je montre que ces plis sont bien perpendiculaires au mouvement, et qu’ils deviennent par une simple contraction, d’abord le cœur, puis le chorion. Il est particulièrement notable que le chorion forme un sac, en refermant progressivement sur eux-mêmes, comme un iris, des plis symétriques situés initialement aux deux extrémités les plus éloignées de l’embryon. Cela constitue également une preuve du caractère physique de ces plis, les forces physiques étant à longue portée dans un matériau continu. Les vitesses caractéristiques de ces phénomènes sont constantes.
Ainsi, un mécanisme simple de mouvement uniforme, formant des plis, organise successivement le dos, la tête et le cœur, puis le chorion, dans cet ordre-là, à vitesse constante. Un point remarquable est que dans ce mouvement, le cœur finit absorbé par le corps, et le corps finit lui-même absorbé par le chorion, en sorte que l’ensemble cœur-corps-chorion présente un phénomène de poupées russes auto-organisées. Au voisinage du corps, la forme hyperbolique de l’écoulement crée le pattern bien connu de vaisseaux dans l’organe extra-embryonnaire de l’amniote (sac vitellin ou placenta).
L’impression finale est que, en changeant simplement la valeur quantitative du mouvement, l’évolution darwinienne fait aller plus ou moins loin l’écoulement, sans nécessairement changer la forme globale, la topologie de cet écoulement. Le changement quantitatif des paramètres (bien entendu génétiques) du problème physique, suffit à induire une succession de plans d’organisation, dans l’ordre d’apparition successif des plis, en changeant le rapport entre la contrainte tangentielle de poussée et le module élastique du matériau, qui est le paramètre usuel de ces mécanismes de pli. Ainsi, le cœur, la tête et le chorion, ne seraient pas induits localement par des territoires présomptifs obtenus au hasard des mutations, comme communément admis, mais des structures dynamiques, que le mouvement va chercher, en avançant plus loin dans le même sens. Evidemment, ces structures, comme un chorion, doivent présenter un avantage évolutif pour être retenues par la sélection, mais il est clair qu’un chorion joue un rôle protecteur favorable. On notera que les deux parties symétriques du chorion se réunissent pour former un sac, tandis que les deux parties symétriques du pli ventral se rassemblent autour du nombril en formant l’abdomen. La contraction du pli du côté antérieur crée le cœur, ce qui laisse à penser que la contraction postérieure du pli pourrait créer un cœur également, or il existe en effet des animaux ayant un cœur caudal, et certains gros vaisseaux abdominaux des vertébrés sont connus pour être battants.
1 Introduction
During evolution of vertebrates a sequence of events is empirically observed: first, animals are bilateral, but they have no heart, no head, and no surrounding bag during development (these primitive animals are called cephalochordates [1]). “Next”, animals have a head and a heart (craniates, like fish or frog [1,2]), and “more evolved” animals (reptiles, birds and mammals) have a head, a heart and a surrounding bag called the chorion [1,2]. In terms of darwinian evolution, it is clear that these structures might confer a positive advantage for animal development [3]. However it is not clear how these structures appear, at a mechanistic level. In particular, the order of appearance seems a pure coincidence, or even a retrospective illusion, with no internal logic. The purpose of this article is to try to explain the sequence of appearance of these structures by a physical process. I shall show below that there exists an order:
In order to prove experimentally the existence of this order, I have studied by in vivo time-lapse microscopy the formation of the body axis, of the head and heart, and of the chorion in an amniote, the chicken. The entire tissue field was analyzed, both in vector direction, and in magnitude. The data reveal that the magnitude of the morphogenetic process is constant, but that non-linearities are induced by the folding. These folds occur, indeed, in a spatially organized order, which is directly related to the evolutionary order of these animals. This is to say that the compulsory physical order of folds, compells the order of appearance of the plans of these animals. This suggests that, by modifying genetically the magnitude of the physical parameters of the developmental process, but not the topology of the movements, evolution was able to induce dynamically the successive appearance of these animal organization plans (or bauplans). Of course, any genetic modification of the magnitude of traction forces is inheritable, thereby the physical, mechanistic description of animal evolution is not contradictory with the genetic view of the same phenomenon, the latter giving the quantitative parameters of the former, which grasps the general law.
2 Methods
Chicken embryos are incubated in a standard Minitüb incubator. Heart development is difficult to image due to the geometry of the embryo, and the fact that the heart lies underneath the embryo, during development. Therefore, for the heart observations, I have cultured the embryos “upside down”. In this case, the embryos are extracted out of the egg with extreme care and laid flat on a glass Petri dish with approx. 5 mm deep of PBS buffer. The Petri dish is gently stirred by hand, and all the yolk is progressively removed. The vitelline membrane is removed as well. The embryo is then gently turned upside down with twizzers, then put to incubate on a flat plate at 37 °C with a second glass plate put on top, at 38.5 °C, the temperature difference between the two plates being crucial for avoiding condensation. In such conditions the embryos may survive and develop for 3–10 hours normally. For the observation of the chorion, the embryo is cultured inside the egg, by cutting off a small window across the shell. Stacks of images of embryos at different stages of development are obtained during a time period of up to 10 hours, with a time resolution of 1 minute using grazing illumination with a fiber lamp from Schott, a Leica MZFlIII microscope, and a B&W Watek 512 analog camera interfaced with the Scion Image software, adapted from Wayne Rasband's NIH Image software. Three movies are provided on the author's website (http://www.msc.univ-paris-diderot.fr/∼vfleury/). Movie 1 shows a time-lapse movie of heart formation at mag. 2.5 × . Movie 2 provides a film of chorion closure at mag. 0.71 × . Movie 3 provides the movement of the blastula prior to embryo folding, at mag. 4.2 × . These experiments are performed under French state law R214-80 alinea 1°, regulating animal experimentation; they do not require approval by an ethical committee.
3 Heart formation
The origin of the heart is often described in terms of presence of a predetermined “cardiac territory” or “cardiogenic plate” [4,5], a concept which has little explanatory power from a physical, mechanistic, point of view. Fig. 1 shows an embryo at 1 day and a half of incubation, evidencing the formation of the dorsal folds, and of the ventral folds (same embryo). The head rudiment (Fig. 1 left) appears at the apex of the dorsal folds as a hammer-shaped fold. The presumptive territory of the heart (Fig. 1 right) is the ventral fold formed below the head, as it moves forward and shears the gastrula plane (gastrula is the name of the embryo at this stage). The heart fold has a large conspicuous edge disjoined from the underlying surface, and forming a cavity.
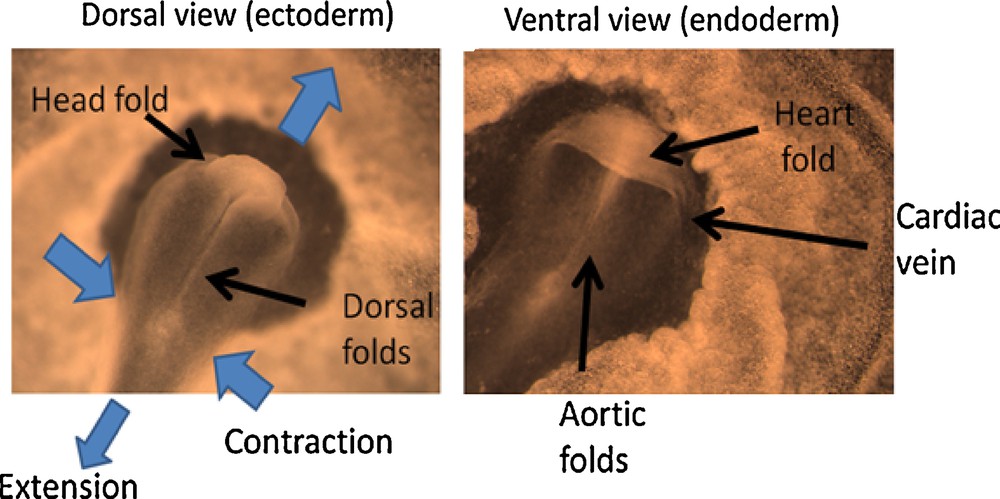
Dorsal view and ventral view of an embryo at the stage when the head rudiment and heart rudiment form (Mag. 4 ×, full scale of the image 3 mm). The images are labeled to explain the topological details. The heart fold is formed when the head fold passes over the blastula plane. The embryo is contracting in the Left-Right direction, and expanding in the antero-posterior direction. This movement generates the folds along the A-P axis, and the forward extension of the folds drags the ventral tissue which itself folds perpendicularly to the A-P folds, as the upper layers pass over it. The cardiac folds are connected to the non-folded surface by a folded edge which forms the cardiac veins.
I have filmed by time-lapse microscopy the fate of this edge, and it is very obvious that it constricts like “a purse”, such that the edge folds upon itself, generating the more compact heart tubular folding (Movie 1 and Fig. 2).

(a) Time-lapse microscopy of the heart formation (Movie 1) shows that the crescent formed in the ventral area by the forward growth of the head starts to move posteriorily (Mag. 2.5 ×, full scale of the embryo 5 mm). As it moves, the large crescent constricts upon itself and shuts progressively, thus forming the heart in the medial part of the body, and the ventral veins. The edge of the fold is split in four strands, two which will form the vitelline veins, and two which will form the ventral veins, these veins descend along the body axis. The snapshots show the stages of heart formation, from a mere crescent, to an almost recognizable heart (please see the Movie 1 for visual impression of the constriction of the edge). The bottom image shows the overlap of the reference frame t = 0, and the frame t = 200′. The somites (vertebrae precursors) have a small movement over these 3 h 20 min, while the edge has moved massively to the left. (b) The kinetics of heart formation is almost perfectly linear. (c) The transition from an edge to a recognizable heart occurs by constriction of the edge. This is not intuitive on the final heart. The left and right vascular connections to the yolk sac are just both ends of the initial fold.
The kinetics of the heart formation is strictly linear in time (Fig. 2 (b)). As the edge constricts, the lateral tubes formed by the folded arch gather along the flanks of the embryo, where folds of the embryo body form concomitantly, due to the antero-posterior extension, until they fuse and form the ventral scar around the navel. Whether any chemotactic force is at play in the movement of the arch-fold is unlikely, since the edge of the fold of the presumptive cardiac territory displays a massive motion oriented towards the posterior direction, this is to say, in Fig. 2 (a), the arrow points to the growth direction of the heart, which is the hollow cavity where there is nothing facing it (stated otherwise, the edge moves in the direction of the “vacuum” facing the edge). It is therefore impossible that the heart territory moves up any chemotactic gradient.
By carefully inclining the direction of view one can explore the inside of the cavity below the cardiac fold, and find nothing but a hollow cavity (data not shown). The cardiac fold (and the so called gut pocket) are just an empty fold. Also, while the developmental process continues, a similar fold “arrives” from the caudal end, and after final convergence of the folds coming from both ends, the ventral side shuts around the navel. However, the caudal fold obvioulsy does not form a heart (more below).
4 Chorion formation
Let us now observe the formation of the chorion. The chorion is a bag, which surrounds the embryo. When followed carefully in vivo, it is observed that the chorion emanates from two dorsal folds, one located “ahead” of the head, and another one located “more caudally” than the tail. These folds constrict again like a purse (Fig. 3 (a) and Movie 2). The kinetics of the constriction is again approximately constant in time (Fig. 3(b)), within measurement errors (at this stage, the embryo shakes slowly the chorion by its movements). The movement of closure of a hole around a singularity, by constriction of the edge, is very much reminiscent of the process of wound healing [6].
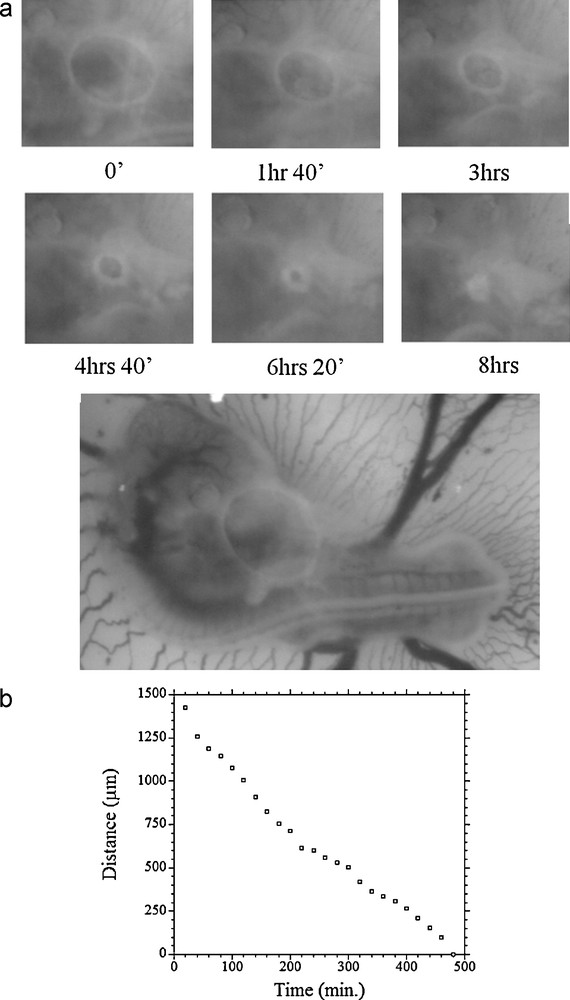
(a) Time-lapse imaging of the closure of the chorionic bag around the embryo (Mag. 0.71, full scale of the embryo 11 mm). (b) the dynamic tracking of the closure (diameter of the hole vs time) shows an almost constant speed of closure. Please note that at this stage the embryo is very large and moves inside the chorion, which might explain small departures from a linear behaviour.
More surprisingly, the magnitude of the speed of closure is very much similar to that of the heart closure. In Fig. 2 (b), the speed of closure of the heart fold is found to be 2.2 μm.sec−1, while the speed of closure of the chorion hole (rate of variation of the radius) is found to be 1.66 μm·sec−1 (note that it was not the same embryo). Remarkably enough, while the heart fold shuts eventually around the umbilical vessels, the chorion shuts around “nothing”, although there remains a visible scar on the surface of the chorion, which is a topological analog of the navel (closure of a circle around its center). All this suggests a possible common origin to both features. This is what we now address.
5 Origin of the heart and chorionic folds
As we have seen, the dynamics and the topology of heart and chorion formation are similar. If we wind backwards in time the development of these features, we find that, actually, the heart and the chorion appear as two modest folds which exist just ahead of the dorsal axis, as this axis starts to appear (Fig. 4 (a)), in the first day of development. Similar folds form at the other end of the embryo, facing the caudal end (Fig. 5), by two and a half days of development. As the dorsal axis pushes forward along the embryonic tissue, these modest folds become the round edges, which eventually constrict and close. The Fig. 4 (a) shows the heart “fold” just prior to contracting (star) and the chorion fold, just prior to contracting (arrow), in a one-day-and-a-half embryo. The corresponding schematic drawing (Fig. 4 (b)) explains the situation.

(a): Two examples of early gastrulas in the chicken embryo, showing both the heart crescent (stars), and the chorion crescent (arrows). Both are parallel (Mag. 4 ×, full scale of the image 3 mm). The heart crescent corresponds to a fold bending down with respect to the plane of the gastrula (early embryonic tissue), and the chorion crescent to a fold protruding upwards over the plane of the gastrula. Fig. 4(b): Scheme of the situation in the plane of the gastrula: the embryo dorsal axis extends, and folds form ahead of the apex of the fold, perpendicularly to it. The first fold is the heart “territory”, the second fold the chorion “territory”. For the sake of clarity, similar folds were drawn at the cranial and at the caudal end, but actually, the caudal folds appear visible one day later than the cranial folds.
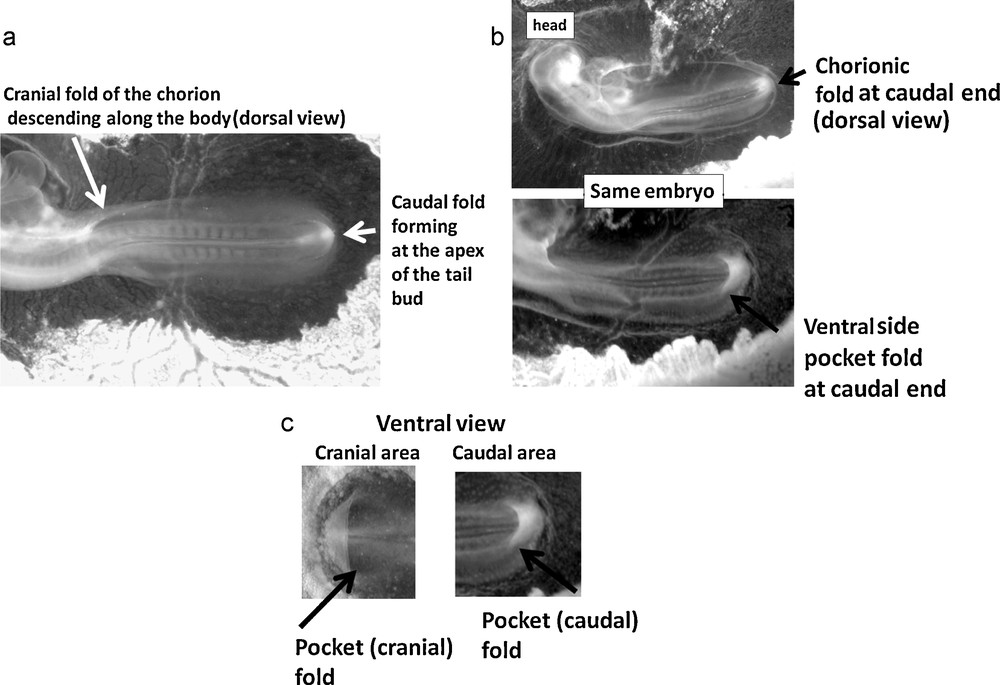
The heart and the chorion shut down like “an iris”. This iris is formed by the merger of symmetrical folds located at both ends of the embryo (cranial and caudal). (a) (full scale 7 mm) shows an entire chicken embryo, as it starts to be shrouded inside the chorion. The large oval of the chorionic fold is well visible. The fold in the head area is already formed while the caudal folds of the chorion are just forming. Indeed, just ahead of the tail bud folds form, as the tail bud “pushes” along the gastrula plane in the posterior direction (arrow in (a)). Progressively, the caudal and cranial folds forming the chorion join up and close down leaving eventually a visible scar on the chorion. (b) shows the dorsal and ventral views of the same embryo. In (b) (top), the caudal part of the chorionic fold is already passing above the tail bud (full scale 7.5 mm). The chorionic edge forms a large oval around the embryo, which is already constricting. By turning the embryo upside down, it is possible to image the ventral side of the same embryo, (b) (bottom) (full scale 6.5 mm). One observes that the caudal end has passed over the ventral fold, which forms a folded pocket very similar to the cardiac pocket (seen in 5(c) Right). The ventral fold in question is pointed by the arrow, and the pocket formed by this fold appears spontaneously whiter, probably because folded tissue diffuses more light. A clear symmetry between the cranial and caudal topology is evidenced. At both ends, the ventral fold forms a pocket, oriented with mirror symmetry. Eventually, the ventral pocket-folds shut down around the umbilical chord. (c) shows ventral views of the pocket folds in the cranial and caudal areas. The comparison of an early cranial (heart) pocket fold in the head area, and of the caudal pocket fold in the tail area shows a very clear similarity, although with a mirror symmetry. This suggests that there should exist animals with a caudal heart.
That the body stretches along the antero-posterior direction during early stages of development is a well-known fact [7]. However, in the context of this article, I have filmed with precision the developmental process just prior to the formation of the ventral and dorsal folds, in order to confirm that the heart and head folds occur perpendicularly to the developmental movement of the embryo at the blastula stage. Fig. 6 shows the vector field of development analyzed by Particle Imaging Velocimetry on a chicken blastula, at the stage when it is flat, and it exhibits only a modest furrow along the presumptive dorsal axis. At this moment, prior to formation of all other folds, the tissue movement is indeed organized with a stagnation point (marked by a red circle), and the tissue flows in the direction of the (future) head, and of the (future) tail.

Particle imaging velocimetry of the tissue flow in an early gastrulating chicken embryo, showing the vector field of movement along the antero-posterior axis. To the left the global pattern of movement over one large area of the embryo. In green, the renomalized vector field of movement, the movement of each piece of tissue located at each blue cross is followed during 50′ to generate the vector of movement. The large black arrows help to visualize the global orientations in the flow map. To the right, a magnification of the area of the apex of the folds. The flow is organized towards the head, and towards the tail, in a symmetrical fashion around a neutral point (circle in red in the image to the right). The neural crest folds have not formed yet. The first folds will form along the antero-posterior direction (dorsal folds), the next folds perpendicularly to the antero-posterior direction (heart head and chorion folds).
These observations show that, from a physical, mechanistic, point of view, there is a tissue flow organized towards the head, and towards the tail, and centered around a stagnation point.
In either direction, the heart and the chorion form, in a quite similar fashion, in a quite similar area, one being a ventral fold and one being a dorsal fold. The folds are strictly parallel, at the onset of instability, and they are found at either end, with a noticeable symmetry. At either ends, the folds are strictly perpendicular to the dorsal axis. The chorionic fold will progressively protrude above the plane of the gastrula, and pass over the embryo head, and tail, as these body features move respectively forward, and backward. Next, this fold descends along the body from the head, and goes up along the body from the tail. Such that, progressively, an “iris shutter” is formed, which closes down fully, and therefore surrounds the embryo. The closure of the fold consists topologically of a reduction, at a constant rate, of the diameter, until there remains a singular point. Therefore the topology, and even the dynamics, of this process are almost identical dorsally, and ventrally, with one important difference: the heart and ventral fold emanate from a fold oriented downwards, with respect to the gastrula plane, while the chorion emanates from a fold which is oriented upwards above the plane of the gastrula plane.
To summarize, the mechanism of heart and chorion formation is shown in Fig. 7. It reveals that actually, the embryo, the heart, and the chorion, form a system of self organized Russian-dolls: the body folds, by stretching along the axis, reach out towards the heart fold, and next the chorion fold. Since these have curvature of opposite signs, with the same dynamics, the heart ends up inside the embryo, while the embryo ends up inside the chorion. The mechanism is extremely simple, although it is not intuitive, and cannot be guessed on the final structure, which seems extraordinary, and seems to lie outside any physical rationale.
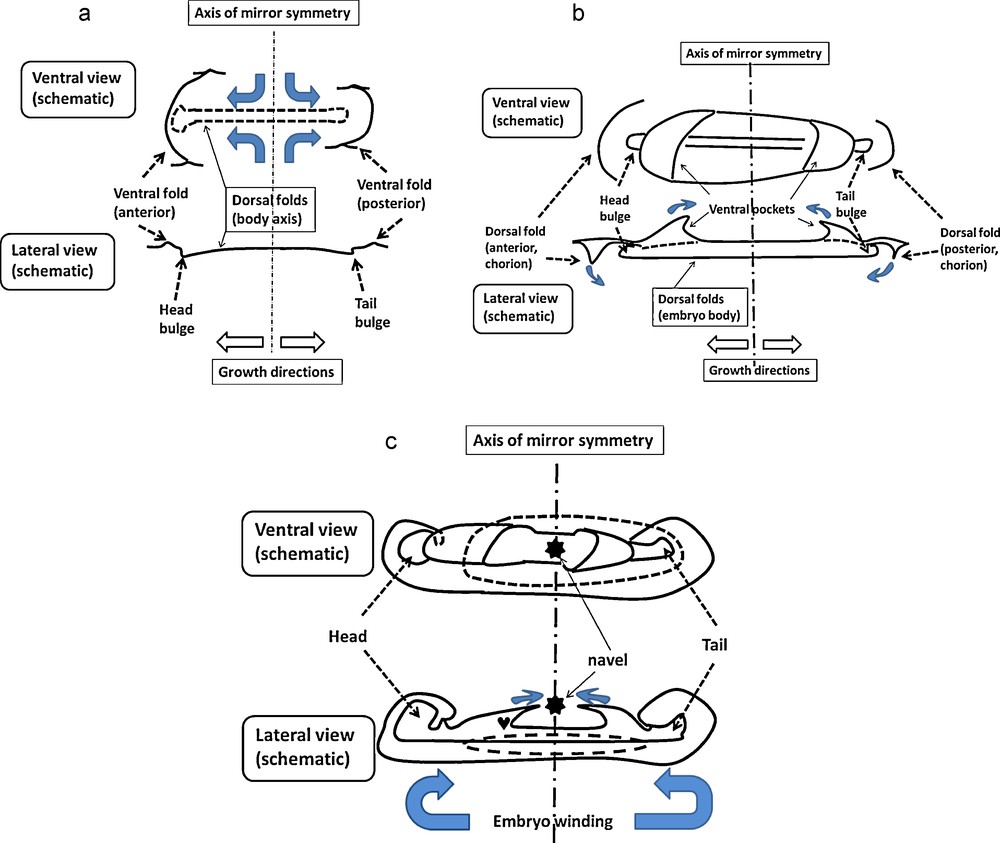
Schemes showing how the axial extension of the body correlates with the establishment of the entire topology, and in particular the formation of the heart and of the chorion. Blue arrows indicate schematically the tissue movement. (A) A ventral scheme and a cross section of the embryo at a stage where it is a flat surface except at the folds regions. The ventral folds are just being formed. The bidirectional compressions by the median axis push on the tissue at the head and caudal poles, and lift the folds below the embryo. Seen from the ventral side, the folds appear as modest sinusoidal waves over the ventral surface. (B) As the median axis puhses more, the head and tail bulges pass frankly over the blastula plane. In the ventral area the tissue is dragged forward and is folded non-linearily into a sigmoid surface ahead of the head rudiment, and somewhat later caudally to the tail. The said folds form two “pockets” on the ventral side, at both the anterior and posterior part of the embryo. The edge of the pocket in the anterior side forms the precursor of the heart. Meanwhile, a new fold appears ahead of the pocket, which will eventually be the chorion tissue. During all this process, the tissue extends from the navel area forward, and backwards, and constricts in the left-right direction. (C) Further growth winds the entire embryo. The head winds towards the chest, while the ventral pocket closes up. Meanwhile the chorion shuts dorsally. The movement of the edges will end in closure of the ventral side around the navel, and complete wrapping by a chorion. Globally, the embryo winds cranially and caudally, it shrouds the heart, and it is shrouded by the chorion.
6 Discussion and conclusion
It is remarkable that the heart lies ahead of the dorsal, median axis, as regards the direction of the vector field of formation of the body axis, and that the chorion lies ahead of the heart, i.e. ahead of both the dorsal folds and the heart fold, as regards the direction of development of the body axis. Therefore, this suggests that there are no “cardiac territory” nor “chorion territory”, pre-determined to create these features, but that the dynamics of the developmental process catches up these features dynamically. This is also confirmed by the fact that animals such as cephalochordates, which have no heart and no head, exhibit a movement of development which is arrested sooner during development, as the embryo axis forms [1]. By arresting the developmental movement sooner, the head and heart would not be able to form. Let alone the chorion, which lies even more ahead. Moreover, the data shows that tissue constriction occurs at a constant pace, and even with an almost constant magnitude in organs as different as a heart and a chorion. The body movements also have the same quantitative speed. However, a chicken embryo, such as those studied here, have evolved “randomly” for 600 My since the Precambrian. Still, they have reached a situation in which a heart, and a chorion, form at a constant speed, with an almost equal dynamics.
This suggests that the physical constraints of the vector field fixes the topology of the problem, and that only the magnitude of the process is determined randomly, by fixing the magnitude of the cell-cell contraction force at a molecular level. During the heart formation, and ventral closure, and during chorion formation and closure, the driving force is constant, and exhibits no non-linearity despite the time given to evolve any complex non-linearity. However, such a constant being given, a heart, and next a chorion will form as a physical process of fold and constant constriction. This is eventually identified as a bifurcation in animal organization plan. This is corroborated by the fact that the two parts (caudal and cranial) of the chorionic folds form actually very far from each other, at both ends of the body axis, and in a symmetrical fashion, prior to reconnect to each other in the form of a circular iris-shutter. Considering the distance across the embryo, this suggests that this kind of fold is general enough to occur at least twice, at both ends of the embryo. From a purely genetic point of view, it would seem a remarkable coincidence that the developmental “program” should have evolved by some evolutionary convergence an identical physical feature at both ends, which would spontaneously reconnect, and eventually close so perfectly. This suggests that the concept of a “chorionic territory” is empty, and that it is the dynamics of the folds which generates such folds, by a physical mechanism. Mechanical interactions in a continuum material are intrisincally long-ranged. This description gives sense to the order cephalochordates anamniotes amniotes, which would occur downstream of a physical movement of embryogenesis (body axis extension in a finite embryonic plate), as the magnitude of the parameter of contraction would progressively increase as a consequence of genetic mutations. Of course, the value of these parameters is genetically passed to offsprings.
The model would predict that there should exist animals with a heart located symmetrically to the heart in the chest, therefore near the tail. By searching in the litterature, I was surprised to see that it is indeed the case, there exist primitive fish, hagfish, with an aneural heart[8].
A puzzling issue is why the early heart fold, at the stage when it is just a wave of modest amplitude, bends ventrally, while the chorion fold bends dorsally. It is classical in physical instabilities that initial modes have a sinus wave form, therefore, it is natural for the first two curvatures to have opposite signs. If such were the case, the formation of amniotes would be a deterministic attractor of a physical process over a flat visco-elastic plane.