1 Introduction
Rainforests in Gabon, like many tropical rainforests, have a high species richness [1]. Although the determinants of species richness in these forests have been much debated, the question remains unsolved for most organisms. Squirrels form an interesting group to address this question because of their conservative morphology [2] and their ecology. Nine species of sciuridæ coexist in the rainforests of Gabon. Each species belongs to a specific niche of the ecosystem and lives in a given level of the arboreal environment, ranging from the ground to the canopy. Emmons [3] studied the ecology of the nine squirrel species of Gabon. Her study showed that all species coexisted thanks to a gradient of adaption to tree-dwelling that varied both vertically and horizontally. The explanation of this adaptive gradient is twofold. From an ecological point of view, competition for food access brings the animals to occupy preferentially or exclusively some sites. From the point of view of morphological adaptation, species have acquired specific structural traits that facilitate and optimize their displacements to access food.
The functional anatomy of the hand and feet in particular is closely linked to posture and locomotion since these organs are directly in contact with the physical medium, and because they form the biomechanical link through which forces are transferred to the postcranium [4–13]. Many studies have been devoted to the locomotion adaptation of rodents [14–16], but more precise studies about squirrels are lacking.
In order to determine the adaptive significance of some morphological and behavioural trait, it is necessary not only to assess its function in a given ecological situation, but also to determine how the trait varies with different environmental conditions and within different species. If a state of character is invariant among all members of a group, then it is likely to be a general adaptation of the group as a whole. If the state of character varies, then the pattern of variation should provide evidence about the selection pressures resulting from specific environmental conditions.
This study aims at identifying morphological traits of the foot of squirrels of Gabon in relation to the species and the environment. The feet of seven sympatric squirrel species were digitalized in three dimensions and the resulting 3-D shapes were compared. Differences in shapes are difficult to assess using simple dimensions such as lengths or widths. We thus used three-dimensional Procrustes analysis [17]. This method permits us to quantify the shape of a complex organ (such as the foot sole) and to assess shape variations within a group of individuals independently of size. Procrustes analysis was used as a substitute to dissection because the muscles of the feet are to small to be measured separately. An underlying assumption of this study is thus that the volume of the foot is related to the volume of muscles.
2 Material and methods
2.1 Species, habitat and locomotion prevalence
This study focused on seven species found in Gabon rain forests: Paraxerus poensis (Smith), Epixerus ebii (du Chaillu), Funisciurus isabella (Gray), Funisciurus lemniscatus (Leconte), Funisciurus pyrrhopus (Cuvier), Heliosciurus rufobrachium (Thomas), and Protoxerus strangeri (Waterhouse).
A survey of the literature concerning the habitat and locomotion prevalence of these species yielded the synthesis illustrated by Fig. 1 [3,18–28]. As regards habitat, four species live below 5 m from the ground whereas the three other species live from 5 to 35 m above ground (Fig. 1a). Prevalent escape mode is running on the ground for E. ebii (that is by far the fastest squirrel in Gabon), F. pyrrhopus and F. lemniscatus, and climbing up trees for the four other species (Fig. 1b). The two species that spend most time on the ground are F. pyrrhopus and E. ebii (Fig. 1c). Then come the other Funisciurus species. P. strangeri never comes on the ground, whereas H. rufobrachium and P. poensis seldom come on the ground. Those species with a low habitat (⩽5 m from the ground) also have a lower range of vertical movements (⩽15 m), whereas the species with a higher habitat have a larger range of vertical movements (up to 30 m; Fig. 1d).
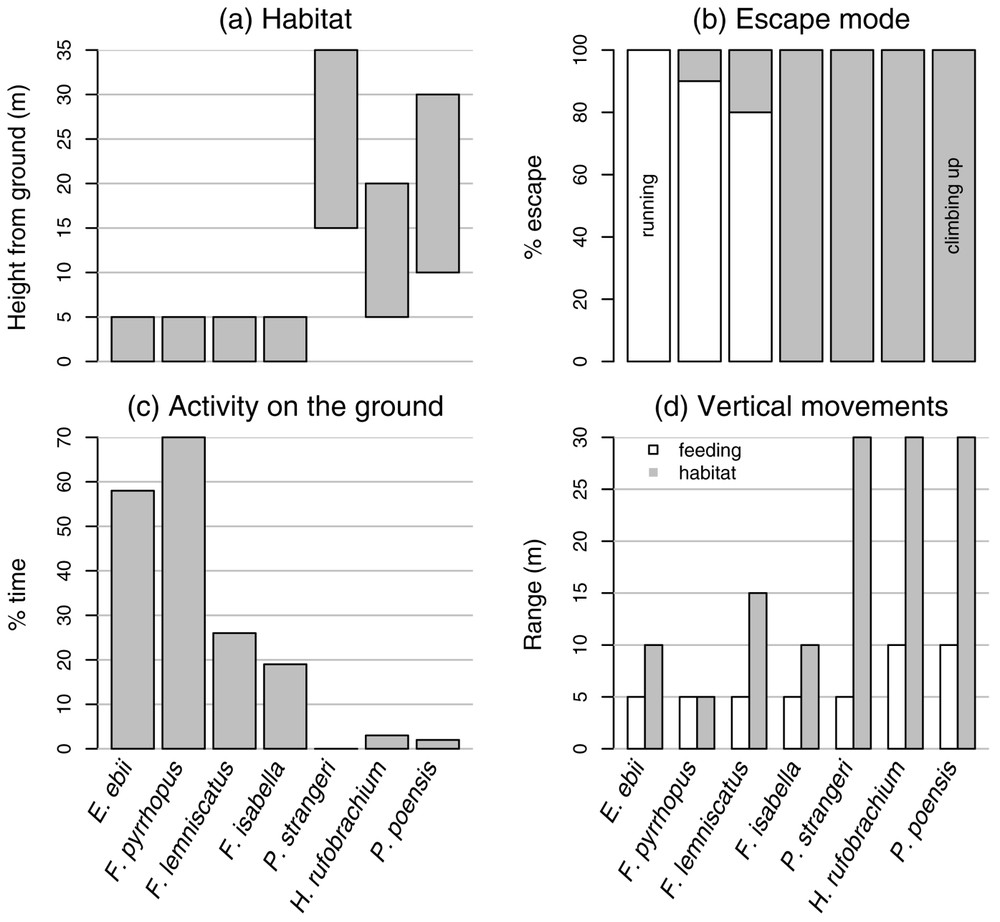
Habitat and locomotion prevalence of seven squirrel species.
Based on this synthesis, we defined four groups of species that correspond to four degrees of tree-dwelling:
- 1. Species that spend more than 50% of their time on the ground. Vertical movements for feeding are about 5 m in range, habitat is less than 10 m from the ground, and running on the ground is the escape mode in more than 80% of cases. It includes E. ebii and F. pyrrhopus.
- 2. Species that spend between 15 and 25% of their time on the ground. Vertical movements for feeding are about 5 m in range, habitat is less than 10 m from the ground, and climbing up is the escape mode in more than 20% of cases. It includes F. lemniscatus and F. isabella.
- 3. Species that spend less than 5% of their time on the ground and whose vertical movements for feeding are about 5 m in range. Habitat is about 25 m from the ground, and climbing up is the exclusive escape mode. It includes P. strangeri.
- 4. Species that spend less than 5% of their time on the ground and whose vertical movements for feeding are about 10 m in range. Habitat is between 5 and 30 m from the ground, and climbing up is the exclusive escape mode. It includes H. rufobrachium and P. poensis.
2.2 Study sites and material
Individuals were collected around Makokou, East of Gabon (N, E, altitude 500 m). Vegetation is evergreen forest with a canopy between 20 m and 35 m in height on average, with higher emergent trees. Flora species is dominated by raphia palms and lianas, especially near rivers. There are two dry seasons with temperatures between 21 and , and two rainy seasons with temperatures between 25 and . Relative humidity is 85% on average [29–31].
Ninety individuals from the seven species were collected and fixed in 10% formaldehyde solution. Table 1 gives the average body sizes and the number of individuals collected for each of the seven squirrel species.
Average body sizes (in mm) and number of individuals collected for each of the seven squirrel species.
Species | n | Head + body | Tail | Foot |
Paraxerus poensis | 11 | 155 | 160 | 35 |
Epixerus ebii | 16 | 285 | 300 | 66 |
Funisciurus isabella | 11 | 167 | 162 | 39 |
Funisciurus lemniscatus | 2 | 195 | 174 | 46 |
Funisciurus pyrrhopus | 13 | 215 | 175 | 47 |
Heliosciurus rufobrachium | 19 | 230 | 246 | 52 |
Protoxerus strangeri | 18 | 265 | 310 | 63 |
2.3 Landmarks
Twenty five landmarks were defined on the sole of the hind foot: eleven (n°1–11) on the outline of the sole, and fourteen (n°12–25) on the foot pads. The 25 landmarks are shown in Fig. 2 and can be described as follows: n°1 = outermost point of the heel, n°2 = internal extremity of the largest part of the heel, n°3 = internal extremity of the narrowest part of the heel, n°4 = internal extremity of pad I (see Fig. 2), n°5 = most advanced point of pad I, n°6 = most advanced point of pad II, n°7 = most advanced point of pad III, n°8 = most advanced point of pad IV, n°9 = external extremity of pad IV, n°10 = external extremity of the narrowest part of the heel, n°11 = external extremity of the largest part of the heel, n°12 = most posterior point of the thenar eminence, n°13 = most advanced point of the thenar eminence, n°14 = most posterior point of pad I, n°15 = most posterior point of pad II, n°16 = most posterior point of pad III, n°17 = most posterior point of pad IV, n°18 = most advanced point of the hypothenar eminence, n°19 = most posterior point of the hypothenar eminence, n°20 = most prominent point of the hypothenar eminence, n°21 = most prominent point of pad IV, n°22 = most prominent point of pad III, n°23 = most prominent point of pad II, n°24 = most prominent point of pad I, and n°25 = most prominent point of the thenar eminence.
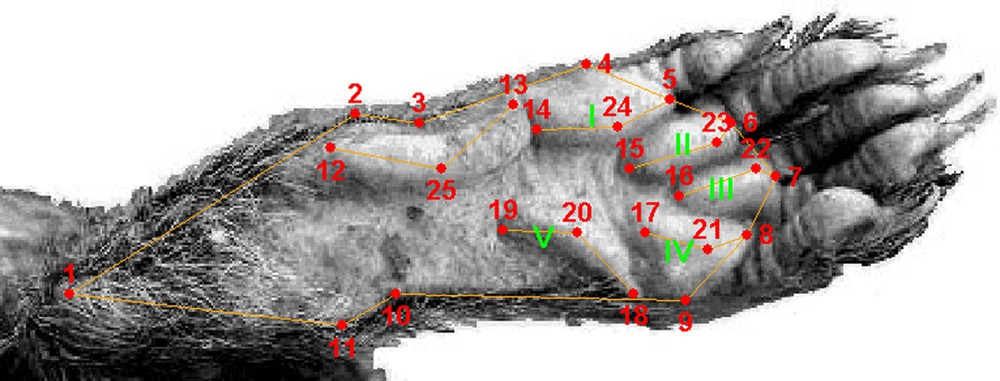
Location of the 25 landmarks on the foot sole (here a left hind foot of P. strangeri). Roman numbers indicate the pads.
The Cartesian coordinates in three dimensions of the 25 landmarks were digitized for each foot sole with 0.1 mm precision using Microscribe-3D®.
2.4 Procrustes superimposition
The set of 25 landmarks for each specimen forms a simplified shape. The n shapes were superimposed using a generalized Procrustes analysis (GPA; see [17,32–34]). This analysis filters out all location, scale and rotational effects to keep the invariant geometrical information contained in the shapes. The outcome of a shape by the transformation that results from GPA is named a conformation, and the average conformation that results from GPA is named the consensus C.
The global orientation of conformations resulting from the GPA is arbitrary. To plot a conformation, we used the viewpoint such that landmarks n°1, 7 and 2 of the consensus be in the horizontal plane with landmarks n°1 and 7 aligned with the x-axis. The plot of a conformation consists of orthogonal projections on the planes and of this viewpoint.
Procrustes superimposition was achieved using APS® software version 2.41 by X. Penin (2001, http://www.cpod.com/monoweb/aps).
2.5 Statistical analysis
The residuals of the n shapes with respect to the consensus C were analyzed using a principal component analysis (PCA) without scaling. The PCA defines a rotation matrix R that gives the coordinates of the principal components in the space of the initial variables. Given a point P in the space of principal components, the corresponding conformation can be reconstructed as . This conformation does not necessarily corresponds to an observation but is representative of location P in the space of principal components.
The coordinates of individuals along principal components can be related to explanatory variables. We used as an explanatory variable the degree of tree-dwelling, categorized according to four levels. For each principal component, an analysis of variance was performed to test whether the coordinates of individuals on this principal component significantly vary with the degree of tree-dwelling.
Statistical analyses were achieved using R software [35] and ADE4 package [36] for PCA.
3 Results
The first two axes of the PCA explain 18.9 and 14.3% of the total variance. As the contribution of the subsequent axes sharply decreases, only the first two axes of the PCA were kept for analysis. Fig. 3 shows the correlation circle of the PCA. Two residuals out of 75 are mainly correlated with the first two axes: the residual of the abscissa of landmark n°25 and the residual of the abscissa of landmark n°1. Both are negatively correlated with the first axis of the PCA. The former is positively correlated with the second axis whereas the latter is negatively correlated with it. Hence the landmarks that contribute the most to discriminate between conformations are the most prominent point of the heel pad (n°25) and the outermost point of the heel (n°1).

Correlation circle of the principal component analysis of the 75 Procrustes residuals for each individual. x1 is the Procrustes residual on the x-axis for landmark n°1, y1 is the Procrustes residual on the y-axis for landmark n°1, z1 is the Procrustes residual on the z-axis for landmark n°1, x2 is the Procrustes residual on the x-axis for landmark n°2, and so on. The width of the grey grid is 0.2.
Fig. 4 shows the changes of conformations when moving along the axes of the PCA. Along the first axis, the sole of the foot is getting longer and the most prominent point of the heel pad is moving backwards as we move from negative to positive values. Along the second axis, the sole of the foot is getting longer and the most prominent point of the heel pad is moving forwards as we move from negative to positive values. The shortening of the foot sole is correlated with other morphological traits of the foot: widening of the distal part of the foot sole, concentration of the foot pads towards the distal part of the foot, fanning out of the foot pads, and thickening of the foot sole.

Changes of conformations along the axes of the PCA. The dashed outline is the consensus. The solid outlines are the conformations at the end of each axes of the PCA. Their corresponding points in the plan 1–2 of the PCA are A, B, C and D (see Fig. 5). Landmarks n°1 and 25 are indicated by their number.
Fig. 5 shows the projection of the individuals on the first two axes of the PCA, together with their species. Each point in Fig. 5 corresponds to an individual, whereas the symbols and the convex hulls indicate the species. Two species, E. ebii and P. strangeri, are well separated on the plan of the PCA, meaning that they have distinct morphological traits. E. ebii is characterized by a long foot and a heel pad whose most prominent point is in back position. P. strangeri is characterized by its heel pad whose most prominent point is in medium position, and has an medium foot length. The remaining five species organize along an axis that is perpendicular to the direction given by variable x25 in Fig. 3. As x25 corresponds to the abscissa of the most prominent point of the heel pad, it means that these five species share in common the position of the most prominent point of the heel pad that is in front position. The axis along which the five species are distributed is parallel to x1 (see Fig. 3) and thus corresponds to a gradient of foot length. At one end, P. poensis and H. rufobrachium have a short foot. These two species are actually superimposed on the plan of the PCA, meaning that they have similar morphological traits. At the other end, F. pyrrhopus and F. lemniscatus have a long foot. F. isabella lies in between.
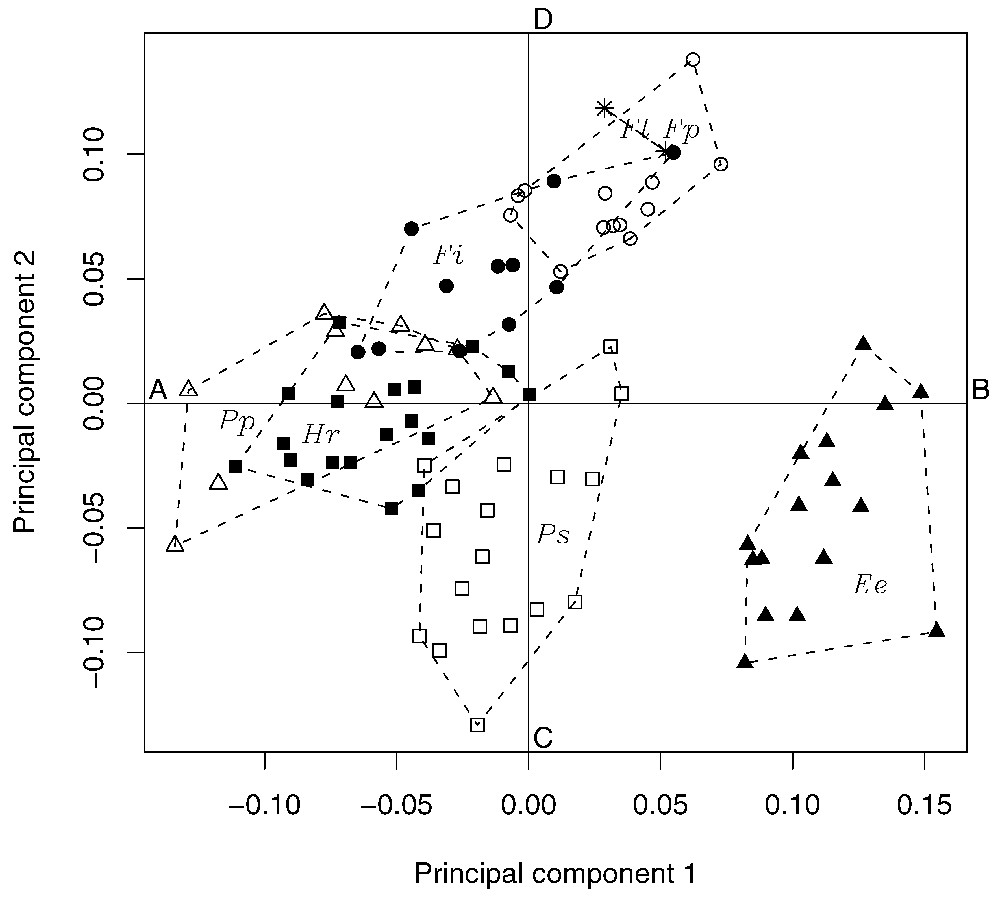
Projection of the individuals of the first two axes of the PCA, together with their species: black triangles = E. ebii, white square = P. strangeri, black squares = H. rufobrachium, white triangles = P. poensis, black dots = F. isabella, asterisks = F. lemniscatus, and white dots = F. pyrrhopus. Points A–D correspond to the conformations shown in Fig. 4.
Fig. 6 shows the projection on the first two axes of the PCA of the four groups of species corresponding to four degrees of tree-dwelling. Each point in Fig. 6 corresponds to an individual and their location are exactly the same as in Fig. 5, whereas the symbols and the convex hulls indicate the groups. The four groups are well separated on the plan of the PCA, meaning that they have distinct morphological traits. Fig. 6 thus indicates that there is a relationship between the degree of tree-dwelling and foot morphology. This is confirmed by the analysis of variance of principal component coordinates with respect to the groups of tree-dwelling. The analysis of variance is highly significant for the first axis of the PCA (, , p-value <0.001) and indicates that the four groups have significantly distinct positions on the first axis. The analysis of variance is also highly significant for the second axis of the PCA (, , p-value <0.001), but not all groups can be distinguished along the second axis: groups 1 and 4 do not have significantly distinct positions on the second axis.

Projection of the individuals of the first two axes of the PCA, together with their degree of tree-dwelling: white squares = group 1, white dots = group 2, white triangles = group 3, black dots = group 4.
These results show that there is a significant relationship between the degree of tree-dwelling and foot morphology. Analysing Fig. 6 in relation with Fig. 3 permits us to specify this relationship: groups 1 and 2 correspond to a long foot whereas groups 3 and 4 correspond to a short foot. Groups 2 and 4 have the most prominent point of the heel pad in front position, whereas groups 1 and 3 have the most prominent point of the heel pad in medium position.
4 Discussion
The PCA of the residuals of the Procrustes analysis revealed a consistent pattern between the squirrel species and the distribution of individuals along the two principal axes. Each axis supports a different interpretation. The first axis of the PCA corresponds to the adaptation gradient to arboreal locomotion. This adaptation corresponds to the shortening of the foot sole, widening of the distal part of the foot sole, concentration of the foot pads towards the distal part of the foot, fanning out of the foot pads, and thickening of the foot sole. The spreading of fingers, pads and muscles gives a greater support area and facilitates clinging by giving wider spatial angles. Assuming that the volume of the foot reflects the volume of muscles, wider and thicker foot soles in arboreal squirrels are in relation with thicker, and thus more powerful, muscles. Clinging efficiency for arboreal squirrels is thus improved by an increased plantar area thanks to a semi-circular pattern, an increased power of planter muscles, and the fanning out of muscles.
The foot of terrestrial squirrels is longer. Intrinsic muscles of the sole, and tendons therein, are arranged in the direction from calcaneum to phalanx [37]. The lengthening of the foot thus corresponds to a lengthening of the muscles that compose it. According to Starlings's law (the contractile strength of a muscle is directly proportional to the length of its fibres at the beginning of the contraction), the contractile strength of the foot muscles of terrestrial squirrels is greater than that of arboreal squirrels. This confers to terrestrial squirrels a more efficient leap and thus a faster running. On the other hand, the foot sole of arboreal squirrels is thicker and wider than that of terrestrial squirrels, which corresponds to a greater volume. The strength of a muscle depends on the number of fibres that it contains and is thus proportional to its cross-sectional area [38]. Assuming that the volume of the foot reflects the volume of muscles, the muscles of the foot sole of arboreal squirrels are therefore more powerful than those of terrestrial squirrels.
In summary, the autopod of arboreal squirrels permits a more efficient clinging whereas the autopod of terrestrial squirrels contributes to horizontal movements. The most ancient known fossils of squirrels date back from the Oligocene and present the morphology of arboreal squirrels [39]. It may thus be hypothesized that terrestrial squirrels are arboreal squirrels that get late adapted to terrestrial locomotion. The lengthening of the hind foot going with the decrease of its width are morphological adaptations of the hind limb to ground locomotion that are close in shape to those of other terrestrial rodents, such as the jerboa in desert areas. Complementary studies showed that these morphological differences go with other adaptations such as a soleus muscle more suitable for quick actions [37].
The distribution of the species along the second axis of the PCA can be interpreted from a phylogenic point of view. Species distributions along the second axis partially overlap. Three groups of species are difficult to separate on the second axis: (a) Epixerus ebii and Protoxerus strangeri, (b) Heliosciurus rufobrachium and Paraxerus poensis, and (c) Funisciurus pyrrhopus, Funisciurus lemniscatus and Funisciurus isabella.
Group (a) (E. ebii and P. strangeri) is composed of the two species that were grouped under genus Protoxerus in the first classification of African squirrels by Thomas [40]. Since 1945, Epixerus has been set as a separate genus but it remains the closest phyletic group to Protoxerus in classifications of African squirrels. The two species have the same coordinates along the second axis of the PCA.
The two species of group (b) (H. rufubrachium and P. poensis) belonged to the same genus Heliosciurus in Thomas' classification [40]. Hollister [41] separated Paraxerus under the name Aethosciurus, as an independent group affiliated to Heliosciurus. The two species overlap on the second axis of the PCA with a small shift. Aethosciurus became Paraxerus when Moore [2] found many affinities that permitted to link up this group to Funisciurus. Correspondingly, P. poensis is slightly shifted on the second axis of the PCA towards Funisciurus sp. Heliosciurus remained in the group of Protoxerini. Correspondingly, H. rufubrachium is slightly shifted on the second axis of the PCA towards Protoxerus.
The three species of group (c) (F. pyrrhopus, F. lemniscatus and F. isabella) belong to the homogeneous genus of Funisciurus. These three species do not overlap on the second axis of the PCA as much as the species of the first two groups. They are arranged along a line with a positive slope that extends to species Paraxerus poensis.
The genera key for the studied sciuridæ relies (as for most mammals) on the shape of cranial bones and teeth [42–45], the colour and distribution of the coat and size being used to determine the species. Yet the morphological affinities that follow from the second axis of the PCA coincide with those obtained from cranial data. The main changes of foot shape along the second axis of the PCA look like that along the first axis of the PCA, where it corresponds to an adaptation to locomotion. This suggests that, even if the way of life influenced the morphology of the foot sole of Gabon squirrels, this morphology was also influenced by their phyletic origin.
In the same way as the morphology of the plantar and palmar area of fossil primates has been used to understand past living conditions [13,46,47], some hypothesis may be formulated as regards the origins of terrestrial locomotion in Gabon squirrels. It is now evidenced that successive episodes of dryness and humidification of the quaternary climate in Central Africa yielded successive disappearances and colonisations of the forest [48,49]. It may thus be hypothesized that the isolation of forest fragments inside savannas led to a terrestrial behaviour among squirrels that were once arboreal.